Abstract
Treatment reproducibility is important to guarantee reproducible treatment-outcome, a low-complication rate and efficient treatment procedures. This study evaluated the performance of loco-regional deep hyperthermia with four BSD-2000 configurations during 1990–2005 using the direct available parameters, i.e., temperature and power. Primary cervical cancer patients (n = 444) were all treated within the Sigma-60. Patients were grouped in three weight-groups: <61 kg, 61–70 kg, >70 kg. Different temperature and power indices were extensively analyzed per BSD configuration, per weight-group, and over the time-period. No substantial variations were found for temperature/power indices over the four BSD configurations or for the temperature doses in similar weight-groups. The ‘bare’ power indices were increased with weight; however, the derivative power-related (W/kg, W/cm2) and temperature indices decreased. Large variations were found in the power-related parameters during 1991–1996 (1st time-period), whereas they were much smaller during 1997–2005 (2nd time-period). The most relevant change noted was the adaptation of the treatment strategy with respect to power modulation. The average frequency of switched-off was 3.4 and 8.9 times/treatment session for the 1st and 2nd time-period, respectively, while the average duration of each switched-off time was 78.2 vs. 38.3 s. The yearly average of vagina T50 was in the range of 39.3–40.2°C (1st time-period) and 40.0–40.5°C (2nd time-period). In 40% of the patients, a positive correlation was found between normalized net integrated power per pelvic area and vagina T50. Good reproducible heating is achieved with the BSD-2000 Sigma-60 irrespective of the regular technological upgrades of the system and variation of trained staff-members.
Introduction
Hyperthermia (HT) is considered a valuable anticancer treatment modality in several Western countries. A review by Wust et al. Citation[1] on state-of-the-art up to March 2001, HT identifies 18 comparative, prospective phase III trials on different HT modalities. Twelve of these 18 trials demonstrated a significant positive effect on treatment outcome of which seven showed benefit in survival. Since then, three more positive phase III trials Citation[2–4] demonstrating the efficacy of adding HT to conventional treatment have been published. For advanced cervical cancer adding HT to radiotherapy (RT) doubles the 3 years survival rate (27 vs. 51%) Citation[5], Citation[6] and this gain in survival persists at least 12 years Citation[7]. For recurrent breast cancer in earlier irradiated areas, the local control rate for RT + HT is doubled or tripled compared to RT alone (39 vs. 79% and 24 vs. 68%) Citation[3], Citation[8]. The doubling of local control- or survival rate following the addition of HT to conventional therapies without increased toxicity is, in historical perspective, an extraordinary and unsurpassed finding.
Generally, HT is applied by electromagnetic radiation and requires the use of complex high-technological equipment Citation[9]. Given the continuous technological evolution of the available HT devices and growing personal experience, extended data that analyses over a long period provide an excellent opportunity to study our ability to induce a reproducible temperature increase in the target region. Of course, reproducible heating is a major factor for the general acceptance of HT as a common treatment modality. Additionally, knowledge about the level of reproducibility is important for the design of treatment protocols, quality assurance procedures, and to make valid assumptions on prognostic factors. No doubt that reproducibility will be reflected in ease of application, time efficiency, safety for the patient, etc. Finally, the knowledge obtained by such studies might be fruitfully used to design education and training programs for HT staff-members.
In the last three decades, several studies were performed on HT device evaluation. For instance, Egawa et al. Citation[10] have evaluated a radio frequency (RF) and a capacitive heating apparatus in the clinical trials of combined RT and HT. Van Rhoon et al. Citation[11] also have performed an investigation of RF capacitive heating device in which they have focused mainly on thermometry. These two independent studies covered only a low number of patients (n = 45 and 11, respectively). Sapozink et al. Citation[12] have analyzed the efficacy of the BSD-2000 with the annular array applicator in a 2-year time-period for 46 patients with abdominal and pelvic tumors. Furthermore, in two separate studies, Sapozink et al. Citation[13], Citation[14] have also evaluated different heating devices for a longer time-period (7 years) and larger patient population (n = 137 and 573, respectively). In these studies, they have investigated the effect of different HT equipment on the quality of treatment, however, they analyzed the data from different tumor sites.
Since 1990, the Hyperthermia Unit of Erasmus Medical Center has used the BSD-2000 system for deep hyperthermia (DHT) treatm Citation[15], Citation[16]. Over the period of 1990 to present (2007), the BSD Medical Corporation (Salt Lake City, Utah, USA) has upgraded three times our BSD-2000 system to follow the latest improvements. In practice, this implies that in this time-period we have used the BSD-2000 system with four different power drive systems to apply DHT to tumors in the pelvic region. For years, the HT treatment data recorded by the PDOS-based computers of the BSD-2000 system were hard to access. Subsequently, research concerning power and temperature was postponed. The development of RHyThM (Rotterdam Hyperthermia Thermal Modulator) Citation[17] offered us the unique ability to perform extensive analysis of the temperature and power behavior of the various BSD-2000 HT systems. To the best of our knowledge, the present study is the first attempt, in which one used power and temperature data in patients with primary cervical cancer to analyze the long duration (15 years) performance of regional deep heating with the BSD-2000 system.
The goal of this study was to evaluate the global behavior of the performance of DHT using the BSD-2000 with the Sigma-60 applicator driven with one of four different power drive systems over the years from 1990 to 2005. As temperature is a primary indicator of treatment quality, immediately available at the end of the treatment session for quality analysis, our intention in this study was to find a power-related parameter that correlates with average temperature in the clinical situation. Our investigations regarding a relationship between thermal dose parameters and treatment outcome, a long-term variable, will be reported in a separate paper.
Patients and materials
Patients
All patients with primary cervical carcinoma who have been treated with HT, in addition to RT or RT plus chemotherapy (ChT), at the Hyperthermia Unit of Erasmus MC-Daniel den Hoed Cancer Center from January 1990 to December 2005 were eligible for this retrospective study. Of this group, 444 patients who have been treated with the BSD-2000 DHT system and the Sigma-60 applicator and with accessible treatment's data were selected.
RT and ChT
RT was performed at different institutes in the Netherlands. The patients received RT to the whole pelvis, conformal to the standard in the Netherlands, mostly 23–28 daily fractions of 1.8–2 Gy in 5 weeks in combination with a brachytherapy boost, if feasible, to a total dose of 17 Gy high-dose rate or 20–30 Gy low-dose rate. For patients with three modality treatments, in whom ChT was applied in combination with RT and HT, ChT consisted of at least four and maximum five courses of weekly cisplatin with standard hydration and antiemetic premedication. For more details of the treatments see van der Zee et al. Citation[5], and Westermann et al. Citation[18].
Hyperthermia
HT was performed using one of the four configurations of the BSD-2000 system, i.e., the BSD-2000 with Quad amplifier; the BSD-2000-3D with Quad amplifier; the BSD-2000-3D using a Quad amplifier with 3D drawer, which divides the four channels into 12 channels; and the BSD-2000-3D with Dodek solid state amplifier. All configurations were connected to one of the two versions of the Sigma-60 applicator. The 2nd version, with the same design, was used from 1998 onward.
One to 5 (mean 4.5) loco-regional DHT treatments were delivered to the whole pelvis volume once weekly during the period of RT. HT was carried out by the institutional protocol of the department as follows. The frequencies used were in the range of 70–120 MHz. The initial RF-power was 400 W. Every 5 min the RF-power output to the applicator was increased (up to 1600 W), in steps of 100 W, until the patient's tolerance threshold was reached. Hereafter, we applied SAR-steering (SAR: specific absorption rate) by changing phase and amplitude settings with the aim to reduce power-limiting hot-spots (i.e., normal tissue temperature >43°C or pain complaints of the patient) and to achieve intraluminal temperatures of 40–43°C as homogeneously as possible. This protocol has changed over the years: in the early years we reduced power after the patient complained of a hot-spot, nowadays we react with a short power-off period followed by phase/amplitude steering.
Patients were carefully instructed to mention any unpleasant sensation that might be the result of a hot-spot, such as a burning sensation, a feeling of pressure, any pain, and bowel or bladder spasm. If the patient reported pain, which disappeared within one minute following power-decrease, it was considered to indicate a too high temperature, and then the treatment settings were adjusted to decrease power input at the specific location. Adjustments of treatment settings could be as follows: adaptation of phase settings, amplitude, and frequency, or by placing additional water boluses. For deep pain complaints phase steering was preferred while for superficial pains amplitude steering was applied.
DHT treatment consisted of a heating phase of 30 min followed by 60 min therapeutic time. The temperature of the applicator's water bolus was maintained at 20°C. Increase in systemic temperature was limited by cooling measures: undressing, air-conditioning, wet towels, ice packs, and cooling bolus placed in the neck. The bladder was kept empty with a Foley catheter.
Thermometry
For thermometry closed-tip catheters (William Cook Europe, P5.0-CE-50-SFT-NS-0, Denmark) were inserted intraluminally in the urinary bladder, rectum, and vagina lumen at the beginning of each treatment session. Thermistors with high-impedance lead, i.e., the Bowman probes Citation[19], as standard delivered by the BSD-2000 system were used to assess real time temperature reading.
After catheter placement, the intraluminal depths were documented: using a standard caliper the HT-technician measured the insertion length of the thermometry catheters manually. Temperature mapping was performed along the length of the thermometry catheter in 1 cm increments to a maximum mapping length of 14 cm. Thermal mapping started just before the HT treatment and was repeated hereafter at 5 min intervals. The accuracy of the temperature measurement was ±0.2°C with a precision of ±0.1°C.
The temperature data were measured intraluminally, in total conformity with the ESHO-guidelines for DHT Citation[20]. The intraluminal temperatures were recorded as normal tissue, tumor-contact, tumor-indicative, and overall measurements. Tumor-contact means that the catheter at the site of measurement lies in contact with tumor. When the site of measurement is in the same transverse plane as the tumor, but not in contact with the tumor, the temperature is called tumor-indicative Citation[21]. The remaining measurements represent normal tissue. The overall intraluminal temperature includes all measurements within one catheter.
Data processing
The RHyThM software was used to access and analyze the HT data. An extensive description of this program and the methods of data analysis are included in two previous publications by Fatehi et al. Citation[17], Citation[22]. The patients were grouped in three weight-groups, i.e., weight-group 1 (w < 61 kg), weight-group 2 (61 ≤ w ≤ 70 kg), and weight-group 3 (w > 70 kg), such that each subgroup holds approximately one third of the treatments. Then the relationship between different power and temperature indices was computed irrespective and respective to the BSD-2000 configurations, weight-groups, and over the time-period.
Variables
The following variables were used for the analysis:
patient's age (years),
patient's weight (kg),
pelvic area (cm2), see Equation 3,
mean forward power (W),
maximum forward power (W),
mean net power (W), which is defined as mean forward power minus mean reflected power,
mean net power per pelvic area (Wcm−2),
mean net power per weight (Wkg−1),
mean net integrated power or more specifically ‘delivered energy’ (kJ), see Equation 2,
mean net integrated power per pelvic area (kJcm−2),
mean net integrated power per weight (kJkg−1),
frequency of power switched-off (times per treatment session),
total switched-off time (s),
average duration of each switched-off time (s),
vagina T50, which is T50 of overall intraluminal measurements within vagina lumen. The TX means the temperature that is exceeded by X percent of all temperature readings.
all lumina T50, which is defined as overall bladder, vagina and rectum lumen T50,
vagina T90, which is T90 of overall intraluminal measurements within vagina lumen. The T90 is representative for minimum temperature.
all lumina T90, which is defined as overall bladder, vagina,and rectum lumen T90,
CEM43°C T90 (cumulative equivalent minutes at 43°C based on T90 temperatures), which the formulation takes the following form:
Calculations and normalization of power and temperature
Following calculations were performed in order to find a positive correlation between normalized net integrated power and temperature. In the first step net integrated power (NIP) was calculated by:where Pfwd and Prfl are forwarded and reflected power (W), respectively, n is number of measurements and Δt is time interval between two measurements (s). In the next step, the pelvic area (A) was computed by:
where AP is the anterior posterior and Lat is the transverse dimension of the pelvis (cm), measured at the CT scan slice at the centre of the tumor in cranio-caudal direction. The net integrated power per pelvic area for individual treatments (x) follows then through NIP/Ax (kJcm−2). In the next step, we computed the mean of net integrated power per pelvic area for all treatments per individual patient. Then, percentage of normalized (nrm) net integrated power per pelvic area [nrm(NIP/A)] for the individual treatment was calculated by:
where the net integrated power per treatment is normalized to the average net integrated power over the whole series of treatments (n) for a patient.
Furthermore, percentage of normalized vagina T50 for each individual treatment (nrmVagT50x) was calculated using the following formula:where the vagina T50 per treatment is normalized to the average vagina T50 over the whole series of treatments (n) for a patient.
Finally, the relationship between the percentages of normalized net integrated power per pelvic area and normalized vagina T50 was evaluated for individual patients, and the results were summarized per patient graphically. The curves, the trend lines, the slopes of the trend lines and the correlation coefficients were computed. The curves with a slope of trend line ≥0.01 and the correlation coefficient ≥0.5 were considered positive correlation. Patients with less than three treatments were excluded from this part of analysis. Variations ≤2% in the power data were considered as noise.
Statistical analysis
The statistical analysis was based on the temperature-dose parameters, as provided in the ASCII files by RHyThM. Temperature measurements were available per patient, per treatment session, per probe, per mapping position, and per time point. A temperature below 37°C was considered indicative for the measuring point to be outside the lumen or to be the effect of local tissue cooling by one of the cold-water boli resulting in nonrepresentative low temperatures. Therefore, all these points were excluded from the analyses. The time points were scaled with respect to the starting time of the treatment. While computing averages, all observations were weighted equally. The T50 and T90 were calculated per patient, per treatment, and per lumen. The CEM43°C T90 was calculated per patient and per treatment. The averages and standard deviations were computed for the thermal dose parameters of different lumina (bladder, vagina, rectum, and all lumina) and different tissue types (normal tissue, tumor-contact, tumor-indicative, and overall). One-way ANOVA was used to compare the average power or temperature in different BSD configurations or weight-groups. In case of a significant difference, we used Bonferroni as a post-Hoc test to address the individual difference. A linear regression model was used to assess the effect of age, adjusted for weight, on the power and temperature indices. The p-values are two-sided at a significance level of α = 0.05. SPSS (version 12) was used for the statistical analysis.
Results
There were two types of limitations for the patient/treatment selection: (a) it was not possible to transfer the HT PDOS data of 11 patients to MSDOS, and (b) RHyThM failed to access the MSDOS data of 205 treatments. With these limitations and based on the selection criteria, in total 1672 HT treatments’ data of 444 primary cervical cancer patients, treated from March 1991 to December 2005, were available for the analysis. The 1672 DHT treatment sessions were delivered using four distinct power amplifiers of the BSD-2000 configurations all provided similar drive of the Sigma-60 applicator, i.e., 431 treatments by the first configuration, 105 treatments by the second, 203 treatments by the third, and 933 treatments by the fourth configuration ().
Table I. The number of primary cervical cancer patients/treatments as analyzed in this study, per weight-group and time-period, in which the Sigma-60 applicator and four configurations of the BSD-2000 system used by the Rotterdam Hyperthermia Group from 1991 to 2005.
The evaluations were done per BSD per weight-group, per BSD configuration, per weight-group, and over the time-period. Additionally, the evaluation on relations between normalized power and temperature is presented in the last section of the ‘Results’. In the following text the ‘bare’ power-indices refer to the mean forward power, maximum forward power, mean net power, and mean net integrated power. All reported numbers are per treatment session, unless otherwise mentioned.
Analysis per BSD per weight-group
In this part of the study, we evaluated the RF-power and temperature data obtained by each configuration of the BSD-2000 for the three weight-groups. The average values of the patient's characteristics, power and temperature data are presented in .
Table II. Average values of patients’ characteristics, RF-power, and temperature data per treatment.
First BSD configuration
The average of mean and maximum forward power increased from weight-group 1 to 3. The highest values for the derivative power related indices belonged to weight-group 2, with the lowest values for weight-group 3. Exception was the mean net integrated power for which the lowest value belonged to weight-group 1 ( (Panel A)). Significant differences (p ≤ 0.001)1 were seen for the ‘bare’ power indices in weight-group 1 vs. 2 and 3. Furthermore, a significant difference (p ≤ 0.003) was found for the mean net power per weight (or per pelvic area) in weight-group 3 vs. 1 and 2. The mean net integrated power per weight (or per pelvic area) in weight-group 2 was significantly different (p ≤ 0.005) from weight-groups 1 and 3.
The average frequency of switched-off was roughly equal for weight-groups 1 and 3, both higher than weight-group 2. The average of total switched-off time for weight-group 2 was lower than those for weight-groups 1 and 3, with the highest value for weight-group 1 ( (Panel A)). The average of each switched-off duration time was decreased slightly from 86, 75, to 67 s for weight-group 1 to 3, respectively. However, none of the differences between the switched-off variables was statistically significant.
The averages of vagina lumen T50, all lumina T50, and CEM43°CT90 decreased slightly from weight-group 1 to 3. The averages of vagina T90 and all lumina T90 were equal for weight-groups 1 and 2, both slightly higher than weight-group 3 ( (Panel A)). All temperature indices for weight-group 3 were significantly different (p ≤ 0.01) from the other two groups.
Second BSD configuration
The results are shown in (Panel B). The average of ‘bare’ power indices increased from weight-group 1 to 3. Again, the highest values for the derivative power related indices belonged to weight-group 2, with the lowest values for weight-group 3. Significant differences (p ≤ 0.001) were found for the ‘bare’ power indices in weight-group 1 vs. 2 and 3.
Similar to the 1st BSD, the average frequency of switched-off was roughly equal for weight-groups 1 and 3, where both were higher than weight-group 2. The average of total switched-off time for weight-group 2 was lower than those for groups 1 and 3, with the highest value for weight-group 1 ( (Panel B)). The average of each switched-off duration time was 57, 42, and 48 s for the weight-groups 1–3, respectively.
The averages of all temperature indices were roughly equal for weight-groups 1 and 2, while both were slightly higher than wei]ght-group 3 ().
Third BSD configuration
The results are presented in (Panel C). The average of forward power and net power increased from weight-group 1 to 3. The lowest and highest values of maximum forward power and mean net integrated power found for weight-groups 2 and 3, respectively. The derivative power related indices decreased slightly from weight-group 1 to 3. Significant differences (p ≤ 0.03) were found between ‘bare’ power indices for weight-group 3 vs. 1 and 2. Furthermore, significant differences (p ≤ 0.03) were found for the derivative power related indices between weight-group 1 vs. 2 and 3.
The average frequency of switched-off was roughly equal for weight-groups 1 and 3; both were slightly lower than weight-group 2. The average of total switched-off time for weight-group 2 was higher than those for groups 1 and 3, with the lowest value for weight-group 3 ( (Panel C)). The average of each switched-off duration time was 37, 37, and 35 s for the weight-groups 1–3, respectively, all shorter than those for the 2nd BSD configuration.
The averages of vagina T50, all lumina T50, and CEM43°CT90 decreased slightly from weight-group 1 to 3. The averages of vagina T90 and all lumina T90 were equal for the three weight-groups ( (Panel C)). No difference was found for the all temperature indices in the three weight-groups, except for all lumina T50 of weight-group 1, which was significantly different (p = 0.008) from weight-group 3.
Fourth BSD configuration
See (Panel D). The average of the ‘bare’ power indices increased from weight-group 1 to 3. In contrast, the average for the derivative power related indices decreased from weight-group 1 to 3. All, except one, power indices of weight-group 1 were significantly different (p ≤ 0.03) from weight-group 2 and 3 as well as for weight-group 2 vs. 3. The exception was the maximum forward power for which significant difference (p < 0.001) was seen between weight-group 3 vs. 1 and 2.
The average frequency of switched-off was roughly equal for weight-groups 2 and 3, which both were slightly higher than group 1. The average of total switched-off time for weight-group 2 was slightly higher than those for weight-groups 1 and 3. The average of each switched-off duration time was decreased slightly from 39, 35, to 34 s for weight-group 1–3, respectively. Significant differences (p ≤ 0.005) were found for the frequency of switched-off in weight-group 1 vs. 2 and 3. No difference was seen for the total switched-off and each switched-off duration time in the three weight-groups.
The averages of all temperature indices were decreased slightly from weight-group 1 to 3 ( (Panel D)). All temperature indices for weight-group 1 were significantly different (p ≤ 0.02) from the other two groups. Additionally, the all lumina T50 and CEM43°CT90 were significantly different (p ≤ 0.05) for weight-group 2 vs. 3.
Comparing the four BSD configurations used to treat similar weight-groups
In this part of the study, we compared the variables from similar weight-groups treated with different BSD configurations. The statistical analysis presented here is limited to the following variables: mean net integrated power, mean net integrated power per weight, total switched-off time, (assuming that they are representative for all other power indices), and all five temperature indices.
Comparing the mean net integrated power for weight-groups 1, significant differences (p ≤ 0.03) were found for the 3rd BSD vs. the other three configurations. When we compared weight-groups 2, a significant difference (p ≤ 0.003) was found for configuration 1 vs. 3 and 4, also for configuration 2 vs. 4. In comparison for weight-group 3, a significant difference (p ≤ 0.001) was found only for the 1st BSD configuration vs. the 4th. shows an overview of the net integrated power for the three weight-groups as function of the BSD configuration.
Figure 1. Average of (a) net integrated power, (b) net integrated power per weight, and (c) vagina T50 in the three weight-groups treated with the four configurations of the BSD-2000 and Sigma-60 applicator.
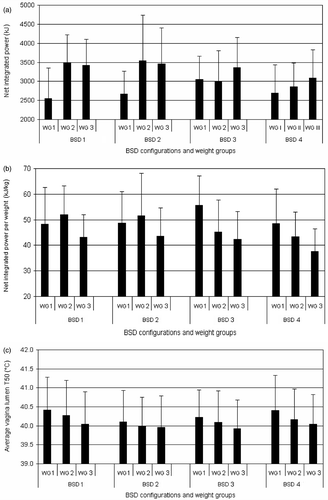
Comparing the mean net integrated power per weight for weight-groups 1, a significant difference (p ≤ 0.02) was found for the 3rd BSD configuration vs. the other three. Comparison of weight-groups 2 shows a significant difference (p ≤ 0.03) for configuration 1 vs. 3 and 4, as well as for configuration 2 vs. 4.
In comparison for weight-groups 3, a significant difference (p ≤ 0.01) was found for the 4th BSD vs. the other three configurations. shows an overview for the net integrated power per weight for the three weight-groups treated by the four BSD configurations.
Comparing the total switched-off time in weight-groups 1 shows a significant difference (p ≤ 0.03) for configuration 4 vs. the other three. Similarly, when we compared weight-groups 2, a significant difference (p ≤ 0.03) was found among configuration 4 vs. the other three, as well as for configuration 1 vs. 3. For weight-groups 3, again significant difference (p < 0.001) was found for the 4th BSD vs. the other three configurations.
With one exception no statistical significant difference was found for the achieved temperatures among similar weight-groups treated by different BSD configurations (). Only a significant difference (p = 0.02) was found for the all lumina T90 in weight-groups 2 treated by the 1st and 4th BSD ().
Analysis per BSD configuration
In this part of the study, the analysis was performed for the four BSD configurations regardless of the weight-group. The average values of the power and temperature data are shown in . The power indices showed small variation (±10%) over the four BSD configurations. The lowest values of mean and maximum forward power belonged to the 1st BSD (647 ± 163 W and 803 ± 221 W, respectively); the highest values of these variables to the 3rd BSD (711 ± 135 W and 876 ± 181 W, respectively). Significant different (p ≤ 0.01) was seen for the mean and maximum forward power of the 1st BSD vs. the other three configurations. The averages of mean net integrated power were almost equal for the configurations 1–3 (2981–3125 kJ), but for the 4th configuration they seem to be lowest (2889 ± 723 kJ). The average of mean net power per weight was 9.2–9.4 Wkg−1 for BSD 1–3 and 8.7 ± 1.9 Wkg−1 for the 4th BSD. The average of mean net integrated power per weight was 47.6–48.6 kJkg−1 for BSD 1–3 and 42.8 ± 11.4 kJkg−1 for the 4th BSD. Similarly, the lowest values of mean net power (or mean net integrated power) per pelvic area belonged to the 4th BSD, 0.94 ± 0.2 Wcm−2 (or 4.6 ± 1.4 k Jcm−2), respectively. Significant differences (p ≤ 0.04) were found for the mean net power per weight, mean net integrated power per weight, and mean net integrated power per pelvic area for the 4th BSD vs. other three configurations.
Table III. Average values of patients’ characteristics, RF-power, and temperature data per treatment for the four configurations of the BSD-2000.
Remarkably, the average frequency of power switched-off increased 4-fold from 2.8 times/treatment session for the 1st configuration to 11.5 for the 4th BSD (). The average of total switched-off time increased from 214 s for the 1st BSD to 410 s for the 4th. In contrast, the average of each switched-off time reduced from 76, 53, 36, to 36 s, respectively. The switched-off variables for the four BSD were significantly different (p ≤ 0.04).
The range of average vagina T50 for the four BSD configurations was 40–40.3°C. It was 40.4–40.6°C for the all lumina T50. The average CEM43°CT90 was in the range of 1.0–1.3 min (). Additionally, the average of vagina T90 for the four BSD was 39.4–39.5°C and 39.7–39.8°C for all lumina T90. No relation was found between temperature indices and BSD configuration.
Analysis per weight-group
This part of the analysis was performed for the three weight-groups regardless of the BSD configuration. The average values of power and temperature data are listed in . The average of mean forward power increased from 609 ± 132 W for weight-group 1 to 751 ± 139 W for weight-group 3. The average of maximum forward power also increased from 768 ± 189 W for weight-group 1 to 934 ± 185 W for weight-group 3. This pattern of increase was also found for the averages of net power and net integrated power (). However, the averages of power indices per weight (or per pelvic area) had different patterns from the ‘bare’ power indices. They were roughly equal for weight-groups 1 and 2, both higher than weight-group 3 (see ). Significant differences (p ≤ 0.001) were seen for all ‘bare’ power indices, net power per weight, net integrated power per weight, as well as net integrated power per pelvic area for the three weight-groups ().
Figure 2. Averages of (a) net integrated power, (b) net integrated power per weight, and (c) vagina T50, in primary cervical cancer patients for the three weight-groups (WG I: W < 61 kg, WG II: 61 ≤ W ≤ 70 kg, WG III: W > 70 kg).
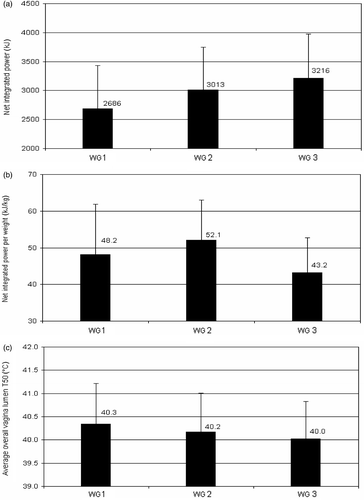
Table IV. Average values of patients’ characteristics, RF-power, and temperature data per treatment for the three weight-groups. Numbers in parentheses show 1 SD.
The highest average of frequency of power switched-off was 9.7 ± 6.6 times/treatment session for weight-group 2, while it was 7 ± 5.6 and 8.8 ± 6.1 times/treatment session for weight-groups 1 and 3, respectively (). The average of each switched-off time reduced from 46 s for the 1st weight-group to 37 s for weight-groups 2 and 3. The averages of total switched-off time were 322, 358, and 324 s for weight-groups 1–3, respectively. Significant differences were found for the frequency of switched-off between weight-group 1 vs. 2 and 3 (p ≤ 0.001) and weight-group 2 vs. 3 (p = 0.05).
The averages of vagina T50 decreased slightly from 40.3 to 40.0°C for weight-group 1 to 3 (). Similarly, the all lumina T50, was decreased slightly from 40.6 to 40.3°C. The average of CEM43°CT90 decreased from 1.3 min for the 1st group to 0.9 min for the 3rd group. The vagina T90 and all lumina T90 also decreased slightly from weight-group1 to 3 (). Although significant differences (p ≤ 0.04) were found for the temperature indices among the three weight-groups, their relevance is small.
Analysis over the time-period
This analysis was performed regardless of the BSD configuration and the weight-group. The yearly average of the power and temperature parameters for the 15 years are shown in . The lowest values for the averages of mean forward power (457 ± 106 W), maximum forward power (567 ± 126 W), and net power (412 ± 98 W) were seen in the year 1995, however, the lowest mean net integrated power (1505 ± 518 kJ) was in 1991. In the years 1991, 1995, and 2002 nearly all other power related variables were lower than those for the other years. The highest values of power indices were spread over the years 1992 to 2004.
Table V. Average values of patients’ characteristics, RF-power, and temperature data per treatment over the 15 years application of loco-regional deep hyperthermia by the Rotterdam Hyperthermia Group.
The averages of net power and net integrated power increased during the first 3 years using the 1st BSD, however, for the other years these values were almost constant. respectively show the average net integrated power and net integrated power per weight over the years for the four BSD configurations.
Figure 3. Average of (a) net integrated power (b), net integrated power per weight, and (c) vagina T50, over the years in primary cervical cancer patients treated with the four configurations of the BSD-2000 and Sigma-60 applicator.
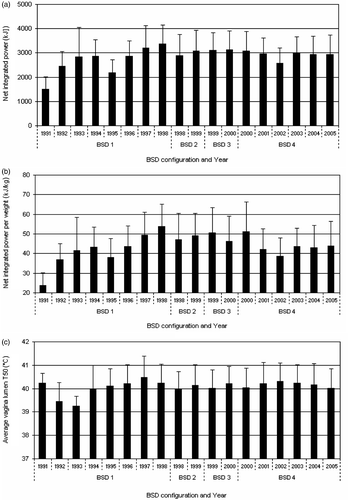
The lowest T50's were achieved in 1993 (vagina T50 39.3 ± 0.4°C, all lumina T50 39.6 ± 0.3°C). The minimum values for T90's were found in 1992 (vagina T90 38.8 ± 0.9°C, all lumina T90 39 ± 0.8°C). The lowest value for CEM43°CT90 (0.3 ± 0.2 min) was seen in 1993. The highest values of average temperature indices were seen in 1997. shows the average of vagina T50 over the years for the four BSD configurations. The average of achieved T50 was low during the 2nd and the 3rd years of the study, however, for the other years using the four BSD configurations it was almost constant.
During the years 1991–1996 the number of primary cervical cancer patients referred to us were low (mean 6.8 patients per year, 25.7 treatments per year). These numbers were much higher during the years 1997–2005 (mean 44.8 patients per year, 168.7 treatments per year). Therefore, we discriminated between the first 6 years, ‘study treatments time-period’, and the last 9 years, ‘standard treatments time-period’.
In the first 6 years, there was a large variation in the yearly averages of power-related variables; however, in the last 9 years it was much lower. For instance, the range of average mean net power was 412–643 W for the 1st time-period and 518–613 W for the 2nd time-period. The variations of the temperature indices were also different for the two time-periods. For example, during the 1st time-period the range of the average vagina T50 and T90 were 39.3–40.2°C and 38.8–39.5°C, respectively; whereas these values were 40–40.5°C and 39.3–39.7°C for the 2nd time-period, respectively ().
The average values of power and temperature data for each time-period are also summarized in the (7th and last rows). Comparison between the average values showed that in the 2nd time-period the power-related parameters were 11–18% higher than those for the 1st time-period. The average frequency of RF-power switched-off was remarkably higher (2.6-fold) in the 2nd time-period (3.4 vs. 8.9 times/treatment session). Additionally, the average of total switched-off time was also 28% higher in the 2nd time-period (266 vs. 341 s). In contrast, the average of each switched-off duration time was substantially lower in the 2nd time-period (75 vs. 44 s). shows the frequency of RF-power switched-off and the average of each switched-off duration time over the 15 years. Finally, the averages of the T50's in the 2nd time-period were only 0.2°C higher than those for the 1st time-period. The difference was 0.3°C for the averages of T90's and 0.1 min for the CEM43°CT90's.
Relation between power and temperature
Based on the selection criteria, 329 patients were eligible for this part of the analysis. Evaluation of relationship between the normalized net integrated power per pelvic area and vagina T50 showed various (poor to strong) correlations: in the 1st weight- group, positive correlation or trend was found in 40.4% (44 of 109 patients), negative correlation in 18.3% (20 patients), and no correlation in 41.3% (45 patients). In the 2nd weight-group, 40.6% (43 of 106 patients) had positive correlation or trend, 11.3% (12 patients) negative, and 48.1% (51 patients) no correlation. In the 3rd weight-group, the correlation was positive in 39.5% (45 of 114 patients), negative in 8.8% (10 patients), and no correlation in 51.8% (59 patients).
In summary, the correlation was positive in 40%, negative in 13%, and no correlation in 47% of the patients. Overall, in the three weight-groups the average value of the positive correlation coefficients was 0.7 (range 0.5–0.99) (see as an example).
Discussion
Since we started applying loco-regional DHT in 1990 with the first BSD-2000 configuration, the practice and environment in our department has undergone several substantial changes. Examples of these changes are growing clinical and technical experience, treatment protocols, introduction of newly developed equipment, etc. Each of these changes may have had its’ own effect on the treatment. In this study a first general analysis is performed to identify whether heating quality was affected by some of these changes and patient specific characteristics. Overall, the current study shows our ability to reproducibly heat advanced cervical tumors to 40–40.5°C with the BSD-2000 system and the Sigma-60 applicator, whereby the individual patient's characteristics (physical condition and motivation) appear to be critical for the achievable quality of the HT. The later is however a common phenomenon for every treatment modality (surgery, RT, ChT, etc.).
Experience and treatment strategy
During the first 6 years of the study, the power indices increased slightly. For this period, we noticed that power prescription changed with the growing experience of the staff-members and improvement of treatment protocols. Both aspects certainly affected the power settings. During the first 2–3 years the staffs spent large parts of their time and effort on training, learning, teaching, and developing the physical and clinical aspects of the technique for loco-regional deep heating. Especially, the understanding of when the maximum tolerance of each individual patient is reached requires a learning period. Projecting the development of the learning curve of our clinical experience we believe that a HT-technician or physician needs to treat 10–20 patients before she/he has sufficient experience to work independently.
Furthermore, during 1996–1999 we gradually changed our treatment strategy. Initially, we decreased the output power with 100 W after any patient's pain complaints; perform SAR-steering and start increasing the power again. With our growing experience we changed the strategy and refrained from decreasing the forward power at each complaint. Instead of reducing the power following a pain complaint caused by a too high temperature, we directly adjusted the treatment settings (phase or amplitude) to decrease energy deposition at the location indicated by the patient. Only when we found that phase, amplitude, and frequency steering were not able to overcome the power limiting complaints, it was decided to reduce the total forward power.
The change in treatment strategy was very well reflected by the very large variation in the frequency of RF-power switched-off over the 15 years. As is shown in , the number of switched-off is about six-fold higher in 2005 compared to 1996, this data is also presented in table A. However, at the same time the average duration of each switched-off reduced from 86 s in 1996 to 41 s in 2005. The result of an increased frequency of power switched-off on the temperature is complex. An increase of the total switched-off time results in a drop of the total delivered energy to the patient. Whether this will result in lower measured temperatures in the target volume, depends on the ratio of heat removal for the target and hot-spot location (perfusion difference between tumor and the tissue at the hot-spot location).
System upgrade
In the last 9 years, excluding 2002 for reasons as explained below, the average power indices showed a variation of less than 15%. For example, the yearly average of mean net integrated power was in the range of 2932–3209 kJ, which provided the vagina T50 in a range of 40.0–40.5°C. When evaluating the relevance of the variation in power input it is important to consider the accuracy of the RF-power readings of the systems. For the first three BSD-2000 configurations, the RF-power to the applicator was measured with Bird Power meters (500 W FS for forward power). In an extensive study on quality assurance for DHT, Hornsleth et al. Citation[23] showed that the accuracy of these meters was rather poor and depends strongly on the power output level. From this study it can be concluded that at the clinical-realistic power levels of 100–250 W per channel (total power 400–1000 W) the accuracy varies between ±10% and ±30%. For the 4th configuration the power reading system was substantially improved using micro-strip bi-directional couplers integrated in the amplifier design and for the PDOS operated system the power reading accuracy is about ±20% Citation[24]. Earlier Lee et al. Citation[25] demonstrated that adding a high performance vector voltmeter system is required to obtain an accuracy of RF-power measurement below 10%. Hence, given the accuracy of the standard system we may conclude that we are able to reproducibly apply loco-regional DHT to the primary cervical cancer patients.
As explained before, during the 15 years of patient treatment there was a gradual difference in the treatment strategy moving towards more frequent and shorter lasting power switched-off intervals. As the electronic design of the four BSD configurations differed minimally, we consider that the 3–8% lower net integrated powers that were found for the 4th BSD compared with the other three configurations could be attributed to the shift in strategy regarding frequency and duration of switched-off (). The largest difference (8%, 236 kJ) for the mean net integrated power is between the configurations 3 and 4 (). Simple calculations show that 80 kJ, i.e., one-third, is caused by the difference in total switched-off times (137 s) multiplied by the mean net power for these two configurations. After correction for this switched-off effect, the average net integrated power applied with the fourth system is in the same range as applied by the other three systems. At the same time it is noted that the temperature does not drop suggesting that we indeed are applying equal levels of RF-power.
Weight effect
An overall view to the three weight-groups shows that the power indices per weight (or per volume) were significantly different (p < 0.001) for the light-, medium-, and heavy- weight patients. As shows, despite all our efforts, the power delivered per kg for weight-group 3 is considerably lower than those for the other two groups. As expected, the achieved temperature in weight-group 3 is also lower than those for the other two groups. It is interesting to note that the drop in the net integrated power per weight from weight-group 1 to 3 () is comparable to the drop in the temperature from weight-group 1 to 3 () [Δ = 11.5% vs. 10% (temperature reference: 37°C), respectively]. This results confirms our intuitively expectation that heavy weight patients are more difficult to be heated to the goal temperature.
A more detailed analysis was performed on power and temperature data of 2001–2005. During these 5 years the averages of mean net integrated powers are not similar, i.e., 2963, 2577, 2998, 2932, and 2946 kJ, respectively (), however, the average of the achieved temperatures, e.g. vagina T50, are almost similar, i.e., 40.2, 40.3, 40.2, 40.2, and 40.0°C, respectively (). We checked the patients’ weight to find out an overall effect of weight on the power and temperature. As shows, in these years the averages of weight are different, i.e., 72.5, 67.7, 69.4, 68.9, and 70.2 kg, respectively, with 2002 being the lowest. For the last 5 years of the study we also evaluated the overall effect of patients’ age on the mean net integrated power per weight. Furthermore, we found a tendency for applying lower power to older patients in the three weight-groups, although the correlation coefficient was low. On the other hand, a tendency for higher vagina T50 was found for older patients. It will be interesting to investigate in more details on a small group of patients to see whether the lower weight of the elderly patient or whether anatomical and physiological differences might explain these findings.
Evaluation over the time-period
From 1996 onwards, the combination of RT plus HT became more and more accepted as a standard treatment modality for cervical carcinoma in the Netherlands and subsequently the number of patients and treatments per year in the last nine years was about 6-fold higher than in the years prior to 1996. We therefore divided the evaluation of the relationship between power and temperature in two time-periods: 1991–1996 and 1997–2005.
The larger variation in the yearly average of the achieved T50's in the first 6 years compared to the last 9 years, 0.9 vs. 0.5°C, is explained completely by the low number of patients (mean: 7 pts. per year) in the 1st time-period. The achieved T50's by the four BSD configurations showed the four systems have provided relatively low temperature in the treatment area. Clearly, the achieved temperature increase depends on many (uncontrollable) physiological parameters such as perfusion, regulation of perfusion, systemic temperature, thermal regulation of the whole organism, etc. Citation[26]. The range of yearly average temperature in the primary cervical cancer patients in this study was 39.3–40.8°C. The recent discussion on which temperatures are optimum for the achievement of maximum treatment results discriminates between so-called ‘mild’ HT, the temperature range that has no negative effect on perfusion and oxygenation, and higher thermal doses that may compromise perfusion Citation[27]. Since the T50's that we achieved during treatment are far below the level from which one might expect a negative influence on oxygenation, our basic assumption is that, within this range, higher temperatures will result in a better treatment quality.
It is of relevance to mention here that the relatively moderate temperatures result in a considerable enhanced clinical outcome for the combined treatment of RT+HT in comparison to RT-alone as has been demonstrated by the results of the Dutch DHT phase III trial, 51% vs. 27% three years overall survival, respectively. We remind that the objective of the current study is to investigate the quality of the HT treatment based upon the temperatures achieved in the target region. Our group is currently also investigating the potential relationship between treatment outcome and target temperature. The scope of that study, including the wide range of prognostic factors other than temperature, is such that the results will be reported in a separate publication. As the measured temperatures in treatment field are the only directly assessable indicators of the achieved quality of the HT treatment we consider a separate analysis of the temperature and power parameters highly warranted and of great importance for further improvement of the DHT procedures.
Relation between power and temperature
Earlier, Wust et al. Citation[26] and Paulsen et al. Citation[28] have shown in theoretical studies the importance to optimize the SAR distribution in pelvic tumors as the maximum increase of pelvic temperature was strongly related to maximum increase of power. In these studies, Wust et al. Citation[26] and Paulsen et al. Citation[28] concluded that higher power results in higher temperatures. In a recent publication Fatehi et al. Citation[22] observed a positive correlation between normalized net integrated RF-power and vagina T50. The later can be seen as a first provisional experimental indication of a possible relation between input power and temperature, as mentioned by Wust et al. Citation[26] and Paulsen et al. Citation[28]. This result stimulated us to do more in-depth investigation of this potential relation for the larger patient population of the present study. Hereto, we looked at the correlation between net power (or net integrated power) and temperature, e.g. vagina T50, but no correlation was found between these basic parameters. When we evaluated this relation between power indices per pelvic area vs. temperature, again no correlation was seen. In an attempt to look at the patient individual level, we normalized the power and temperature indices over the whole treatment series for each patient and computed the relationship between them, as we experienced it in the earlier study Citation[22]. The positive correlation found in 40% of the patients indicates that higher power creates higher temperature, which is in line with the earlier results from modeling studies. However, as the correlation is found in a relatively small proportion of the patients, it also indicates that other individual patient's characteristics are still very important for the power prescription.
Limitations
When we looked at the average power-related data over the 15 years, we recognized that the lowest mean net power or net integrated power was applied in the years 1991, 1995, and 2002. Taking into account that 1991 is the second year of the application of DHT by our department, the low power for this year finds some justification by the fact that the experience of the HT staff-members was still growing. Additionally, as in this year only two patients (six treatments), with accessible data, were treated; the low power can also be explained by patient specific characteristic such as weight. To find the causes for the low power in the years 1995 and 2002, we checked the documentation. For 1995 the most plausible explanation for the low power values is low patients’ weight of 58.4 kg on average, being the lowest over the 15 years (the average of all patients’ weight was 67.4 kg). For the year 2002 the situation was more complicated. In this year there were several instances in which the amplifier or the software stopped working during the treatments, requiring a reboot of the whole system. As RF-interference to the computer was more likely to occur at high power, thus, losing the treatment data for these patients means that in 2002 the treatments with low power are relatively over represented. Another noticeable reason is that in this year the Sigma-Eye applicator went out of service for a 4–5 month period. Hence, in this period even patients, who were eligible for a Sigma-Eye treatment, i.e., in general smaller and of lower weight, were treated with the Sigma-60.
This study is the first attempt to find relationships between power and temperature respecting the weight and/or age. The results encourage us to further investigate these aspects within a smaller group of patients but more focused on the individual patient level and the 4th configuration of the BSD-2000.
Conclusions
In this study, we showed that the BSD-2000 Sigma-60 is able to reproducibly generate moderate loco-regional deep heating to 40–40.5°C, as measured intraluminally, in all 444 patients. Hence, the good clinical results as reported by van der Zee et al. Citation[5] have been obtained at relatively moderate temperatures.
The four distinct power amplifiers of the BSD-2000 configurations all provided similar drive of the Sigma-60 applicator. Throughout all upgrades over the 15 years, the heating pattern did not change significantly.
Over the 15 years the achieved temperatures were relatively stable, even though we changed our strategy (switched-off modulations) to apply the RF-power to the patient and the increased personnel experiences.
For heavy weight patients, higher power levels can be applied but the resulting power per unit weight (W/kg) is lower than that of low or medium weight patients. As a conclusion, lower target temperatures are achieved in heavy weight patients and thus, in general, heavy weight patients are less easy to heat adequately.
Power prescription still depends to individual patient's characteristics.
Acknowledgments
This work was supported by the Dutch Cancer Society, grant DDHK 2003-2884. The first author was supported financially by the Shahrekord University of Medical Sciences (related to the Iranian Ministry of Health, Treatment, and Medical Education). The authors would like to thank L. Verloop, L. Groenendijk, A. Ameziane, and other hyperthermia staff-members of the Daniel den Hoed Cancer Centre in Rotterdam for their technical assistance. We also thank A.Dehghan for his statistical consults.
Notes
Notes
[1]. In the following text range of p-values for the different group testing is omitted and represented by p ≤ 0.0X where 0.0X represents the highest value and indicating that all other p-values were smaller.
References
- Wust P, Hildebrandt B, Sreenivasa G, Rau B, Gellermann J, Riess H, Felix R, Schlag PM. Hyperthermia in combined treatment of cancer. Lancet Oncol 2002; 3: 487–497
- Colombo R, Da Pozzo LF, Salonia A, Rigatti P, Leib Z, Baniel J, Caldarera E, Pavone-Macaluso M. Multicentric study comparing intravesical chemotherapy alone and with local microwave hyperthermia for prophylaxis of recurrence of superficial transitional cell carcinoma. J Clin Oncol 2003; 21: 4270–4276
- Jones EL, Oleson JR, Prosnitz LR, Samulski TV, Vujaskovic Z, Yu D, Sanders LL, Dewhirst MW. Randomized trial of hyperthermia and radiation for superficial tumors. J Clin Oncol 2005; 23: 3079–3085
- Verwaal VJ, van Ruth S, de Bree E, van Sloothen GW, van Tinteren H, Boot H, Zoetmulder FA. Randomized trial of cytoreduction and hyperthermic intraperitoneal chemotherapy versus systemic chemotherapy and palliative surgery in patients with peritoneal carcinomatosis of colorectal cancer. J Clin Oncol 2003; 21(20)3737–3743
- Van der Zee J, González GD, van Rhoon GC, van Dijk JD, van Putten WL, Hart AA. Comparison of radiotherapy alone with radiotherapy plus hyperthermia in locally advanced pelvic tumours: a prospective, randomised, multicentre trial. Dutch Deep Hyperthermia Group. Lancet 2000; 355: 1119–1125
- Van der Zee J, van Rhoon GC. Cervical cancer: Radiotherapy and hyperthermia. Int J Hyperthermia 2006; 22: 229–234
- Franckena M, Stalpers LJA, Koper PCM, Wiggenraad RGJ, Hoogenraad WJ, van Dijk JDP, Wárlám-Rodenhuis CC, Jobsen JJ, van Rhoon GC, van der Zee J. Long-term improvement in treatment outcome after radiotherapy and hyperthermia in locoregionally advanced cervix cancer: An update of the Dutch Deep Hyperthermia Trial. Int J Radiat Oncol Biol Phys 2007, (in press)
- Vernon CC, Hand JW, Field SB, Machin D, Whaley JB, van der Zee J, van Putten WL, van Rhoon GC, van Dijk JD, González GD, et al. Radiotherapy with or without hyperthermia in the treatment of superficial localized breast cancer: Results from five randomized controlled trials. Int J Radiat Oncol Biol Phys 1996; 35: 731–744
- Stauffer PR. Evolving technology for thermal therapy of cancer: Review. Int J Hyperthermia 2005; 21: 731–744
- Egawa S, Ishioka K, Kawada Y. Trials of combined radiation and hyperthermia with various heating modalities in cancer therapy. Radiat Med 1988; 2(4)260–264
- Van Rhoon GC, van der Zee J, Broekmeyer-Reurink MP, Visser AG, Reinhold HS. Radiofrequency capacitive heating of deep-seated tumours using pre-cooling of the subcutaneous tissues: results on thermometry in Dutch patients. Int J Hyperthermia 1992; 28: 843–854
- Sapozink MD, Frederic A, Gibbs JR, Gates KS, Stewart R. Regional hyperthermia in the treatment of clinically advanced, deep seated malignancy: Results of a pilot study employing an annular array applicator. Int J Radiat Oncol Biol Phys 1984; 10: 775–786
- Sapozink MD, Cetas T, Corry PM, Egger M, Fessenden P. The NCI Hyperthermia Equipment Evaluation Contractors’ Group. Introduction to hyperthermia device evaluation. Int J Hyperthermia 1988; 4: 1–15
- Sapozink MD, Frederic A, Gibbs JR, Gibbs P, Stewart R. Phase I evaluation of hyperthermia equipment—University of Utah Institutional Report. Int J Hyperthermia 1988; 4: 117–132
- Turner PF, Schaefermeyer T. BSD-2000 approach for deep local and regional hyperthermia: Clinical utility. Strahlenther Onkol 1989; 165: 700–704
- Turner PF, Tumeh A, Schaefermeyer T. BSD-2000 approach for deep local and regional hyperthermia: Physics and technology. Strahlenther Onkol 1989; 165: 738–741
- Fatehi D, de Bruijne M, van der Zee J, van Rhoon GC. RHyThM, a tool for analysis of PDOS formatted hyperthermia treatment data generated by the BSD2000/3D system. Int J Hyperthermia 2006; 22: 173–184
- Westermann AM, Jones EL, Schem BC, van der Steen-Banasik EM, Koper P, Mella O, Uitterhoeve ALJ, de Wit R, van der Velden J, Burger C, et al. First results of triple modality treatment by combining radiotherapy, chemotherapy and hyperthermia for treatment of stage IIB-III-IVA cervical cancer. Cancer 2005; 104: 763–770
- Bowman R. A probe for measuring temperature in radio frequency heated material. IEEE Trans MTT 1976; 24: 43–45
- Lagendijk JJW, van Rhoon GC, Hornsleth SN, Wust P, De Leeuw ACC, Schneider CJ, Dijk JDP, van der Zee J, van Heek-Romanowski R, Rahman SA, et al. ESHO quality assurance guidelines for regional hyperthermia. Int J Hyperthermia 1998; 14: 125–133
- Wielheesen DHM, de Bruijne M, Graveland WJ, van Rhoon GC, van der Zee J. Leg coverage with towels during regional deep hyperthermia treatment and its effect on pelvic temperature and temperature distribution. Int J Hyperthermia 2005; 21: 77–87
- Fatehi D, van der Zee J, van der Wal E, van Wieringen WN, van Rhoon GC. Temperature data analysis for 22 patients with advanced cervical carcinoma treated in Rotterdam using radiotherapy, hyperthermia and chemotherapy: A reference point is needed. Int J Hyperthermia 2006; 22: 353–363
- Hornsleth SN, Frydendal L, Mella O, Dahl O, Raskmark P. Quality assurance for radiofrequency regional hyperthermia. Int J Hyperthermia 1997; 13: 169–185
- Kongsli J, Hjertaker BT, Frøystein T. Evaluation of power and phase accuracy of the BSD Dodek amplifier for regional hyperthermia using an external vector voltmeter measurement system. Int J Hyperthermia 2006; 22: 657–671
- Lee WM, Ameziane A, van den Biggelaar AMC, Rietveld PJM, van Rhoon GC. Stability and accuracy of power and phase measurements of a VVM system designed for online quality control of the BSD-2000 (-3D) DHT system. Int J Hyperthermia 2003; 19: 74–88
- Wust P, Seebass M, Nadobny J, Felix R. Electromagnetic deep heating technology. Principles and practice of thermoradiotherapy and thermochemotherapy, MH Seegenschmiedt, P Fessenden, CC Vernon. Springer Verlag, Berlin 1995; 219–251
- Song CW, Park HJ, Lee CK, Griffin R. Implications of increased tumor blood flow and oxygenation caused by mild temperature hyperthermia in tumor treatment. Int J Hyperthermia 2005; 21: 761–767
- Paulsen K, Geimer S, Tang J, Boyse W. Optimization of pelvic heating rate distributions with electromagnetic phased arrays. Int J Hyperthermia 1999; 15: 157–187