Abstract
Purpose: The aim of this review article is to provide a concise overview of the pre-clinical development of electrochemotherapy (ECT), its present utility in clinical practice and to examine its potential application to therapeutic modalities in the future.
Results: Results from the ESOPE trial demonstrate an 85% objective response rate (ORR) in solid cutaneous and subcutaneous tumours of varying histologies, that would previously have been recalcitrant to conventional therapies. Experimentally, neoadjuvant immunogene therapy of primary cancers has been found to be effective against minimal residual disease in metastatic models. As such, combinations of electrogene delivery and electrochemothearpy offer exciting possibilities for both local and systemic control of heretofore incurable cancers.
Conclusions: Electrochemotherapy is a quick, safe, inexpensive treatment modality that has been shown to give consistently reproducible results in the treatment of solid cutaneous and subcutaneous malignant tumours. To date, its clinical license has limited its application to a palliative setting. Future work includes looking to extend this therapeutic profile to the management of primary tumours and earlier stage disease, as well as examining the potential for combining electrochemotherapy with gene and immunotherapies and developing novel electrode designs to facilitate the application of this treatment to internal cancers.
Introduction
Local control is a critically important part of the treatment of malignant solid tumours as it relieves symptoms, forestalls complications, facilitates responsiveness to systemic therapies and is often curative. Many cancers are resistant to multimodal treatments and there is a need for therapeutic innovations and discovery. The causes of intractability include advanced stage disease at presentation which limits the application or effectiveness of treatments, resistance of tumour cells to chemotherapy and radiation therapy, anatomical locations of the cancer that preclude complete excision or ablation by radiotherapy, and the presence of inter-current illnesses which either limit the application or adversely alter the risk and benefit of treatment. Locally recurrent disease particularly after optimal treatment of head and neck and breast cancer or malignant melanoma is difficult to manage and the unfortunate patients are consigned to live in despair for several months with obviously progressing painful, bleeding and malodorous tumours. A major problem for many patients with incurable tumours is their poor quality of life and the intrusion on their valuable remaining time that results from invasive or toxic therapies. In this context an ideal treatment of cancer should effectively control local disease, be applicable to a diversity of tumour types and anatomical locations, be effective against locally recurrent disease, facilitate multimodal and systemic therapies, be minimally intrusive and improve patient wellbeing and life expectancy by tumour control or cure.
Electrochemotherapy is a revolutionary treatment where the local application of pulses of electric current to tumour tissue render the cell membranes permeable to otherwise impermeant or poorly permeant (hydrophilic) anticancer drugs (such as bleomycin or cisplatin), thereby facilitating a potent localised cytotoxic effect Citation[1–5]. A major limiting factor in the clinical effectiveness of potential therapeutic agents such as hydrophilic drugs, macromolecules, DNA and certain peptides relates to their poor intracellular delivery. Methods that transiently destabilise the cell membrane overcome this physical barrier and allow impermeant drugs to passively enter the cell Citation[6],Citation[7]. The application of short, intense square wave pulses of direct current to tumours result in a 300–1000-fold enhancement of intracellular concentration and cytotoxicity of bleomycin Citation[8],Citation[9]. The optimal electropermeabilisation current alone does not affect the growth of tumours suggesting that permeabilisation of cells is transient and not injurious Citation[10]. The dose of bleomycin is about one twentieth of that used in conventional chemotherapy regimens and only has antitumour efficacy when combined with the electric current. Thus systemic toxicity and collateral injury is minimal, as cytotoxicity is restricted to the tissues covered by the applied field. Because the resulting cell death is by apoptosis there is minimal inflammatory reaction or systemic illness during involution of the tumour.
The first use of electrochemotherapy with bleomycin in humans was published in 1991 on head and neck tumour nodules Citation[10]. Since then, the area has advanced considerably in terms of the systems used and the cancers demonstrated to be suitable for treatment Citation[11–14]. A number of companies, notably IGEA (IGEA, Carpi, Italy) and Inovio (Inovio Biomedical Corporation, CA, USA) have developed pulse generators with approval for use in patients. Over the last decade, studies using these systems have established ECT to be safe and effective in treating all cutaneous and subcutaneous lesions. The system has proven effective in cases where cancers were either unsuitable or unresponsive to surgery, chemotherapy or radiotherapy.
Electrochemotherapy may be given with conventional anticancer treatments and experiences may be used to inform the development or to expand the utility of other therapies. This electrical protocol can be safely applied for the intracellular delivery of macromolecules including gene delivery of naked DNA into solid tumours and normal tissues, where cell viability for gene transcription would be important Citation[15],Citation[16]. It has been suggested that regression of tumours after electrochemotherapy induces anti-tumour immunological responses which may be amplified for systemic disease control. Administration of the cytokine IL-2 perilesionally during treatment has been shown to induce durable specific anti-tumour immunological responses resulting in regression of second tumours at distal sites Citation[17]. Sensitisation of solid tumours to ultrasound or radiation therapy has been reported for electrical treatment alone or for electrochemotherapy respectively suggesting alternative approaches to selected bulky tumours or radiation-resistant cancers Citation[18].
While all cancer types appear responsive to electrochemotherapy Citation[10–12], Citation[14],Citation[19],Citation[20] experience to date has been confined to cutaneous malignancies, often recurrent and metastatic after multimodal therapies. The treatment is minimally disturbing for patients, is easy to perform in an outpatient setting and is inexpensive. These encouraging clinical experiences promise the development of systems for treatment of intractable deep-seated malignancies. Electrode systems are currently under design to facilitate intraluminal, endoscopic or image-guided approaches and treatment of internal cancers Citation[21]. In this report we describe electrochemotherapy and review the clinical experience and potential for further development.
Cell permeabilisation: Electroporation
The cell membrane represents a physical barrier to the intracellular transfer of hydrophilic drugs, macromolecules, nucleotides and peptides. The phospholipid bilayer of the plasma membrane restricts the trans-membrane movement of hydrophilic and polar molecules, other than by specific channel or receptor-mediated assistance. The application of electric fields has been utilized to surpass this barrier and to permit the traffic of various molecules, including those that destabilize the cell membrane. This strategy is referred to as electroporation and involves the direct application of an electrical field to target tissue/tumours Citation[6],Citation[7]. Cell membranes, both in vitro and in vivo, are rendered transiently permeable or porous through the application of short high voltage electrical field pulses optimised to balance the reversible and irreversible effects of the procedure.
Mechanisms of electroporation
One of the longest-standing theories to explain the principle of electroporation is based on the formation of electropores, leading to the term electroporation Citation[22]. Studies suggest that exposure to a sufficiently long and strong electric field leads to the formation of hydrophilic pores whereby adjacent lipid molecules are re-orientated such that their hydrophilic heads are arranged pore-like, while their hydrophobic tails are embedded within the plasma membrane Citation[23]. Using this technique, a wide range of molecules, e.g., ions, peptides, oligonucleotides, RNA and DNA and hydrophilic drugs have been successfully delivered intracellularly Citation[24–27].
Electric pulse parameters
Permeabilisation can only occur in areas of the cell membrane where the internal transmembrane potential has surpassed a critical value Citation[28],Citation[29] normally between 200–300 mV, Citation[30],Citation[31]. Successful poration is dependent upon the field strength applied, E, and must exceed a critical value, Ep Citation[32]. Any increase in E above Ep leads to an increase in the permeabilised area of the cell membrane. The extent of permeabilisation is then determined by both the electric pulse number and duration Citation[33]. In the context of cell electropermeabilisation a number of electrical pulse parameters have been examined. Essentially, these can be reduced to two forms: exponentially decaying pulses and square wave pulses, of which only the latter allows for independent control of the pulse amplitude and length Citation[34]. In clinical practice, optimised pulse parameters (8 square wave pulses; amplitude 1300 V/cm; duration 10 µs; frequency 1Hz) are utilised to permeabilise tumour cell membranes. Using these pulse parameters, drug dosages that have minimal or no anti-tumour effectiveness demonstrate high (75%) complete response rates in treated tumour nodules Citation[35],Citation[36].
Clinical application: Systems employed
Two companies have developed clinically approved electroporation equipment (Cliniporator–IGEA; The MEDPULSER–Inovio) and associated electrodes. The electric field distribution is largely determined by the geometry of the electrodes and these can be divided into two different types: plate electrodes and needle electrodes. Plate electrodes are suited for use in skin or superficial lesions. Needle electrodes are either arranged in two parallel rows or in a circular (hexagonal) array. Unlike plate electrodes, these are impaled transcutaneously into the target tissue. Regardless of the type of electrode, the electric field is highest around and between the electrodes. In the case of plate electrodes, the electric field is substantially impeded by the resistance of the skin ().
Figure 1. The electrical fields generated through either needle (A) or plate electrodes (B) are demonstrated above, top. Plate electrodes generate a more homogenous field but their efficiency is affected by the high resistance encountered through the skin. In total a train of eight 100 µsec pulses (Frequency–5 MHz) are applied with a field strength of approximately 1300 v/cm.
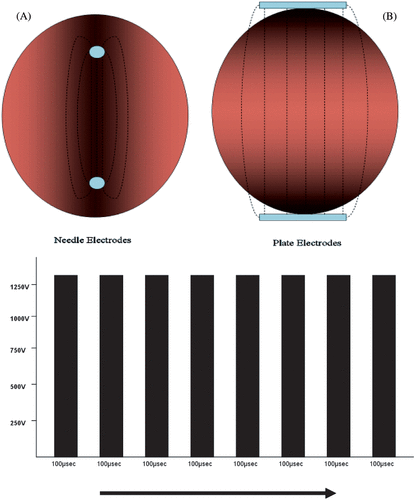
Physical impact: The vascular lock
While the effects of electropermeabilisation on the cell membrane have been well documented, it also appears to have a secondary effect on tumour vasculature Citation[25], Citation[37–39]. The electric pulses induce a state of hypoperfusion by causing local vasoconstriction at an arteriolar level, mediated by the sympathetic nervous system. While this effect is transient in normal tissue Citation[37], it exerts an immediate, severe hypoperfusion in the fragile abundant vasculature of tumour tissue lasting from 12 hours (electropermeabilisation alone) to 5 days (electrochemotherapy) Citation[40],Citation[41]. This finding mirrors the clinical observation that bleeding, ulcerated lesions cease to bleed following electropermeabilisation.
Several theories have been proposed to explain this phenomenon Citation[39]. The first is that electropermeabilisation has a direct effect on tumour endothelial cells. A second theory suggests that the high interstitial pressure associated with permeabilisation induces collapse of the vasculature structures surrounding the tumour. This effect on local vasculature has implications for the timing of administration of chemotherapeutic drugs. If the drug is administered following electropermeabilisation, the ‘vascular lock’ can restrict access to target tissue. This is the case for either systemic or local drug administration. On the other hand, if the drug were already present at the time of electropermeabilisation, the electric pulses should have the effect of trapping the drug within the electropermeabilised area, effectively creating a ‘vascular lock’. This would mean that the chemotherapeutic agent would essentially be trapped within the treated area, maintaining a high local concentration by transiently delaying the dispersion of the drug by the local vasculature. This effect has been confirmed in vivo using diffusion markers such as Cr-EDTA and Patent Blue Citation[42].
Electrochemotherapy
Appropriate drugs for electrochemotherapy
The flow of metabolites into and out of the cell interior is a process regulated by channels or transporters at the level of the cell membrane. An impermeant drug cannot cross the cell membrane because of its hydrophilic nature and lack of specific channels or transport proteins Citation[24]. Most commonly used chemotherapeutic agents are permeable molecules for which the cell membrane does not pose a barrier Citation[8],Citation[43]. Factors generally considered characteristic of drugs suitable for this therapy include localizing toxicity to the electroporated tissue, reducing systemic side effects, and logarithmically increasing drug efficacy. Two such drugs have been applied clinically, bleomycin and cisplatin. Bleomycin is an impermeant chemotherapeutic agent that possesses high intrinsic cytotoxicity. In vitro, bleomycin kills cells by two different mechanisms; mitotic cell death or pseudoapoptosis Citation[44], depending on the amount of bleomycin internalised in the electropermeabilised cells. These cell death mechanisms are linked to the ability of bleomycin to act intracellularly by causing single and double stranded breaks in DNA Citation[45–47]. Previous studies performed with both mice and rats have shown that, when bleomycin is administered in combination with electroporation, its effectiveness as an anti-tumour agent is greatly enhanced. These studies were performed with a variety of tumour types, including melanoma, hepatocellular carcinoma, lung carcinoma, breast carcinoma, fibrosarcoma, and glioma Citation[1],Citation[2],Citation[20],Citation[48],Citation[49]. The cytotoxicity of bleomycin was shown to be increased by up to 700-fold resulting in objective response rates as high as 90% and complete response rates as high as 85% Citation[8],Citation[9].
The cisplatinum complexes are another example of a poorly permeant drug whose toxicity is greatly enhanced when used in combination with electroporation. The mechanism by which cisplatin becomes internalised is still poorly understood. Both diffusion and facilitated transport have been implicated Citation[50]. In vitro results have shown that electropermeabilisation increases the cytotoxicity of cisplatin by a factor of between three Citation[51] and eight Citation[52], depending on experimental conditions. This increased cytotoxicity results directly from increased cisplatin uptake Citation[51].
Routes of administration: Bleomycin
Present day clinical trials utilise doses of intravenous bleomycin that are similar to those used in conventional chemotherapeutic regimens (15 000 IU/m2). As electrochemotherapy is often a once-only treatment however, the cumulative dose of bleomycin is much lower as compared to conventional chemotherapy, where multiple treatments are often necessary. As the systemic dose of bleomycin is one twentieth that of most conventional chemotherapeutic regimens, systemic side effects tend to be limited to nausea. The downside of intravenous drug administration however, is that a number of factors such as poor circulation or a poorly vascularised tumour can adversely affect treatment outcome by restricting the amount of the drug present at the tumour site. The decision to deliver the drug either systemically or via local application is based on the number, size and location of the tumour. Statistically results from the ESOPE study Citation[50] demonstrate that both delivery systems provide nearly equivalent results in terms of tumour response.
In an attempt to overcome this problem, it was hypothesised that the response rate could be increased using intratumoural (i.t.) administration of bleomycin. This would eliminate vascular factors as a negative influence. It would also expand the time window associated with treating multiple lesions by treating each site individually. As well as this, administration of bleomycin intratumourally would minimise the already greatly attenuated side effect and toxicity profile associated with chemotherapeutic doses used for electrochemotherapy. Although the dosage for intratumoural bleomycin (500 IU/cm3) and cisplatin (1 mg/cm3) is tumour volume dependent, unless the tumour is very large, or there are a large number of tumours requiring treatment, the overall dose tends to be smaller than that required intravenously Citation[53].
The optimal efficacy of electrochemotherapy occurs when a sufficient amount of drug is present at the tumour site during administration of the electric pulses. Previous studies by Domenge et al. in head and neck tumours in humans demonstrated that the greatest effect was observed when electroporation took place 8–28 minutes following the administration of bleomycin intravenously Citation[54]; intratumoural drug injection, however, requires that the pulses be delivered within 1 to 10 minutes Citation[12], Citation[55].
Anaesthesia
The decision to administer electrochemotherapy under general or local anaesthesia is dependent upon a number of factors. These include the site, size and number of lesions to be treated, as well as the general medical condition of the patient. In the case of head and neck tumours for example, treatment is often carried out under general anaesthesia. When smaller, discrete tumours are involved, e.g. chest wall metastases from a breast adenocarcinoma, treatment is carried out using local anaesthesia, often in combination with epinephrine. The adrenaline acts synergistically with the vascular effects of electroporation, causing local vasoconstriction and delaying systemic dissemination of the drug by the local vasculature.
Patient follow-up
Most patients who have electrochemotherapy are discharged home the same day. Relative indications for overnight admission include patients who have previously had adverse reactions to anaesthesia, those who are at high anaesthetic risk and patients who have mitigating social circumstances. Patients are recalled after a period of 4–6 weeks at which time they are examined by their clinician. The number of treatments required will be dependent upon the size, number and responsiveness of the lesions involved.
Response criteria
The WHO classification of complete response (elimination of the nodule), partial response (>50% reduction in tumour volume), no change (<25% increase/>50% reduction in tumour volume) and progressive disease (>25% increase in tumour volume) is utilised in an attempt to determine tumour response. This system is comparable to the RECIST system of response classification where complete response refers to complete disappearance of all targeted lesions; partial response where there is at least a 30% reduction in the sum of the longest diameter of targeted lesions; progressive disease which denotes a less than 20% increase in the sum of the longest diameter of targeted lesions; and stable disease where there is neither sufficient shrinkage to qualify for PR nor sufficient increase to qualify for PD.
Clinical utility of electrochemotherapy
Clinical experience to date with electrochemotherapy has been encouraging, with objective responses and effective symptomatic palliation achieved in a variety of cancers (). Due to technological limitations, current clinical experience has been confined to cutaneous malignancies usually recalcitrant to conventional therapies. Despite that all cancer types are sensitive, in a clinical setting, anatomical location may preclude delivery of an electroporation current. Electrode systems are only recently development for intraluminal delivery by endoscopic and laparoscopic systems or intravascular catheters Citation[21].
Figure 2. Treatment of a scalp metastatic lesion with electrochemotherapy. Images are representative of pre-treatment (A) and 3 months post-treatment follow-up (B).
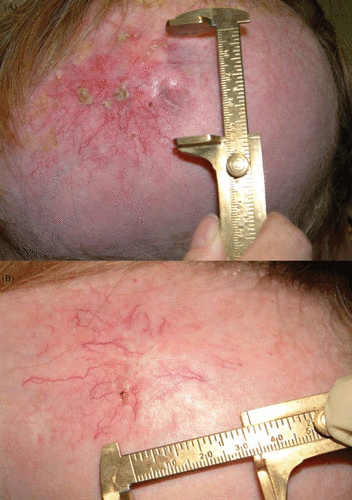
Figure 3. Clinical example of ECT effectiveness for palliative patients. (A) Chest wall recurrence of an inflammatory breast cancer prior to ECT treatment and unresponsive to systemic chemotherapy. ECT was applied to the whole chest wall once at every 3 months follow up (B, C and D). The ulcerated tissue shows a dramatic decrease in size and as a result large areas of re-epithelialisation are evident.
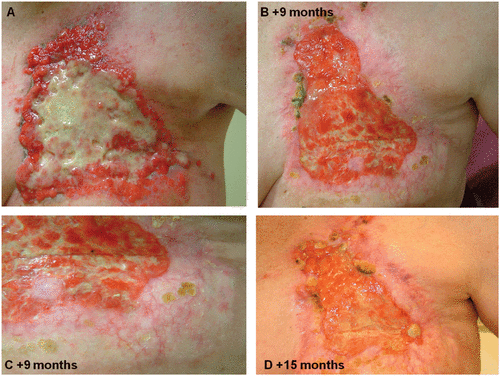
Until recently, the various studies involving electrochemotherapy were utilizing a variety of protocols with different doses of chemotherapy, pulse parameters and pulse generators in conjunction with different electrode types. In an attempt to rectify this, a European study (ESOPE, European Standard Operating Procedures of Electrochemotherapy) was launched with the aim of standardising the techniques involved in the clinical application of electrochemotherapy based on the experience of leading European cancer centres on electrochemotherapy. Treatment response after electrochemotherapy according to tumour type, drug used, route of administration and types of electrodes were tested Citation[35],Citation[56],Citation[57]. Seventy-two patients with a total of 324 nodules were included in the trial which ran from March 2003 to April 2005.
The four centres involved were the Institute Gustave-Roussy (IGR) Villejuif, France, Institute of Oncology Ljubljana (OI), Ljubljana, Slovenia, University of Copenhagen at Herlev Hospital (HH), Herlev, Denmark and the Cork Cancer Research Center (CCRC), Mercy University Hospital, Cork, Ireland. The four participating centres prepared protocols for treatment and pain control based on previous experience as well as a list of indications for electrochemotherapy. Cutaneous and subcutaneous tumour nodules in patients with progressive disease were treated by electrochemotherapy using bleomycin (given intravenously or intratumourally) or cisplatin (given intratumourally). The ESOPE project also anticipated the use of a new, CE approved, medical grade, electric pulses generator Cliniporator™. This device allows for the monitoring and storage of current and voltage parameters as well as pulse parameter settings, both for immediate control and later retrieval and analysis. The results of all clinical trials up to and including the ESOPE trial are included in .
Table I. Tumours treated with electrochemotherapy in clinical trials.
Developmental perspective
Now that the efficacy, safety and clinical utility of electrochemotherapy has been established for certain malignant tumours, there is a continued impetus to modify and further develop the clinical potential of this technology. The only limitation to the delivery of this treatment would be the inability to insert electrodes into the target tissue. Modification of electrodes will permit endoscopic, laparoscopic or image-guided delivery of electric current to more deep-seated internal tumours Citation[21]. For example, if it were possible to deliver permeabilising electric pulses to intra-abdominal, intra-thoracic or genitourinary tumours, many lesions that would previously have been deemed inoperable would be amenable for treatment with electrochemotherapy. In particular, cancers of the foregut and bladder are often associated with high mortality. The failure of conventional therapies in this setting is often related to features such as advanced stage of disease and poor patient health at the time of presentation. These patients, whose tumours are easily accessible endoscopically, would benefit from a quick, safe, minimally invasive treatment such as electrochemotherapy. As part of a multimodal treatment regime, it is likely that many more primary cancers which are currently inoperable could be treated with curative intent. Because cisplatin is a commonly used chemotherapy agent as part of conventional multidrug regimens, ECT could easily be added to improve the efficacy of many current multimodal programmes. Similarly, ECT can be applied to sensitise recalcitrant tumours to radiation therapy, thus allowing for a more targeted tumouricidal therapy with less collateral injury.
Newer therapeutic agents are being tested for electrical delivery and cytocidal efficacy including interferon alpha in mycosis fungoides, cytolytic derived peptides and cytokines Citation[58].
The electropermeabilisation current on its own does not alter the growth characteristic of experimental tumours indicating cell recovery and continued viability - advantages for gene therapy where therapeutic gene expression requires a duration of normal cell function. Electrical delivery is currently the most efficient way of delivering non-viral gene constructs into tumours and results in tissue transfection efficiency in up to 15% of cells. Thus, while the delivery of oncolytic genes might not be effective, the induced production of immunostimulatory cytokines within the tumour mass has been shown to modulate the local immune system resulting in immune sensitization and in local and systemic disease control for some cancers Citation[59]. Experimentally, neoadjuvant immunogene therapy of primary cancers has been found to be effective against minimal residual disease in metastatic models. This suggests possibilities for clinical application in some currently recalcitrant cancers, such as those of the lung and foregut. A schedule of electroporation-mediated gene delivery followed by electrochemotherapy–mediated tumour ablation several days later has been shown to be feasible and curative in some murine models Citation[60]. In addition, the finding that perilesional cytokine treatment augments the immune response to an involuting tumour following electrochemotherapy, has spurred on current research addressing the most appropriate cytokine, chemokine or combination of the two to fully exploit this phenomenon. Thus combinations of electrogene delivery, electrochemotherapy and perilesional cytokine delivery offer exciting possibilities for local and systemic control of many heretofore incurable cancers.
Conclusion
Electrochemotherapy is an effective drug delivery system appropriate for the treatment of cutaneous or subcutaneous malignant tumours in the setting of recurrent, progressive or inoperable disease refractory to the conventional anticancer therapies. It acts by greatly enhancing the local cytotoxicity of bleomycin, a non-permeant, hydrophilic drug. The treatment can be performed on externally accessible tumours on any part of the body. It is easy and quick to perform, is safe, inexpensive and gives consistently reproducible results. The potential for combining electrochemotherapy with conventional therapies or with appropriate immunotherapies and gene therapies offers many exciting possibilities for the future expansion and development of the clinical utility of this treatment. Electrical delivery of immunogenes into the primary tumour offers the possibility of a neoadjuvant therapy which would induce systemic responses effective against minimal residual disease. Further development of technologies will permit application of this treatment to internal cancers and to earlier stage disease.
Corrigendum: Electrochemotherapy: An emerging cancer treatment
Electrochemotherapy: An emerging cancer treatment, by Mira Sadadcharam, Declan M. Soden, Gerald C.O'Sullivan, International Journal of Hyperthermia, Volume 24, Issue 3, 2008 (pp. 263–273).
The publishers were notified this paper contained errors which were missed at the author correction stage by the authors when this article was first published online ahead of the print publication. We have corrected the errors and republished the paper in this issue (pp. 263–273).
The incorrect first publication is published as an online only supplementary file. We would like to apologize for these publication errors on behalf of the Authors.
Acknowledgements
This work was kindly supported through generous support from the Leslie C. Quick Family and grant awards from the EU (ESOPE- QLK3-2002-02003), Enterprise Ireland and The Irish Cancer Society.
Corrigendum: Electrochemotherapy: An emerging cancer treatment
Electrochemotherapy: An emerging cancer treatment, by Mira Sadadcharam, Declan M. Soden, Gerald C. O'Sullivan, International Journal of Hyperthermia, Volume 24, Issue 3, 2008 (pp. 263–273).
The publishers were notified this paper contained errors which were missed at the author correction stage by the authors when this article was first published online ahead of the print publication. We have corrected the errors and republished the paper in this issue (pp. 263–273).
The incorrect first publication is published as an online only supplementary file. We would like to apologize for these publication errors on behalf of the Authors.
References
- Mir LM, Orlowski S, Belehradek Jr J, Paoletti C. Electrochemotherapy potentiation of antitumour effect of bleomycin by local electric pulses. Eur J Cancer 1991; 27: 68–72
- Belehradek J, Jr, Orlowski S, Poddevin B, Paoletti C. Electrochemotherapy of spontaneous mammary tumours in mice. Eur J Cancer 1991; 27: 73–76
- Heller R, Gilbert R, Jaroszeski MJ. Electrochemotherapy: An emerging drug delivery method for the treatment of cancer. Adv Drug Deliv Rev 1997; 26: 185–197
- Belehradek M, Domenge C, Luboinski B, Orlowski S, Belehradek Jr J, Mir LM. Electrochemotherapy, a new antitumor treatment. First clinical phase I-II trial. Cancer 1993; 72: 3694–3700
- Heller R, Gilbert R, Jaroszeski MJ. Clinical applications of electrochemotherapy. Adv Drug Deliv Rev 1999; 35: 119–129
- Weaver JC. Electroporation theory. Concepts and mechanisms. Methods Mol Biol 1995; 55: 3–28
- Teissie J, Eynard N, Vernhes MC, Benichou A, Ganeva V, Galutzov B, Cabanes PA. Recent biotechnological developments of electropulsation. A prospective review. Bioelectrochemistry 2002; 55: 107–112
- Orlowski S, Belehradek J, Paoletti C, Mir LM. Transient electropermeabilization of cells in culture. Increase of the cytotoxicity of anticancer drugs. Biochem Pharmacol 1988; 37: 4727–4733
- Gehl J, Skovsgaard T, Mir LM. Enhancement of cytotoxicity by electropermeabilization: An improved method for screening drugs. Anticancer Drugs 1998; 9: 319–325
- Mir LM, et al. [Electrochemotherapy, a new antitumor treatment: First clinical trial]. C R Acad Sci III 1991; 313: 613–618
- Effective treatment of cutaneous and subcutaneous malignant tumours by electrochemotherapy. Br J Cancer 1998; 77: 2336–2342
- Heller R, Jaroszeski M, Perrott R, Messina J, Gilbert R. Effective treatment of B16 melanoma by direct delivery of bleomycin using electrochemotherapy. Melanoma Res 1997; 7: 10–18
- Sersa G, Stabuc B, Cemazar M, Miklavcic D, Rudolf Z. Electrochemotherapy with cisplatin: Clinical experience in malignant melanoma patients. Clin Cancer Res 2000; 6: 863–867
- Bloom DC, Goldfarb PM. The role of intratumour therapy with electroporation and bleomycin in the management of advanced squamous cell carcinoma of the head and neck. Eur J Surg Oncol 2005; 31: 1029–1035
- Heller R, Jaroszeski M, Atkin A, Moradpour D, Gilbert R, Wands J, Nicolau C. In vivo gene electroinjection and expression in rat liver. FEBS Lett 1996; 389: 225–228
- Rols MP, Delteil C, Golzio M, Dumond P, Cros S, Teissie J. In vivo electrically mediated protein and gene transfer in murine melanoma. Nat Biotechnol 1998; 16: 168–171
- Gehl J, et al. Electroporation theory and methods perspectives for drug delivery, gene therapy and research. Acta Physiol Scand 2003; 177: 437–447
- Kranjc S, Camazar M, Grosel A, Sentjurc Sersa G. Radiosensitising effect of electrochemotherapy with bleomycin LPB sarcoma cells and tumors in mice. BMC Cancer 2005; 5: 115
- Sersa G, Stabuc B, Camazar M, Miklavcic D, Rudolf Z. Electrochemotherapy with cisplatin: The systemic antitumour effectiveness of cisplatin can be potentiated locally by the application of electric pulses in the treatment of malignant melanoma skin metastases. Melanoma Res 2000; 10: 381–385
- Larkin J, Collins C, Aarons S, Tangney M, Whelan M, O'Reily S, Breathnach O, Soden D, O'Sullivan G. Electrochemotherapy aspects of preclinical development and early clinical experience. Annals Surg 2007; 245: 469–479
- Soden D, Larkin J, Collins C, Tangney M, Aarons S, Piggott J, Morrissey A, Dunne C, O'Sullivan G. Successful application of targeted electrochemotherapy using novel flexible electrodes and low dose bleomycin to solid tumours. Cancer Letters 2005; 232: 300–310
- Chang DC, Reese TS. Changes in membrane structure induced by electroporation as revealed by rapid-freezing electron microscopy. Biophys J 1990; 58: 1–12
- Lopez A, Rols MP, Teissie J. 31P NMR analysis of membrane phospholipid organization in viable, reversibly electropermeabilized Chinese hamster ovary cells. Biochemistry 1988; 27: 1222–1228
- Mir LM, Banoun H, Paoletti C. Introduction of definite amounts of nonpermeant molecules into living cells after electropermeabilization: Direct access to the cytosol. Exp Cell Res 1988; 175: 15–25
- Engstrom PE, Persson BR, Salford LG. Studies of in vivo electropermeabilization by gamma camera measurements of (99 m)Tc-DTPA. Biochim Biophys Acta 1999; 1473: 321–328
- Belehradek Jr J, Orlowski S, Ramirez LH, Pron G, Poddevin B, Mir LM. Electropermeabilization of cells in tissues assessed by the qualitative and quantitative electroloading of bleomycin. Biochim Biophys Acta 1994; 1190: 155–163
- Jaroszeski MJ, Dang V, Pottinger C, Hickey J, Gilbert R, Heller R. Toxicity of anticancer agents mediated by electroporation in vitro. Anticancer Drugs 2000; 11: 201–208
- Kotnik T, Miklavcic D. Analytical description of transmembrane voltage induced by electric fields on spheroidal cells. Biophys J 2000; 79: 670–679
- Hibino M, Shigemori M, Itoh H, Nagayama K, Kinosita Jr K. Membrane conductance of an electroporated cell analyzed by submicrosecond imaging of transmembrane potential. Biophys J 1991; 59: 209–220
- Teissie J, Rols MP. An experimental evaluation of the critical potential difference inducing cell membrane electropermeabilization. Biophys J 1993; 65: 409–413
- Gabriel B, Teissie J. Direct observation in the millisecond time range of fluorescent molecule asymmetrical interaction with the electropermeabilized cell membrane. Biophys J 1997; 73: 2630–2637
- Schwister K, Deuticke B. Formation and properties of aqueous leaks induced in human erythrocytes by electrical breakdown. Biochim Biophys Acta 1985; 816(2)332–348
- Gabriel B, Teissie J. Time courses of mammalian cell electropermeabilization observed by millisecond imaging of membrane property changes during the pulse. Biophys J 1999; 76: 2158–2165
- Mir LM, Orlowski S. Mechanisms of electrochemotherapy. Adv Drug Deliv Rev 1999; 35: 107–118
- Mir LM, et al. Bases and rationale of the electrochemotherapy. Eur J Cancer 2006; 4(Suppl)38–44
- Marty M, Sersa G, Garbay JR, Gehl J, Collins CG, Snoj M, Billard V, Geertsen PF, Larkin JO, Miklavcic D, et al. Electrochemotherapy: An easy highly effective and safe treatment of cutaneous and subcutaneous metastases. Results of ESOPE (European Standard Operating Procedures of Electrochemotherapy) study. Eur J Cancer 2006; 4(Suppl.)3–13
- Ramirez LH, Orlowski S, An D, Bindoula G, Dzodic R, Ardovin P, Bognel C, Belehradek Jr J, Munck JN, Mir LM. Electrochemotherapy on liver tumours in rabbits. Br J Cancer 1998; 77: 2104–2111
- Mir LM. Therapeutic perspectives of in vivo cell electropermeabilization. Bioelectrochemistry 2001; 53: 1–10
- Gehl J, Skovsgaard T, Mir LM. Vascular reactions to in vivo electroporation: Characterization and consequences for drug and gene delivery. Biochim Biophys Acta 2002; 569: 51–58
- Engstrom PE, Persson BR, Bron A, Salford LG. A new antitumour treatment combining radiation and electric pulses. Anticancer Res 2001; 21: 1809–1815
- Sersa G, Cemazar M, Miklavcic D, Chaplin DJ. Tumor blood flow modifying effect of electrochemotherapy with bleomycin. Anticancer Res 1999; 19: 4017–4022
- Mitchell MS. Combining chemotherapy with biological response modifiers in treatment of cancer. J Natl Cancer Inst 1988; 80: 1445–1450
- Mir LM, Tounekti O, Orlowski S. Bleomycin: Revival of an old drug. Gen Pharmacol 1996; 27: 745–748
- Tounekti O, Belehradek PG, Jr, et al. Bleomycin, an apoptosis-mimetic drug that induces two types of cell death depending on the number of molecules internalized. Cancer Res 1993; 53: 5462–5469
- Pron G, Mahrour N, Orlowski S, Tounekti O, Poddevin B, Belehradek J, Mir LM. Internalisation of the bleomycin molecules responsible for bleomycin toxicity: A receptor-mediated endocytosis mechanism. Biochem Pharmacol 1999; 57: 45–56
- Poddevin B, Belehradek OS, Jr, et al. Very high cytotoxicity of bleomycin introduced into the cytosol of cells in culture. Biochem Pharmacol 1991; 42(Suppl)S67–S75
- Tounekti OKA, Foray N, et al. The ratio of single- to double-stranded DNA breaks and their absolute values determine cell death pathway. Br J Cancer 2001; 84: 1272–1279
- Okino M, Tomie H, Kanesada H, Marumoto M, Esata K, Suzuki H. Optimal electric conditions in electrical impulse chemotherapy. Jpn J Cancer Res 1992; 83: 1095–1101
- Heller R MJ, Leo-Messina J, Perrott R, Van Voorhis N, Reintgen D. Treatment of B16 melanoma with the combination of electroporation and chemotherapy. Bioelectrochemistry 1995; 36: 83–87
- Gately DP, Howell SB. Cellular accumulation of the anticancer agent cisplatin: A review. Br J Cancer 1993; 67: 1171–1176
- Melvik JE EOP, Gordon PB, Seglen PO. Increase in cis-dichloroammineplatinum (II) cytotoxicity upon reversible electropermeabilization of the plasma membrane in cultured human NHIK 3025 cells. Eur J Cancer 1986; 22: 1523–1530
- Sersa G MC, Miklavcic D. Antitumor effectiveness of electrochemotherapy with cis-diamminedichloroplatinum (II) in mice. Cancer Res 1995; 55: 3450–3455
- Mir LM, Gehl J, Sersa G, Sersa G, Collins CE, Garbay JR, Billard V, Geertsen PF, Rudolf Z, O'Sullivan GC, et al. Standard operating procedures for electrochemotherapy: Instructions for the use of bleomycin or cisplatin administered either systemically or locally and electric pulses delivered by the Cliniporator by means of invasive or non-invasive electrodes. Eur J Cancer 2006; 4: 14–25
- Domenge C, Orlowski S, Luboinski M, De Baere T, Schwaab G, Belehradek Jr J, Mir LM. Antitumor electrochemotherapy: New advances in the clinical protocol. Cancer 1996; 77: 956–963
- Cemazar M, Milacic R, Miklavcic D, Dolzan V, Sersa G. Intratumoral cisplatin administration in electrochemotherapy: Antitumor effectiveness, sequence dependence and platinum content. Anticancer Drugs 1998; 9: 525–530
- Marty M, Sersa G, Garbay JR, Gehl J, Collins GC, Snoj M, Billard V, Geertsen PF, Larkin JO, Miklavcic D, et al. Electrochemotherapy–An easy, highly effective and safe treatment of cutaneous and subcutaneous meastases: Results of ESOPE (European Standard Operating Procedures of Electrochemotherapy) study. Eur J Cancer 2006; 4((Suppl.))3–13
- Whelan M, Larkin JO, Collins GC, Cashman J, Breathnach O, Soden DM, O'Sullivan GC. Effective treatment of an extensive recurrent breast cancer which was refractory to multimodal therapy by multiple applications of electrochemotherapy. Eur J Cancer 2006; 4(Suppl.)32–34
- Peycheva E, Daskalov I, Tsoneva I. Electrochemotherapy of mycosis fungoides by interferon alpha. Bioelectrochemistry 2007; 70: 283–286
- Collins C, Tangney M, Larkin JO, Casey G, Whelan MC, Cashman J, Murphy J, Soden D, Vejda S, McKenna S, et al. Local gene therapy of solid tumors with GM-CSF and B7-1 eradicates both treated and distal tumors. Cancer Gene Therapy 2006; 13: 1061–1071
- Heller L, Joroszeski MJ, Coppola D, Pottinger C, Gilbert R, Heller R. Electrically mediated plasmid DNA delivery to hepatocellular carcinomas in vivo. Gene Ther 2000; 7: 826–829