Abstract
The effects of hyperthermia on natural killer (NK) cell cytotoxicity against tumor cell targets are not yet fully understood. A more complete understanding of these effects could be important for maximizing the clinical benefits obtained by using hyperthermia for cancer therapy. Here, we summarize results in the literature regarding the effects of elevated temperatures on NK cells and our own recent data on the effects of fever-range temperatures. At treatment temperatures above 40°C, (which is above the physiological body temperatures normally achieved during fever or exercise), both enhancing and inhibitory effects on cytotoxic activity of NK cells against tumor cells have been reported. Our own results have shown that fever-range thermal stress (using a temperature of 39.5°C) enhances human NK cell cytotoxicity against tumor target cells. This effect requires function of the NKG2D receptor of NK cells, and is maximal when both NK and tumor cell targets are heated. Reported heat sensitive cellular targets affected by hyperthermia on tumor cells include heat shock proteins, MICA and MHC Class I. In NK cells, plasma membrane reorganization may occur after mild heat stress. We conclude this review by listing several unresolved questions that should be addressed for a more complete understanding of the molecular mechanisms which underlie the effects of thermal stress on the function of NK cells. Altogether, the available data indicate a strong potential for heat-induced enhancement of NK cell activity in mediating, at least in part, the improved clinical responses seen when hyperthermia is used in combination with other therapies.
Introduction
Reports that link the occurrence of fevers with improved recovery from cancer have been cited in the literature for well over a century Citation[1], and various protocols to artificially induce hyperthermia have also been investigated as tools for treating cancer patients in combination with other therapies Citation[2]. Recent clinical trials utilizing the addition of heat to the tumor site during treatment with radiation continue to provide very encouraging data regarding overall clinical benefit (i.e. complete response rate, overall and relapse free survival) of this combination Citation[3–7], Citation[8]). These studies have renewed enthusiasm for combining hyperthermia with radiation, and recent studies also confirm the strong clinical benefit of combining hyperthermia with chemotherapy Citation[9]. However, the molecular or cellular events contributing to overall survival benefits seen by adding hyperthermia to radiation or chemotherapy are not entirely clear. While the historical origin of the use of hyperthermia has been linked to the potential anti-cancer benefits observed when patients developed high fevers, modern studies have largely focused on two non-exclusive explanations of why hyperthermia enhances efficacy of radiation or chemotherapy: (1) the direct cytotoxic effects of high temperatures observed in tumor cells and intra-tumoral vascular structures, which could be particularly feasible during local hyperthermia treatments Citation[4], Citation[10]; or (2) the indirect effects of elevated temperatures on blood flow, or on other aspects of the tumor microenvironment. Most literature on the indirect effects of hyperthermia center on tumor re-oxygenation which could result in enhanced efficacy of radiation treatment Citation[11–14] and reviewed in Citation[4], Citation[10]. However, an emerging set of literature Citation[15] identifies the potential of hyperthermia to serve as an adjuvant to the immune system, helping to provoke stronger anti-tumor responses. Hyperthermia, particularly in the mild, physiologically relevant temperature range, may trigger long-conserved, heat sensitive molecules in cells of the immune system that have evolved to respond to the thermal element of fever, which occurs as a host inflammatory response to viral or bacterial challenges to the immune system. Recent evaluations Citation[16–20] strongly support thermal enhancement of anti-tumor immunity as at least part of the basis for improved long-term survival of patients given hyperthermia since intra-tumoral temperatures high enough to cause direct thermal killing of tumor cells is not as commonly achieved in the clinical setting as was previously believed Citation[4]. However, even if non-physiologically high temperatures are used in the clinic, delivered locally at the site of the tumor, causing rapid tumor or vascular endothelial cell death, other regions of the body will eventually be warmed by dissipated heat; thus, even under tumor ablative thermal conditions, there is still considerable potential for enhancement of the immune system by milder hyperthermia in the surrounding tissue.
In terms of identifying which immune effector cells could play critical roles in mediating the improved survival seen when hyperthermia is combined with cancer treatment, NK cells have emerged as one likely possibility. NK cells have already been recognized as being able to recognize and kill tumor cells treated at heat shock temperatures Citation[21] through recognition of heat shock proteins on the surface of the tumor cell. Major questions which need to be addressed include whether there is an optimal temperature range or duration of heating for maximal enhancement of NK cell cytotoxicity, or whether various forms of heating (e.g. ultrasound, microwave or infrared) differentially affect NK cell function. In order to gain some understanding of these questions, it is important to review previous literature on cellular and molecular effects of increased temperature on NK cells, with a particular focus on studies that address anti-tumor activity. We summarize studies on the effects of elevated temperatures on NK cell distribution and number, tumor infiltration and cytotoxic function, and on temperature dependent sensitivity of tumor cells to NK cell killing. We attempt to separate enhancing and inhibitory effects, and to correlate these effects with the temperatures used for the study (e.g. heat shock conditions versus mild, physiologically relevant elevations in temperature) (see ). We conclude this review with a set of questions which should be addressed in order to fully understand the role of NK cells in mediating the positive benefits of clinical hyperthermia.
Table I. Chronological summary of studies investigating the effects of hyperthermia on NK cell function.
Key role of NK cells in anti-tumor immunity
NK cells are a subset of the innate immune system and play a major role in the control of tumor cells via direct cytotoxicity against target cells exhibiting altered MHC Class I structure or levels on their cell surface Citation[22], Citation[23]. Unlike adaptive immune responses, NK cells do not require prior sensitization with an antigen, but when sensitized to haptens, recent studies have shown that NK cells have potential for generating memory responses Citation[24]. An NK cell response precedes the adaptive anti-tumor immune response and is critical for downstream tumor-specific T cell memory responses mediated by CD27-CD70 interaction Citation[25].
The earliest phase of NK cell activation, termed the ‘non-specific phase’, precedes the triggering of any receptors on the surface of NK cells. Pro-inflammatory cytokines in the microenvironment have been shown to regulate the non-specific phase of NK cell activation Citation[26]. TNF-α is known to recruit NK cells to the inflammatory sites Citation[27], Citation[28], where pro-inflammatory cytokines like IL-2, IL-7, IL-12, IL-15 and Type I IFNs Citation[26], Citation[29–32] are known to rapidly activate them. Importantly, some of these cytokines that activate NK cells (e.g. TNF-α, IFN-α/β) are also ‘pyrogenic’ in that they stimulate an increase in body temperature (fever), which occurs during infection and inflammation Citation[33], Citation[34]. Although there is a temporal link between NK cell activation and the pyrogenicity of these cytokines, whether there is a specific role for increased temperature in regulation of NK cell function is not yet fully understood.
Following the cytokine-associated ‘non-specific’ activation phase, induction of cytotoxicity for NK cells is further regulated by a complex mechanism of balance between activating, e.g. NKG2D Citation[35–37], natural cytotoxicity receptors (NCR) (i.e. NKp46 Citation[38], NKp30 Citation[39] and NKp44 Citation[40], Citation[41], and several inhibitory receptors (i.e. KIR and CD94-NKG2 heterodimers Citation[42], Citation[43]). The magnitude of NK cell activation is a function of altering the balance in the signaling strength between activating and inhibitory receptors. If the balance shifts towards activating receptors, NK cells respond with secretion of effector molecules leading to the lysis of their targets. This fine tune of activation causes NK cells to play a major role in immunosurveillance of tumors Citation[44]. During the formation of an immunological synapse between an NK cell and its target cell, receptors on NK cells reorganize into clusters that are associated with the lipid raft fraction of the NK cell plasma membrane Citation[45]. Intriguing experimental evidence (summarized below) suggests that such receptor-associated activity of NK cells may indeed be thermally sensitive. However, further characterization of the mechanisms by which physiologically relevant temperatures affect NK cell-mediated cytotoxicity is essential.
NK cells in clinical hyperthermia studies
Identification of NK cells as critical effector cells in immune responses mediated by clinical use of local and systemic hyperthermia resulted in further interest for the study of this cell type in detail. Clinical studies reviewed below have investigated effects of hyperthermia on several parameters of NK cell function in patients, including NK cell cytotoxicity and changes in peripheral NK cell numbers (summarized in and ).
Figure 1. Summary of preclinical and clinical studies that have investigated NK cell responses to hyperthermia. NK cells can be activated through a series of activating and inhibitory receptors, and the balance of these signals decides the fate of NK cell cytotoxic activity. The reported effects of hyperthermia on NK cell cytotoxicity, distribution in the periphery and cell number and infiltration into tumor beds are summarized in this schematic.
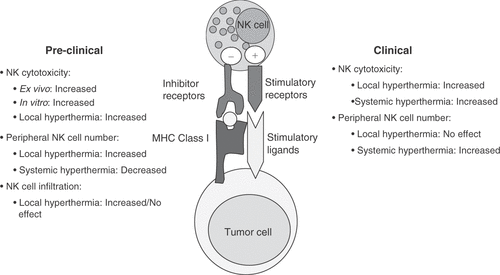
Effects on NK cell cytotoxic activity
The role of hyperthermia on NK cell function was first investigated over 25 years ago, in a study by Zanker et al., which reported an enhancement of NK cell cytotoxicity with whole body hyperthermia at 41.8°C in a patient with Ewing's sarcoma Citation[46]. In support of this observation, isolated limb perfusion in patients with malignant melanomas (40.5–41.8°C for 1 hour) resulted in enhanced NK cytotoxicity of peripheral blood cells during heating Citation[47]. Furthermore, treatment of prostate carcinoma patients with local transrectal microwave hyperthermia (45°C, 30 min, six treatments) resulted in a significant increase of their NK cytotoxic activity against K562 target cells in vitro Citation[48]. Another study found that cancer patients who received whole body hyperthermia (40.5°C, 75 min) and IFN-γ therapy also demonstrated increased NK cell cytotoxicity, but this increase was not statistically significant when compared to the group who received IFN-γ alone Citation[49]. Application of local hyperthermia to healthy human subjects had no effect on the cytotoxic activity of peripheral blood-derived NK cells in this study. When mild whole body hyperthermia at 38°C core temperature was applied to healthy volunteers, an enhanced NK cell activity was again observed Citation[50]. In summary, although the extent of the effect varies, clinical studies appear to generally reveal an enhancement of NK mediated cytotoxic activity upon hyperthermia treatment of either cancer patients or healthy subjects.
Effects on peripheral NK cell numbers or distribution
Increased NK cell numbers in peripheral blood can concur with enhanced cytotoxic activity measurements, and thus support the clinical outcome of hyperthermia against tumors, thus here we summarize the studies reporting NK cell counts in patients after clinical hyperthermia. Our group observed that NK cell numbers in peripheral blood of cancer patients were increased immediately after a 6-hour whole body hyperthermia treatment of 39.5°–40°C Citation[51]. Moreover, when cancer patients with various metastatic tumors were treated with whole body hyperthermia of 41.8°–42.2°C for 1 hour, absolute numbers and percentage of NK cells in the peripheral blood increased significantly starting from 2 hours after hyperthermia Citation[52]. Most recently, similar observations regarding increased numbers of NK cells circulating in peripheral blood of patients with various tumors were made by two groups using whole body hyperthermia protocols of 41°–41.8°C for 1 hour Citation[53], or 39.4°C for 30 min Citation[54]. In healthy human subjects, mild whole body hyperthermia of 38°C also increased peripheral blood NK cell counts, while local hyperthermia had no effect Citation[50].
In conclusion, these clinical studies propose a role for hyperthermia to affect the distribution of NK cells and their cytolytic function, which supports possible use of hyperthermia as an adjuvant for cancer therapies.
NK cells in pre-clinical hyperthermia studies
Experimental application of elevated temperatures in vitro, using either human or animal cells, or in vivo, using animal models, have been performed by some investigators to better define the effects of hyperthermia on NK cell function, as well as on the tumor target cells. Here we summarize many of these studies regarding the application of hyperthermia in preclinical studies, where analyses of NK cytotoxicity, peripheral NK cell numbers, and NK cell infiltration into tumor beds were performed.
Effects on NK cell cytotoxicity
In the first study to describe the effect of hyperthermia on tumor cells, malignant thyroid cells from patients were found to be more sensitive to killing by IL-2 activated NK cells when they were heated ex vivo to heat shock temperatures (44°C for 20 min) and used for cytotoxicity assays Citation[55]. Interestingly, this observation was thought to be dependent on de novo protein synthesis by tumor cells. In recent studies, heat shock temperatures (42°C, 1 hr) on tumor cells significantly enhanced NK cell killing of KM12 colon carcinoma cells Citation[56], but not Colo205 cells Citation[57]. In our studies, cytotoxicity of purified human peripheral blood NK cells against Colo205 human colon adenocarcinoma cells was found to be significantly enhanced at fever range temperatures (39.5°C, 6 hours) Citation[57]. More specifically, the cytotoxic activity of human peripheral blood NK cells, but not NK cell lines or IL-2 activated NK cells, was enhanced when both NK effectors and tumor target cells were exposed to fever-range temperatures. Thermal enhancement of cytotoxicity was not observed when autologous PBMC were used as targets for NK cells, indicating that thermal induction of NK cytolytic activity was specific for the Colo205 cells Citation[57]. In an earlier study from our group, similar fever-range temperatures applied via whole body hyperthermia enhanced NK cell killing in a manner that was responsible for the growth delay of human breast tumor xenografts in SCID mice in vivo Citation[58]. Splenocytes taken from B16.F10 melanoma bearing C57BL/6, mice treated with local hyperthermia (42°C, 10 min) also displayed increased natural cytotoxicity against YAC-1 cells in vitro Citation[59].
In contradiction to these studies describing thermal enhancement of NK cell cytotoxicity, there have also been studies which indicate that hyperthermia can inhibit NK cell cytotoxicity. Azocar Citation[60] and Nurmi et al. Citation[61] observed a decrease in human NK cell cytotoxic activity at 40°C in vitro, which was also supported by observations of Dinarello et al., using 39°C for 18 hours Citation[62]. In a mouse model of hyperthermia, long-term (up to 16 days), continuous mild whole body hyperthermia of 38.5°C in C3H/HeNCrj mice suppressed the natural cytotoxic activity of splenocytes against YAC-1 cells Citation[63].
In conclusion, there is conflicting information regarding the effects of hyperthermia on human and mouse models for NK cell cytotoxicity, which in part, may relate to duration of heating at various temperatures. A consensus on thermal regulation of NK cell anti-tumor responses may require consideration of additional parameters such as NK cell numbers in the periphery or infiltration into tumor beds and the tumor model used, and also duration of heating at various temperatures.
Effects on peripheral NK cell numbers or tissue distribution
Similar to the cytotoxicity studies discussed above, there are also conflicting reports on the effects of hyperthermia on the numbers of NK cells in tissues and peripheral blood. These differences could be due in part to temperatures used, as well as duration of heating. Local hyperthermia for 42°C for 10 min on B16.F10 melanoma-bearing C57BL/6, mice was found to increase Citation[59], whereas long-term, continuous mild hyperthermia (38.5°C) was found to decrease the number of NK cells in the spleen Citation[63]. In a rat model of peritonitis, hyperthermia (42°C, 15 min) either did not change Citation[64] or decreased (with hyperthermic preconditioning at 40°C for 4 hours) Citation[65] the percentage of NK cells in peripheral blood.
It is important to recognize that differences in the distribution of NK cells in the periphery after hyperthermia may reflect their altered trafficking to tumors or other sites of inflammation in response to temperature changes. Thus, since NK cells must make physical contact with tumor cells in order to recognize their targets, we next examined the literature regarding the effects of hyperthermia on the presence of NK cells within the tumor microenvironment.
Effects on tumor infiltration of NK cells
In mouse models, an increased number of NK cells and other leukocytes were found to infiltrate the tumor bed of B16 melanomas in C57BL/6, mice given local hyperthermia at 43°C for 15 min Citation[66–68] or 42°C for 30 min Citation[69]. However, a recently published study found no significant change in NK cell infiltration between control and local hyperthermia (42°C for 7 min) groups using the same melanoma model Citation[59]. An increased number of NK cells were found to infiltrate the tumor bed of breast xenografts in SCID mice treated with whole body hyperthermia (39.8°C, 6–8 hours) Citation[58]. Thus, in mouse models that investigated the changes of NK cell infiltration, there is some support for the possibility of thermal enhancement of NK cell infiltration at the tumor site, however, much more work should be done on this question.
Potential mechanisms for thermal regulation of NK cell function
In the clinical setting, it would be expected that NK cells in the heated field and their tumor targets would each experience hyperthermia conditions. To understand the effects of hyperthermia on NK cells and their tumor cell targets observed in clinical and preclinical studies, several molecular mechanisms have been proposed and investigated. Here we summarize many of these mechanisms, focusing first on thermally induced changes that occur in NK cells and second on thermally induced molecular changes in tumor cells (which may make them more sensitive to NK cell killing).
Effects on NK cells
The nature of heat induced changes in NK cells, resulting in altered cytotoxic function is still undefined. However, it is at least thought to be independent of MHC Class I/peptide complexes Citation[70] as reviewed by Milani et al. Citation[71]. Early on, Fleishmann et al. showed that elevated temperatures (39.4°C) greatly enhanced anti-proliferative function of IFN-γ on tumor cells, which is one of the major effector molecules secreted by NK cells Citation[72]. On NK cells, clustering of activating receptor NKG2D occurs at the site of target cell contact during the formation of conjugates between NK cells and target cells during IL-2 enhanced NK cell cytotoxicity Citation[45]. Thus, we investigated the effect of mild thermal stress on NKG2D localization on the NK cell surface. NKG2D surface expression was not changed but NKG2D clustering on NK cell surface increased significantly, albeit transiently with fever-range thermal stress. Cross-linking the NKG2D receptor with anti-NKG2D antibodies or IL-2 activation of NK cells resulted in a similar clustering Citation[57]. Disruption of lipid rafts, which have been shown to play a key role in the formation of NK cell synapse, caused a loss of thermally enhanced NK cell cytotoxicity Citation[57]. It is known that NKG2D ligand MICA also clusters at the NK cell synapse in lipid rafts Citation[73], which has direct consequences in its recognition by NK cells. It has been recently shown that, NKG2D localizes in both lipid raft and non-raft fractions on the NK cell line NKL, and its recruitment to raft fractions increase with NKG2D cross-linking Citation[74]. However it is also important to note here that the NKL cell line was found to be insensitive to hyperthermia Citation[57], possibly due to the activated state of NKL cells during culture with IL-2, which is required for their survival.
NK cells in an activated state (i.e. cultured in presence of IL-2) are widely used for NK cell functional assays. NK cell activation state in peripheral blood (‘resting’) could be fundamentally different than their state in culture conditions with cytokines such as IL-2 in vitro (‘activated’). Sensitivity to thermal stress could be affected by the activation state of NK cells as the cytotoxicity of freshly isolated NK cells is enhanced by incubation at 39.5°C, while IL-2 activated NK cells fail to demonstrate a thermally enhanced cytotoxicity Citation[57]. There might be a correlation between clustering of activating receptor NKG2D and the activation state of NK cells, as IL-2 activated NK cells show enhanced NKG2D clustering, which could not be further enhanced with hyperthermia. The non-responsiveness of IL-2 activated NK cells to elevated temperatures has been previously observed by others. Cytotoxicity of IL-2 activated NK cells heated at 40°C for 1 hr and then cultured for 7 days was not enhanced against either NK sensitive K562 or NK resistant Raji cell lines Citation[75]. Higher thermotolerance of poly I:C activated NK cells compared to untreated, resting cells was observed at temperatures above 40°C Citation[76], therefore NK cells might have a selective sensitivity to thermal stress depending on their activation status.
In conclusion, thermal stress enhances clustering of NKG2D in resting NK cells, (similar to the clustering observed with crosslinking of NKG2D with antibodies) and disruption of lipid rafts prevents the thermal enhancement of NK cell cytotoxicity. These results imply that NKG2D clustering upon thermal stress in the NK cell plasma membrane has functional consequences in thermal enhancement of NK cell cytotoxicity.
Effects on tumor cells and other immune cells
Thermal stress triggers a variety of physiological changes in tumor cells. Studies investigating effects of hyperthermia on tumor cells concentrate on three molecules with potential to affect NK cell functions.
MHC Class I molecules
MHC Class I molecules have fundamental roles in recognition of target cells by NK cells. Down-regulation of these molecules on the cell surface was hypothesized to result in their recognition by NK cells, as described in the ‘missing-self’ hypothesis Citation[22], Citation[77]. Hyperthermia at 45°C for 30 minutes has been shown to down-regulate MHC class I molecules on melanoma cells Citation[78], which would directly enhance NK cell target recognition and cytotoxic activity. In contrast, MHC Class I expression was found to be unchanged on K562 cells cultured at 41.8°C Citation[79], or on Colo205, HCT116, HT29 cells cultured at fever-range temperatures (39.5 C for 6 hours) Citation[57]. However in rat glioma cells, cell surface presentation of class I antigens were increased with hyperthermia (43°C 1 hr), leading to suppressed tumor growth in vivo Citation[80]. Thus, although MHC Class I might be altered with hyperthermia in some circumstances, it appears that possible down-regulation may not be a predominant mediator of enhanced NK cytotoxicity seen at temperatures normally achieved during clinical hyperthermia treatment.
Heat shock proteins
An important change induced by heat shock on tumor cells is the synthesis of heat-inducible proteins, collectively known as heat shock proteins (HSPs). These proteins appear to play key roles in the activation of NK cells, and might mediate the NK cell response to thermal stress. NK cell recognition of heat shock inducible immunologic determinants on tumors was first described by Multhoff et al. Citation[21]. In this study the induction of HSP72, a member of the HSP70 family, was observed on the surface of Ewing's sarcoma and osteosarcoma cells with heat shock. Heat shock also up-regulated HSP70 expression on osteosarcoma and chondrosarcoma cells (42.5°C, 90 minutes) Citation[81], and on human ocular melanoma cells (45°C, 30 minutes) Citation[78], resulting in increased susceptibility to NK cells. In addition, a more moderate hyperthermia (41.8°C, 3 hours) was found to enhance cytotoxicity of IL-2 activated NK cells through induction of HSP70 expression on the surface of human renal cell carcinoma ACHN cells Citation[82]. HSP70 has also been found to be induced by mild thermal stress (below 40°C) on CT26 tumor cells Citation[83] and several tissues of BALB/c mice Citation[51], and with heat shock on human melanoma cell lines Citation[78]. An HSP70-derived peptide, as well as HSP70 positive tumor exosomes, has been shown to induce chemotaxis and cytotoxic activity of purified NK cells Citation[84], Citation[85]. Furthermore, an increase in HSP70 levels of B16 tumors after whole body hyperthermia treatment (40°C for 2 hours daily for 18 days) correlated with a significant decrease in tumor weight Citation[86]. Similarly, melanoma cell lines transfected to express HSP25 were shown to be more susceptible to killing from IL-2 activated NK cells, despite unaltered surface MHC class I levels Citation[87]. Also, HSP70 transfected human colon adenocarcinoma cells show an enhanced sensitivity to human NK cells that can be blocked with anti-HSP70 antibodies Citation[88]. While CD94 has been proposed to be a receptor for HSP70 on NK cells Citation[89] and potentially mediate the ability of HSP70 to stimulate both the proliferation and activity of NK cells, it has also been proposed that it is the binding of HSP70 to granzyme B that renders tumor cells more sensitive to the cytolytic attack of NK cells Citation[88].
Interestingly, when B16 melanoma cells were heated at 43°C for 30 minutes in vitro, the high levels of HSP70 observed in the viable cells correlated with enhanced maturation of dendritic cells that were pulsed with these tumor cells Citation[90]. In vivo, B16 bearing mice treated with hyperthermia (43°C, 30 min) and injected intratumorally with immature dendritic cells displayed enhanced survival Citation[90]. When in vitro NK cell activity was measured in these surviving mice, NK cell activity was found higher in this group than in the naïve mice. The role of HSP70 activation in antigen presentation and the resulting immune stimulation (including NK cell stimulation) is reviewed by Milani and Multhoff Citation[91], Citation[92].
The effects of hyperthermia were also investigated on HSP90, another member of the heat shock protein family, whose role as a molecular chaperone under heat shock is well established. Indirect effects of HSP up-regulation on immune cells were found to regulate NK cell activation. For example, HSP90 was shown to be up-regulated in bone marrow derived dendritic cells via treatment with fever-range thermal stress. Fever range thermal stress was found to activate these cells to secrete IFN-γ, a pro-inflammatory cytokine that sustains NK cell activation as well as causing dendritic cells to mature Citation[83].
Another HSP capable of triggering NK cell responses is the endoplasmic reticulum resident gp96/grp94 (HSP96) Citation[93]. Although heat stress does not up-regulate gp96 levels significantly Citation[94], this member of the HSP90 heat shock family was indicated for its role in inducing NK cell responses. An enhanced NK activity in peripheral blood mononuclear cells of colorectal cancer patients was observed (18 out of 26 patients) after their vaccination with autologous tumor-derived gp96. This activity is accompanied by an expansion in the CD3-CD56+ NK cell population, up-regulation of activating receptors NKG2D and NKp46, enhanced IFN-γ secretion and cytotoxicity against K562 targets Citation[95]. Dendritic cells pulsed with tumor-derived gp96 show tumor growth delay in C57BL/6 mice carrying an OVA-transfected lung tumor cell line LLC, and this delay is lost with the depletion of NK cells Citation[96].
Overall, it is obvious through these studies that HSP induction on tumor cells and other immune cells by hyperthermia has significant potential to affect NK cell functions.
MHC Class I like molecule A (MICA)
Another candidate molecule on tumor cells that might play a mechanistic role in the thermal enhancement of NK cell activity is the stress inducible, non-classical MHC Class I ligand A (MICA) which is recognized by the NKG2D activating receptor on NK cells Citation[97]. The gene for MICA contains heat shock response element-like sequences in its promoter region and its expression has been shown to be enhanced with heat shock (42°C) in HeLa cells Citation[98], Citation[99] and HCT116 cells Citation[100]. Our group has found that a milder fever-range thermal stress (39.5°C, 6 hr) is capable of inducing up-regulation of MICA expression in human colon adenocarcinoma cell lines Citation[57]. MICA is encoded within the MHC cluster, and transcription factors Hsf1 and Sp1 have been suggested to regulate its transcription during heat shock Citation[100]. Rodriguez-Rodero et al. also found that the MICA promoter is not polymorphic and mutation in the Hsf1 binding site, together with inhibitors of Sp1, can cause significant loss of activity in the MICA promoter in HeLa and Caco-2 cells Citation[101].
Altogether, MHC Class I molecules, HSPs and MICA are three current candidates involved in hyperthermia-induced changes on tumor cells that might result in enhanced NK cell recognition and killing of tumor targets (see ).
Figure 2. Hypothetical model for effects of hyperthermia enhanced cytotoxicity. (1) Hyperthermia acts on both NK cells and tumor targets independently. (2) Thermal enhancement of the membrane clustering of the NKG2D stimulatory receptors on the NK cell surface membrane has been reported on NK cells. (3) Increased expression of stimulatory ligands on tumor cells (e.g. MICA, Hsp70) has been found with hyperthermia, and this increase might be accompanied by (4) a decrease of MHC class I expression on tumor cell surface, which could further activate NK cells. (5) Tumor derived Hsp70 has also been suggested to be presented to NK cells indirectly by antigen presenting cells. (6) These changes result in enhanced killing of tumor targets by NK cells.
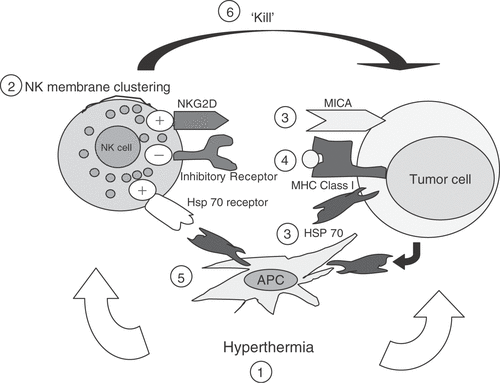
Together, these findings suggest that the overall effect of hyperthermia on NK cell function depends on alterations in both the effector and target cells, as we have previously proposed Citation[57].
Unresolved questions concerning the effects of hyperthermia on NK cells
The studies summarized above point out changes in NK cell function and distribution in various models in response to hyperthermia. While these results have significantly contributed to the understanding of the potential for thermal enhancement of NK cells during clinical hyperthermia, the available information is still insufficient (and often conflicting).
Several studies reviewed here support an increase in NK cell infiltration into tumors during hyperthermia. On the other hand, results in pre-clinical in vivo and in vitro models of hyperthermia measuring NK cell cytotoxicity and distribution are conflicting. It is important to note, though, that these studies use the same model to investigate the NK cell infiltration in several published studies and fail to distinguish NK cells from NKT cells in their models. Therefore, a more complete characterization of infiltrating cells into the tumor bed constitutes an important future goal. Moreover, it is still not clear how heat triggers a change in NK cell trafficking patterns or tumor infiltration that enhances their numbers within the tumor microenvironment.
Apart from the recruitment of NK cells to the tumors, a critical parameter for NK cell mediated control of tumor is the recognition and formation of an immunological synapse between NK cells and their targets (NKIS) Citation[102]. Although several aspects of NKIS have been previously examined with regards to its role in NK signal transduction for cytotoxicity Citation[103] and the intercellular exchange of molecules between NK cells and targets Citation[104], Citation[105], the role of hyperthermia in NKIS formation has not been investigated. As NK mediated tumor killing could potentially be affected by the cells on both sides of the NKIS (i.e. NK cells or tumor targets), hyperthermia could also be affecting cell surface molecules on both sides. Furthermore, it has been previously proposed that an activating NK immune synapse, resulting in NK cell activation, is different from an inhibitory synapse with regard to the recruitment of distinct proteins to the synapse site Citation[106]. Thus, one possible mechanism for enhanced NK cell function with hyperthermia is the increase of stimulatory receptor levels recruited to the activating immunological synapse site on NK cells. We have previously shown that total surface NKG2D levels on peripheral blood NK cells do not change with hyperthermia Citation[57]. However, it is yet to be determined whether other stimulatory receptors such as CD16, CD56, NKp30, NKp44, NKp46, CD94/NKG2C, DNAM-1, KIR2DS, 2B4, NKp80, NKR-P1C are affected during hyperthermia treatments of NK cells. Conversely, the corresponding ligands to these activating receptors, such as HSPs, MICB, ULBP family members, pp65, HLA-C, CD48, AICL, and Nectin-2, as well as MICA (which has already been shown to localize into the NK cell synapse site on tumor cells Citation[73]), need to be investigated with regard to their expression levels and/or localization on the tumor/target cell membrane with hyperthermia. Furthermore, as the cytotoxic function of NK cells is a balance between their activating and inhibitory receptors, a decrease on the expression of inhibitory receptors or their corresponding ligands on tumor cells could result in enhanced NK cell function with hyperthermia. We investigated the surface expression of the major inhibitory receptor for NK cells (HLA-A,B,C) and found that their expression does not change with fever-range thermal stress Citation[57]. However, numerous NK cell inhibitory receptor members such as KIR2DL and the KIR3DL family, the CD158/KIR2DL5 family, p70/AIRM (Siglec 7), Siglec 9, LAIR 1, NKG2A, and the Ly49 family as well as their ligands have not been investigated at all following hyperthermia.
Changes in effector molecule function of NK cells such as IFNγ (as mentioned above), TNF-α, GM-CSF, perforin, and granzymes might be another way of affecting NK cell functions by hyperthermia. As NK cells depend on these effector molecules for target cell cytotoxicity, as well as recruiting other immune cells to the tumor site, investigation of these effector molecules could answer fundamental questions about hyperthermia effects on NK cell responses.
Activation of stimulatory signaling pathways of NK cells by various kinases or inhibition of these signaling pathways by phosphatases could play a regulatory role in transmitting the effects of hyperthermia into a functional outcome for NK cells, which needs further studies to address. Recent publications suggest a transfer of activating receptor ligands such as MICA Citation[104] and MICB Citation[105] between interacting tumor and NK cells with functional consequences in cytotoxicity. The rate of this exchange with thermal stress has never been investigated.
Finally, MICA as a soluble ligand for the activating receptor NKG2D was found to be secreted through metalloproteinase activity in patients with tumors Citation[107–109]. Yet it is unknown whether other stimulatory or inhibitory ligands could be solubilized and how hyperthermia might affect this mechanism in cancer patients. However, our group has already found that soluble MICA levels were not affected with hyperthermia in Colo205 cells Citation[57].
Conclusions
In this review we have summarized the literature regarding the effects of hyperthermia on NK cytotoxic function, their number and distribution in the peripheral blood and spleen, as well as tumor infiltration. We have discussed possible molecular targets of heat on the anti-tumor activity of NK cells.
Potential mechanisms by which hyperthermia affects NK cell function include the involvement of MHC class I, HSPs (especially HSP70) and MICA on tumor cells, and changes in surface NKG2D and lipid raft clustering on NK cells. These mechanisms are summarized in . Several other mechanisms proposed in this review such as formation of NK cell synapse, changes in the levels of activating receptors and their corresponding ligands, effector molecules and downstream activation signaling that might play a role on the effects of hyperthermia on NK function are currently under investigation.
Clinical studies agree on enhanced cytotoxicity as well as increased NK cell numbers in the tissues of cancer patients and healthy subjects with hyperthermia. Several clinical and preclinical results of hyperthermia are summarized in and . Collectively, we believe that the data generally support the idea that hyperthermia can serve as an adjuvant, enhancing NK cell mediated tumor killing. Furthermore, we have recently demonstrated that the ability of hyperthermia to act as a adjuvant is not limited to cancer immunotherapy, but is also effective in regulating immune cell function in the setting of autoimmune disease. Recent studies have demonstrated that administration of weekly fever-range whole body hyperthermia in a non-obese diabetic mouse model can significantly prevent disease progression, and this effect is associated with an increase in NK cell cytotoxicity which may be important for suppressing autoimmune T cells Citation[135]. Further dissection of the role of temperature on NK cell mediated cytotoxic activity will greatly assist the efforts of clinical and laboratory scientists to identify optimal hyperthermia protocols for more successful therapies in patients that can harness long term disease control by the immune systems.
Acknowledgements
This work was supported by grants NIH P01 CA94045, R01 CA71599, R21 CA098852, and the Roswell Park Cancer Institute Core grant CA16056. The authors would like to thank Melissa Grimm, Chen-ting Lee and Thomas Mace for their helpful comments on the manuscript.
References
- Nauts HC, McLaren JR. Coley toxins - The first century. Adv Exp Med Biol 1990; 267: 483–500
- Wust P, et al. Hyperthermia in combined treatment of cancer. Lancet Oncol 2002; 3: 487–497
- Harima Y, et al. A randomized clinical trial of radiation therapy versus thermoradiotherapy in stage IIIB cervical carcinoma. Int J Hyperthermia 2001; 17: 97–105
- Dewhirst MW, et al. Re-setting the biologic rationale for thermal therapy. Int J Hyperthermia 2005; 21: 779–790
- Sharma S, et al. Side-effects of local hyperthermia: Results of a prospectively randomized clinical study. Int J Hyperthermia 1990; 6: 279–285
- Sneed PK, et al. Survival benefit of hyperthermia in a prospective randomized trial of brachytherapy boost +/− hyperthermia for glioblastoma multiforme. Int J Radiat Oncol Biol Phys 1998; 40: 287–295
- van der Zee J, et al. Comparison of radiotherapy alone with radiotherapy plus hyperthermia in locally advanced pelvic tumours: A prospective, randomised, multicentre trial. Dutch Deep Hyperthermia Group. Lancet 2000; 355: 1119–1125
- Falk MH, Issels RD. Hyperthermia in oncology. Int J Hyperthermia 2001; 17: 1–18
- Issels R, Schlemmer M, Lindner L. The role of hyperthermia in combined treatment in the management of soft tissue sarcoma. Curr Oncol Rep 2006; 8: 305–309
- Corry PM, Armour EP. The heat shock response: Role in radiation biology and cancer therapy. Int J Hyperthermia 2005; 21: 769–778
- Horsman MR, Overgaard J. Can mild hyperthermia improve tumour oxygenation?. Int J Hyperthermia 1997; 13: 141–147
- Shakil A, Osborn JL, Song CW. Changes in oxygenation status and blood flow in a rat tumor model by mild temperature hyperthermia. Int J Radiat Oncol Biol Phys 1999; 43: 859–865
- Song CW, et al. Implications of increased tumor blood flow and oxygenation caused by mild temperature hyperthermia in tumor treatment. Int J Hyperthermia 2005; 21: 761–767
- Thrall DE, et al. Changes in tumour oxygenation during fractionated hyperthermia and radiation therapy in spontaneous canine sarcomas. Int J Hyperthermia 2006; 22: 365–373
- Repasky EA, Issels RD. Hyperthermia and the Immune System. Int J Hyperthermia 2002; 18: 485–615
- Ostberg JR, Repasky EA. Emerging evidence indicates that physiologically relevant thermal stress regulates dendritic cell function. Cancer Immunol Immunother 2006; 55: 292–298
- Wang XY, Ostberg JR, Repasky EA. Effect of fever-like whole-body hyperthermia on lymphocyte spectrin distribution protein kinase C activity and uropod formation. J Immunol 1999; 162: 3378–3387
- Di YP, Repasky EA, Subjeck JR. Distribution of HSP70 protein kinase C and spectrin is altered in lymphocytes during a fever-like hyperthermia exposure. Journal of Cellular Physiology 1997; 172: 44–54
- Noessner E. Thermal stress-related modulation of tumor cell physiology and immune responses. Cancer Immunol Immunother 2006; 55: 289–291
- Chen Q, et al. Dynamic control of lymphocyte trafficking by fever-range thermal stress. Cancer Immunol Immunother 2006; 55: 299–311
- Multhoff G, et al. CD3- large granular lymphocytes recognize a heat-inducible immunogenic determinant associated with the 72-kD heat shock protein on human sarcoma cells. Blood 1995; 86: 1374–1382
- Ljunggren HG, Karre K. In search of the ‘missing self’: MHC molecules and NK cell recognition. Immunol Today 1990; 11: 237–244
- Karre K. NK cells MHC class I molecules and the missing self. Scand J Immunol 2002; 55: 221–228
- O'Leary JG, et al. T cell- and B cell-independent adaptive immunity mediated by natural killer cells. Nat Immunol 2006; 7: 507–516
- Kelly JM, et al. Induction of tumor-specific T cell memory by NK cell-mediated tumor rejection. Nat Immunol 2002; 3: 83–90
- Biron CA, et al. Natural killer cells in antiviral defense: Function and regulation by innate cytokines. Annu Rev Immunol 1999; 17: 189–220
- Smyth MJ, et al. An essential role for tumor necrosis factor in natural killer cell-mediated tumor rejection in the peritoneum. J Exp Med 1998; 188: 1611–1619
- Pilaro AM, et al. TNF-alpha is a principal cytokine involved in the recruitment of NK cells to liver parenchyma. J Immunol 1994; 153: 333–342
- Young HA, Ortaldo J. Cytokines as critical co-stimulatory molecules in modulating the immune response of natural killer cells. Cell Res 2006; 16: 20–24
- Lotzova E. Definition and functions of natural killer cells. Nat Immun 1993; 12: 169–176
- Nguyen KB, et al. Coordinated and distinct roles for IFN-alpha beta IL-12 and IL-15 regulation of NK cell responses to viral infection. J Immunol 2002; 169: 4279–4287
- Carson WE, et al. Interleukin (IL) 15 is a novel cytokine that activates human natural killer cells via components of the IL-2 receptor. J Exp Med 1994; 180: 1395–1403
- Kluger MJ. Fever revisited. Pediatrics 1992; 90: 846–850
- Scholz H. Fever. Am J Physiol Regul Integr Comp Physiol 2003; 284: R913–R915
- Ho EL, et al. Costimulation of multiple NK cell activation receptors by NKG2D. J Immunol 2002; 169: 3667–3675
- Jamieson AM, et al. The role of the NKG2D immunoreceptor in immune cell activation and natural killing. Immunity 2002; 17: 19–29
- Regunathan J, et al. NKG2D receptor-mediated NK cell function is regulated by inhibitory Ly49 receptors. Blood 2005; 105: 233–240
- Sivori S, et al. NKp46 is the major triggering receptor involved in the natural cytotoxicity of fresh or cultured human NK cells. Correlation between surface density of NKp46 and natural cytotoxicity against autologous allogeneic or xenogeneic target cells. Eur J Immunol 1999; 29: 1656–1666
- Pende D, et al. Identification and molecular characterization of NKp30 a novel triggering receptor involved in natural cytotoxicity mediated by human natural killer cells. J Exp Med 1999; 190: 1505–1516
- Vitale M, et al. NKp44 a novel triggering surface molecule specifically expressed by activated natural killer cells is involved in non-major histocompatibility complex-restricted tumor cell lysis. J Exp Med 1998; 187: 2065–2072
- Bottino C, et al. The human natural cytotoxicity receptors (NCR) that induce HLA class I-independent NK cell triggering. Hum Immunol 2000; 61: 1–6
- Borrego F, et al. Structure and function of major histocompatibility complex (MHC) class I specific receptors expressed on human natural killer (NK) cells. Mol Immunol 2002; 38: 637–660
- McQueen KL, Parham P. Variable receptors controlling activation and inhibition of NK cells. Curr Opin Immunol 2002; 14: 615–621
- Smyth MJ, Godfrey DI, Trapani JA. A fresh look at tumor immunosurveillance and immunotherapy. Nat Immunol 2001; 2: 293–299
- Lou Z, et al. A balance between positive and negative signals in cytotoxic lymphocytes regulates the polarization of lipid rafts during the development of cell-mediated killing. J Exp Med 2000; 191: 347–354
- Zanker KS, Lange J. Whole body hyperthermia and natural killer cell activity. Lancet 1982; 1: 1079–1080
- Nakayama J, et al. Kinetics of immunological parameters in patients with malignant melanoma treated with hyperthermic isolated limb perfusion. J Dermatol Sci 1997; 15: 1–8
- Szmigielski S, et al. Effects of local prostatic hyperthermia on human NK and T cell function. Int J Hyperthermia 1991; 7: 869–880
- Robins HI, et al. Phase I trial of human lymphoblastoid interferon with whole body hyperthermia in advanced cancer. Cancer Res 1989; 49: 1609–1615
- Blazickova S, et al. Effect of hyperthermic water bath on parameters of cellular immunity. Int J Clin Pharmacol Res 2000; 20: 41–46
- Kraybill WG, et al. A phase I study of fever-range whole body hyperthermia (FR-WBH) in patients with advanced solid tumours: Correlation with mouse models. Int J Hyperthermia 2002; 18: 253–266
- Ahlers O, et al. Stress induced changes in lymphocyte subpopulations and associated cytokines during whole body hyperthermia of 41.8–42.2°C. Eur J Appl Physiol 2005; 95: 298–306
- Atanackovic D, et al. Patients with solid tumors treated with high-temperature whole body hyperthermia show a redistribution of naive/memory T-cell subtypes. Am J Physiol Regul Integr Comp Physiol 2006; 290: R585–R594
- Tomiyama-Miyaji C, et al. Modulation of the endocrine and immune systems by well-controlled hyperthermia equipment. Biomed Res 2007; 28: 119–125
- Fujieda S, et al. Heat shock enhances the susceptibility of tumor cells to lysis by lymphokine-activated killer cells. Arch Otolaryngol Head Neck Surg 1995; 121: 1009–1014
- Kim JY, et al. Increase of NKG2D ligands and sensitivity to NK cell-mediated cytotoxicity of tumor cells by heat shock and ionizing radiation. Exp Mol Med 2006; 38: 474–484
- Ostberg JR, et al. Enhancement of natural killer (NK) cell cytotoxicity by fever-range thermal stress is dependent on NKG2D function and is associated with plasma membrane NKG2D clustering and increased expression of MICA on target cells. J Leukoc Biol 2007
- Burd R, et al. Tumor cell apoptosis lymphocyte recruitment and tumor vascular changes are induced by low temperature long duration (fever-like) whole body hyperthermia. J Cell Physiol 1998; 177: 137–147
- Kubes J, et al. Immunological response in the mouse melanoma model after local hyperthermia. Physiol Res 2007
- Azocar J, Yunis EJ, Essex M. Sensitivity of human natural killer cells to hyperthermia. Lancet 1982; 1: 16–17
- Nurmi T, Uhari M, Kouvalainen K. Temperature and natural killer cell activity. Lancet 1982; 1: 516–517
- Dinarello CA, et al. Inhibitory effects of elevated temperature on human cytokine production and natural killer activity. Cancer Res 1986; 46: 6236–6241
- Won SJ, Lin MT. Thermal stresses reduce natural killer cell cytotoxicity. J Appl Physiol 1995; 79: 732–737
- Ozveri ES, et al. The effect of hyperthermic preconditioning on the immune system in rat peritonitis. Intensive Care Med 1999; 25: 1155–1159
- Gulluoglu BM, et al. Optimal timing and temperature for hyperthermic preconditioning in an animal model of fecal peritonitis. J Invest Surg 2002; 15: 117–124
- Nakayama J, et al. Experimental approaches for the treatment of murine B16 melanomas of various sizes. I: Local injection of ethanol with a combination of interleukin-2 or microwaval hyperthermia for B16 melanomas with a size of less than 7 mm in diameter. J Dermatol Sci 1997; 15: 75–81
- Nakayama J, et al. A combined therapeutic modality with hyperthermia and locally administered rIFN-beta inhibited the growth of B16 melanoma in association with the modulation of cellular infiltrates. J Dermatol Sci 1993; 6: 240–246
- Nakayama J, et al. Experimental approaches for the treatment of murine B16 melanomas of various sizes. II: Injection of ethanol with combinations of beta-interferon and microwaval hyperthermia for B16 melanomas with a size of greater than 10 mm in diameter. J Dermatol Sci 1997; 15: 82–88
- Nakayama J, et al. In situ detection of immunocompetent cells in murine B16 melanoma locally treated with interleukin-2 or microwaval hyperthermia. Pigment Cell Res 1993; 6: 111–116
- Jaattela M. Effects of heat shock on cytolysis mediated by NK cells LAK cells activated monocytes and TNFs alpha and beta. Scand J Immunol 1990; 31: 175–182
- Milani V, Noessner E. Effects of thermal stress on tumor antigenicity and recognition by immune effector cells. Cancer Immunol Immunother 2006; 55: 312–319
- Fleischmann WR, Jr, Fleischmann CM, Gindhart TD. Effect of hyperthermia on the antiproliferative activities of murine alpha- beta- and gamma-interferon: Differential enhancement of murine gamma-interferon. Cancer Res 1986; 46: 8–13
- Eleme K, et al. Cell surface organization of stress-inducible proteins ULBP and MICA that stimulate human NK cells and T cells via NKG2D. J Exp Med 2004; 199: 1005–1010
- Endt J, et al. Inhibitory receptor signals suppress ligation-induced recruitment of NKG2D to GM1-rich membrane domains at the human NK cell immune synapse. J Immunol 2007; 178: 5606–5611
- Tanaka M, et al. Effect of heat-pretreatment on interleukin-2-activated killer cells for in vitro purging. Pathobiology 2000; 68: 124–128
- Lamon EW, et al. Synergistic induction of thermotolerance in murine natural killer cells by interferon alpha and mild heat shock. Radiat Res 1994; 139: 364–369
- Raulet DH, Vance RE. Self-tolerance of natural killer cells. Nat Rev Immunol 2006; 6: 520–531
- Blom DJ, et al. Effect of hyperthermia on expression of histocompatibility antigens and heat-shock protein molecules on three human ocular melanoma cell lines. Melanoma Res 1997; 7: 103–109
- Multhoff G. Heat shock protein 72 (HSP72) a hyperthermia-inducible immunogenic determinant on leukemic K562 and Ewing's sarcoma cells. Int J Hyperthermia 1997; 13: 39–48
- Ito A, et al. Augmentation of MHC class I antigen presentation via heat shock protein expression by hyperthermia. Cancer Immunol Immunother 2001; 50: 515–522
- Kubista B, et al. Hyperthermia increases the susceptibility of chondro- and osteosarcoma cells to natural killer cell-mediated lysis. Anticancer Res 2002; 22: 789–792
- Roigas J, et al. Heat shock protein (HSP72) surface expression enhances the lysis of a human renal cell carcinoma by IL-2 stimulated NK cells. Adv Exp Med Biol 1998; 451: 225–229
- Basu S, Srivastava PK. Fever-like temperature induces maturation of dendritic cells through induction of HSP90. Int Immunol 2003; 15: 1053–1061
- Gastpar R, et al. Heat shock protein 70 surface-positive tumor exosomes stimulate migratory and cytolytic activity of natural killer cells. Cancer Res 2005; 65: 5238–5247
- Gastpar R, et al. The cell surface-localized heat shock protein 70 epitope TKD induces migration and cytolytic activity selectively in human NK cells. J Immunol 2004; 172: 972–980
- Zhang H, et al. Comparison of the anti-tumor effects of various whole-body hyperthermia protocols: Correlation with HSP 70 expression and composition of splenic lymphocytes. Immunol Invest 2005; 34: 245–258
- Jantschitsch C, et al. Overexpression of Hsp25 in K1735 murine melanoma cells enhances susceptibility to natural killer cytotoxicity. Cell Stress Chaperones 2002; 7: 107–117
- Gross C, et al. Cell surface-bound heat shock protein 70 (Hsp70) mediates perforin-independent apoptosis by specific binding and uptake of granzyme B. J Biol Chem 2003; 278: 41173–41181
- Gross C, et al. Heat shock protein 70-reactivity is associated with increased cell surface density of CD94/CD56 on primary natural killer cells. Cell Stress Chaperones 2003; 8: 348–360
- Tanaka K, et al. Intratumoral injection of immature dendritic cells enhances antitumor effect of hyperthermia using magnetic nanoparticles. Int J Cancer 2005; 116: 624–633
- Milani V, et al. Heat shock protein 70: Role in antigen presentation and immune stimulation. Int J Hyperthermia 2002; 18: 563–575
- Multhoff G. Activation of natural killer cells by heat shock protein 70. Int J Hyperthermia 2002; 18: 576–585
- Yang Y, Li Z. Roles of heat shock protein gp96 in the ER quality control: redundant or unique function?. Mol Cells 2005; 20: 173–182
- Kozutsumi Y, et al. The presence of malfolded proteins in the endoplasmic reticulum signals the induction of glucose-regulated proteins. Nature 1988; 332: 462–464
- Pilla L, et al. Natural killer and NK-Like T-cell activation in colorectal carcinoma patients treated with autologous tumor-derived heat shock protein 96. Cancer Res 2005; 65: 3942–3949
- Shinagawa N, et al. Immunotherapy with dendritic cells pulsed with tumor-derived gp96 against murine lung cancer is effective through immune response of CD8(+) cytotoxic T lymphocytes and natural killer cells. Cancer Immunol Immunother 2007
- Bauer S, et al. Activation of NK cells and T cells by NKG2D a receptor for stress-inducible MICA. Science 1999; 285: 727–729
- Diefenbach A, Raulet DH. Natural killer cells: Stress out turn on tune in. Curr Biol 1999; 9: R851–R853
- Groh V, et al. Cell stress-regulated human major histocompatibility complex class I gene expressed in gastrointestinal epithelium. Proc Natl Acad Sci USA 1996; 93: 12445–12450
- Venkataraman GM, et al. Promoter region architecture and transcriptional regulation of the genes for the MHC class I-related chain A and B ligands of NKG2D. J Immunol 2007; 178: 961–969
- Rodriguez-Rodero S, et al. Transcriptional regulation of MICA and MICB: A novel polymorphism in MICB promoter alters transcriptional regulation by Sp1. Eur J Immunol 2007; 37: 1938–1953
- Davis DM, et al. The human natural killer cell immune synapse. Proc Natl Acad Sci USA 1999; 96: 15062–15067
- Vyas YM, et al. Spatial organization of signal transduction molecules in the NK cell immune synapses during MHC class I-regulated noncytolytic and cytolytic interactions. J Immunol 2001; 167: 4358–4567
- McCann FE, et al. The activating NKG2D ligand MHC class I-related chain A transfers from target cells to NK cells in a manner that allows functional consequences. J Immunol 2007; 178: 3418–3426
- Roda-Navarro P, et al. Transfer of NKG2D and MICB at the cytotoxic NK cell immune synapse correlates with a reduction in NK cell cytotoxic function. Proc Natl Acad Sci USA 2006; 103: 11258–11263
- McCann FE, et al. The size of the synaptic cleft and distinct distributions of filamentous actin ezrin CD43 and CD45 at activating and inhibitory human NK cell immune synapses. J Immunol 2003; 170: 2862–2870
- Salih HR, Rammensee HG, Steinle A. Cutting edge: Down-regulation of MICA on human tumors by proteolytic shedding. J Immunol 2002; 169: 4098–4102
- Salih HR, Goehlsdorf D, Steinle A. Release of MICB molecules by tumor cells: Mechanism and soluble MICB in sera of cancer patients. Hum Immunol 2006; 67: 188–195
- Waldhauer I, Steinle A. Proteolytic release of soluble UL16-binding protein 2 from tumor cells. Cancer Res 2006; 66: 2520–2526
- Onsrud M. Effects of hyperthermia on human natural killer cells. Acta Pathol Microbiol Immunol Scand [C] 1983; 91: 1–8
- Kalland T, Dahlquist I. Effects of in vitro hyperthermia on human natural killer cells. Cancer Res 1983; 43: 1842–1846
- Johnston RL, et al. Effects of in vivo ultrasound hyperthermia on natural killer cell cytotoxicity in the hamster. Bioelectromagnetics 1986; 7: 283–293
- Downing JF, Taylor MW. The effect of in vivo hyperthermia on selected lymphokines in man. Lymphokine Res 1987; 6: 103–109
- Hughes CS, et al. Effects of hyperthermia on spectrin expression patterns of murine lymphocytes. Radiat Res 1987; 112: 116–123
- Shen RN, et al. Effect of whole-body hyperthermia and cyclophosphamide on natural killer cell activity in murine erythroleukemia. Cancer Res 1988; 48: 4561–4563
- Yoshioka A, Miyachi Y, Imamura S. Immunological effects of in vitro hyperthermia. J Clin Lab Immunol 1989; 29: 95–97
- Yang H, Mitchel R, Lemaire I. The effects of in vitro hyperthermia on natural killer activity from lung blood and spleen. J Clin Lab Immunol 1990; 32: 117–122
- Yoshioka A, et al. Effects of local hyperthermia on natural killer activity in mice. Int J Hyperthermia 1990; 6: 261–267
- Kappel M, et al. Effects of in vivo hyperthermia on natural killer cell activity in vitro proliferative responses and blood mononuclear cell subpopulations. Clin Exp Immunol 1991; 84: 175–180
- Lu L, et al. Efficacy of recombinant human macrophage colony-stimulating factor in combination with whole-body hyperthermia in the treatment of mice infected with the polycythemia-inducing strain of the Friend virus complex. Exp Hematol 1991; 19: 804–809
- Yang HX, Mitchel RE. Hyperthermic inactivation recovery and induced thermotolerance of human natural killer cell lytic function. Int J Hyperthermia 1991; 7: 35–49
- Taradi M, et al. Augmentation of mouse natural killer cell activity by combined hyperthermia and streptococcal preparation OK-432 (Picibanil) treatment. Int J Hyperthermia 1991; 7: 653–665
- Yang H, Lauzon W, Lemaire I. Effects of hyperthermia on natural killer cells: Inhibition of lytic function and microtubule organization. Int J Hyperthermia 1992; 8: 87–97
- Lu L, et al. In vivo effects of purified recombinant human macrophage colony-stimulating factor in combination with local hyperthermia on tumor progression in B16a melanoma bearing mice. Int J Hematol 1993; 58: 139–152
- Pedersen BK, Ullum H. NK cell response to physical activity: Possible mechanisms of action. Med Sci Sports Exerc 1994; 26: 140–146
- Kappel M, et al. Somatostatin attenuates the hyperthermia induced increase in neutrophil concentration. Eur J Appl Physiol Occup Physiol 1998; 77: 149–156
- Fuggetta MP, et al. In vitro effect of hyperthermia on natural cell-mediated cytotoxicity. Anticancer Res 2000; 20: 1667–1672
- Payne J, et al. Mild hyperthermia modulates biological activities of interferons. Int J Hyperthermia 2000; 16: 492–507
- Rabinovich BA, et al. Stress renders T cell blasts sensitive to killing by activated syngeneic NK cells. J Immunol 2000; 165: 2390–2397
- Gulluoglu BM, et al. Immunologic influences of hyperthermia in a rat model of obstructive jaundice. Dig Dis Sci 2001; 46: 2378–2384
- Atanackovic D, et al. 41.8 degrees C whole body hyperthermia as an adjunct to chemotherapy induces prolonged T cell activation in patients with various malignant diseases. Cancer Immunol Immunother 2002; 51: 603–613
- Dieing A, et al. Whole body hyperthermia induces apoptosis in subpopulations of blood lymphocytes. Immunobiology 2003; 207: 265–273
- Ostapenko VV, et al. Immune-related effects of local hyperthermia in patients with primary liver cancer. Hepatogastroenterology 2005; 52: 1502–1506
- Koga T, et al. Hyperthermia suppresses the cytotoxicity of NK cells via down-regulation of perforin/granzyme B expression. Biochem Biophys Res Commun 2005; 337: 1319–1323
- Capitano ML, et al. Fever-range whole body hyperthermia prevents the onset of type 1 diabetes in non-obese diabetic mice. Int J Hyperthermia 2008, (in press)