Abstract
Purpose: We examined whether hyperthermia attenuated the metastatic potential of colon cancer through the induction of heat shock protein 70 (Hsp70).
Materials and methods: Colon26 cells were separated into four groups: (1) no pretreatment, (2) hyperthermia at 42°C for 1 hour, (3) pretreatment with geranylgeranylacetone (GGA) 10−6 M for 2 hours, and (4) hyperthermia after GGA treatment. We measured cell viabilities and the contents of Hsp70. We assessed nuclear factor-κ-B (NF-κ-B) status with and without tumor necrosis factor-α (TNF-α) stimulation. For in vivo study, colon26 cells were injected via the tail vein or into a subcutaneous area of mice and the numbers of lung metastatic nodules or the volumes of subcutaneous tumors were assessed. Untreated cells were incubated with PKH26. Experimental metastasis models were then generated and used to assess the fixed cancer cells.
Results: Tumor development in the subcutaneous tumor models and cell viabilities were similar among the four groups. However, the GGA plus hyperthermia group had fewer lung metastatic nodules in the experimental lung metastasis model and higher Hsp70 induction than the other cell groups. The GGA plus hyperthermia pretreatment group also showed a lower number of fixed cells in lungs and lower activation of NF-κ-B by TNF-α than the other cell groups.
Conclusions: It is suggested the metastatic potential but not the proliferation potency of cancer cells is inhibited by the transient induction of Hsp70.
Introduction
The transcription factor NF-κ-B mediates many gene expressions, such as cyclinD1, ornithine decarboxylase (ODC) and vascular endothelial growth factor (VEGF) as cell proliferation factors, cytokines, chemokines, nitric oxide (NO), adhesion molecules, cyclooxygenase-2 (COX-2) and matrix metalloproteinase (MMP) as inflammation factors, Bcl-xL and inhibitors of apoptosis (IAPs) such as cIAP1 and cIAP2 as anti-apoptosis proteins, and I-κ-B α and β as negative feedback components Citation1–3. In many human cancers, activated NF-κ-B induces the expression of multiple genes, including cyclinD1, COX-2, VEGF, and Bcl-2 Citation4–8. Therefore, the blocking of NF-κ-B activity may be associated with the suppression of cell proliferation, angiogenesis, and induction of apoptosis Citation9–14.
Recently, we confirmed that heat treatment inhibited the activation of NF-κ-B in a human colon cancer cell line and an arterial endothelial cell line Citation15, Citation16. Other researchers reported that the heat shock protein (Hsp) inhibited the activation of NF-κ-B through the inhibition of I-κ-B Citation17.
Thus, these facts raise a possible scenario of Hsp70 induced by hyperthermia inhibiting the activation of NF-κ-B. In other words, we speculated that hyperthermia might provide an anti-tumor effect by both direct cytocidal action due to fever and by indirect blocking of NF-κ-B in the cancer cells.
We previously reported that the combination of Hsp derivative with hyperthermia enhanced the anti-tumor effects of hyperthermia under an experimental condition in which hyperthermia alone inhibited tumor growth in a mouse subcutaneous tumor model and metastases to the lung in a mouse model Citation18, Citation19. However, it is not clear whether the suppression of the metastases to the lung results from the suppression of the metastasis process or from the growth suppression of lung nodules that were already implanted. To clarify this point, we investigated hyperthermia treatment and induction of Hsp for cancer cells. Treated cancer cells were implanted subcutaneously or seeded into lungs. The Hsp derivative that we used in a mouse subcutaneous tumor model and mouse metastases to lung model in our prior and current studies is geranylgeranylacetone (GGA). This has been clinically used as an anti-gastric ulcer drug in Japan that protects gastric mucosa by inducing Hsp70. In addition, GGA can induce other heat shock proteins such as Hsp60 and Hsp90 by activating heat shock factor-1 Citation20, Citation21.
The major aims of this study were to investigate: (1) whether hyperthermia inhibits the hematogenous metastases of cancer cells to the lungs, (2) whether any inhibitory effects are associated with the cytocidal action of hyperthermia, cancer cell growth inhibition, or inhibition of metastasis morphosis, and (3) whether the induction of Hsp is involved in the suppression of metastases.
Materials and methods
Animals
We used 7-week-old male BALB/c mice purchased from Shimizu Animal Inc. (Kyoto, Japan). Mice were maintained under our standard laboratory conditions. The Animal Care Committee of Kyoto Prefectural University of Medicine (Kyoto, Japan) approved all of the experimental procedures described below.
Cancer cell line
The colon26 murine colon adenocarcinoma cell line Citation22 was kindly provided by Dr A. Hagiwara (Department of Digestive Surgery, Kyoto Prefectural University of Medicine) and was maintained in monolayer cultures in RPMI-1640 medium supplemented with 10% fetal bovine serum and L-glutamine at 37°C in a humidified atmosphere of 5% CO2.
Reagents
All chemicals in this study were prepared immediately before use. GGA was provided by Eisai (Tokyo, Japan). Recombinant human tumor necrosis factor-α (TNF-α) was purchased from R&D Systems (Rockville, MD). A PKH26 red fluorescent cell linker kit was purchased from Sigma-Aldrich Japan. We used these reagents according to the manufacturers’ recommendations.
Cell treatments
Colon26 cells were separated into four groups and pretreated in vitro. The first group of colon26 cells was only pretreated in a temperature-controlled incubator at 37°C; these were the control cells. The second group of cells was pretreated with hyperthermia at 42°C for 1 h. The third group of cells was incubated with GGA (10−6 M) for 2 h. The fourth group of cells was pretreated with hyperthermia after incubation with GGA. After colon26 cells in the second and fourth groups were pretreated with hyperthermia, they were exposed to passive cooling in a temperature-controlled CO2 incubator (37°C).
After each pretreatment, colon26 cells were evaluated by the following examinations.
Cell viability assay
Cell viability was measured by WST-1 assay (tetrazolium salts-based assay) (Dojin Laboratory, Kumamoto, Japan) according to the manufacturer's instructions. Briefly, WST-1 solution was added to cells in 96-well plates, cells were incubated at 37°C for 1 h, and the optical density of each well was read at 450 nm.
ELISA for NF-κ-B
Nuclear proteins that were extracted from pretreated colon26 cells were assayed with a sensitive enzyme-linked immunosorbent assay (ELISA) kit (IMGENEX, San Diego, CA) according to the manufacturer's protocol and the NF-κ-B binding activity was quantified.
Western blot analysis for Hsp70
Pretreated colon26 cells were thawed on ice and homogenized at 4°C in a solution of 50 mmol/L Tris-HCL, pH 7.6, 300 mmol/L NaCl, 0.5% Triton X-100, 10 μg/mL aprotinin, 10 μg/mL leuptin, 1 mmol/L phenylmethylsulfonyl fluoride, 1.8 mg/mL iodoacetamide, 50 mmol/L NaF, and 1 mM DTT for extracting total cell protein. Proteins were separated by sodium dodecyl sulfate-polyacrylamide gel electrophoresis (SDS-PAGE) and transferred on to nitrocellulose membranes (Bio-Rad Laboratories, Hercules, CA). These membranes were probed with specific antibody against Hsp70 (Stressgen, Victoria, Canada). The immune complexes were visualized by western blotting with a commercial kit (ECL by Amersham, UK) according to the manufacturer's recommendations.
Colon26 subcutaneous tumor model
To establish tumors in mice, 1.0 × 106 viable pretreated colon26 cells in 100 μL of saline were injected subcutaneously in the mid-dorsal region. We measured the tumor size 18 days later. The major and minor axes of tumors were measured and volumes were calculated using the formula:
Colon26 experimental metastasis model
To establish experimental metastases tumors in mice, 2.0 × 105 viable colon26 cells in 500 μL of saline were injected via the tail vein. Cancer cells were allowed to infiltrate and proliferate in lung tissue. The control mice were injected with saline at the same time points in an identical manner. We counted the metastatic nodules on the lung surface by injecting India ink into the bronchus 14 days after the injection of cancer cells.
For estimating the fixed metastatic cells in the lungs, we used a PKH26 red fluorescent cell linker kit according to the manufacturer's recommendations. The cells were incubated with PKH26 before injection.
Statistical analysis
Results are presented as mean ± standard error of the mean (SEM). Data were compared using one-way analysis of variance and differences were considered significant for values of P < 0.05 according to PLSD or Scheffe's F multiple comparison test. All analyses were performed using Stat View 4.11-J software (Abacus Concepts, Berkeley, CA) on a computer with a Windows operating system.
Results
Expression of Hsp70 by western blot
We have already examined the time course of the expression of Hsp70 after hyperthermia. Hyperthermia induced the expression of Hsp70 3 h later, with a peak 24 h later and return to a prehyperthermia state 48 h later. Therefore, to estimate the expression of Hsp70 by GGA and/or hyperthermia, we examined the status of Hsp70 by western blotting 24 h after pretreatment with GGA and/or hyperthermia. Compared with the control group, the hyperthermia, GGA, and GGA plus hyperthermia treated groups showed higher levels of expression of Hsp70. The GGA plus hyperthermia pretreatment induced the highest expression of Hsp70 ().
Figure 1. The accumulation of Hsp70 proteins induced by pretreatment with GGA and/or hyperthermia. Colon26 adenocarcinoma cells were pretreated with GGA and/or hyperthermia. The induction of Hsp70 24 h after treatment is shown. The accumulation of Hsp70 was assessed by western blotting. Lane 1, untreated control cells; Lane 2, pretreatment with GGA; Lane 3, pretreatment with hyperthermia; Lane 4, pretreatment with GGA plus hyperthermia. We used β-actin as a loading control and found the molecular weight of Hsp70 (70 kD).
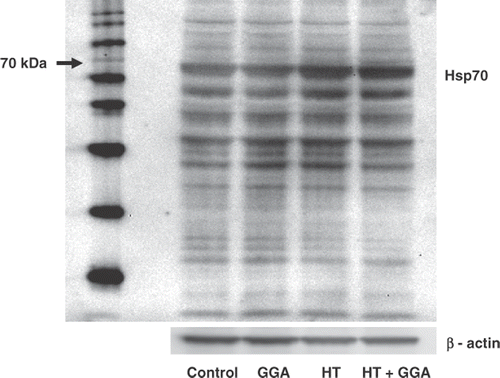
Effect of GGA and hyperthermia on cell viability
To assess the effect of GGA and/or hyperthermia on cell viability, we estimated the status of cell viability by WST-1 assay 24 h after pretreatment with GGA and/or hyperthermia. The results showed no remarkable difference in cell viability between the control group and the GGA plus hyperthermia treated group. Hyperthermia alone significantly decreased the cancer cell viability as compared with the control group ().
Figure 2. The cell viability of colon26 cells after each pretreatment. Colon26 adenocarcinoma cells were pretreated with GGA and/or hyperthermia. After each pretreatment, we examined the cell viability with WST-1. Data are presented as the mean ± SEM of five samples. *P < 0.01 compared with the control.
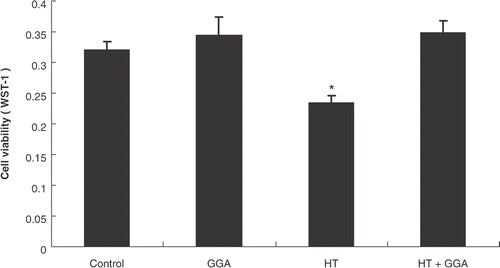
Effect of GGA and hyperthermia on the growth of subcutaneous tumors
To examine the effect of GGA and/or hyperthermia on colon26 cancer cells, we assessed the subcutaneous tumor model. We injected each group of cancer cells subcutaneously in the mid-dorsal region and measured the tumor size 18 days after injection. Compared with the tumors developed from the control cells, the tumors developed from the GGA and/or hyperthermia treatment cells did not show a significant difference in their volumes ().
Effect of GGA and hyperthermia on the development of experimental lung metastatic nodules
To assess the effect of GGA and/or hyperthermia on lung metastatic nodules, we used experimental metastasis models created by injecting the treatment cells via the tail vein of mice. We removed the lungs 14 days after the injection of cancer cells and injected India ink into the bronchi. Compared with the control group, the GGA group, hyperthermia group, and GGA plus hyperthermia treated groups showed a decrease in the lung metastatic nodules (). By statistical analysis, the GGA group, hyperthermia group, and the GGA plus hyperthermia group showed a significantly decreased number of lung metastatic nodules compared with the control group ().
Figure 4. Lung metastatic nodules of each group of pretreated colon26 cells in an experimental lung metastasis model. (A) Macroscopic observation of lung metastatic nodules from untreated, GGA pretreated, hyperthermia pretreated, and GGA plus hyperthermia pretreated groups. (B) Formations of lung nodules by colon26 cells. We counted the metastatic nodules on the lung surface 14 days after cancer cell injection. Data are presented as the mean ± SEM of five mice. *P < 0.05 compared with the control, **P < 0.001 compared with the control, ***P < 0.0001 compared with the control.

Effect of GGA and hyperthermia on binding activity of NF-κ-B
Following pretreatment of the colon26 cells, we extracted the nuclear protein and measured the binding activity of NF-κ-B by ELISA. At the same time, the four groups of colon26 cells were stimulated with recombinant TNF-α (50 ng/ml) for 1 h. After the end of stimulation by TNF-α, we measured the binding activity of NF-κ-B by ELISA. Without stimulation with TNF-α, there were no significant differences among the groups regarding the binding activity of NF-κ-B, but after the stimulation with TNF-α, compared with the control group, the GGA plus hyperthermia pretreatment cells significantly decreased the binding activity of NF-κ-B ().
Figure 5. Effect of GGA plus hyperthermia on binding activity of NF-κ B. The binding activity of NF-κ B of each group of pretreated cells was measured by ELISA. At the same time, each group of pretreated cells was stimulated with recombinant TNF-α (50 ng/ml) for 1 h; after stimulation with TNF-α, we measured the binding activity of NF-κ B by ELISA. Data are shown as the mean ± SEM of five samples in a representative experiment. *P < 0.005 compared with the control + TNF-α stimulation group, #P < 0.05 compared with GGA + TNF-α stimulation group, and hyperthermia + TNF-α stimulation group.
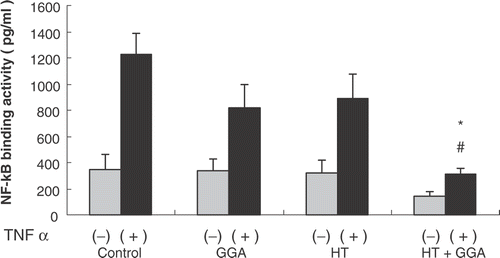
Effect of GGA and hyperthermia on the adhesion of cancer cells to lungs
For estimating the number of cells that adhered to the lungs 24 h after injecting the cells via the tail vein, we used the fluorescent dye PKH26. At that time, we removed the lungs from mice and examined lung slices with a fluorescent microscope (). We estimated five views with 200× magnification for each group. The number of PKH26-positive cells was significantly decreased by the treatment of GGA, hyperthermia, and GGA plus hyperthermia as compared with the control. GGA plus hyperthermia treatment significantly decreased the number of PKH26-positive cells as compared with GGA alone or hyperthermia alone ().
Figure 6. Colon26 cells stained with PKH26 in the lungs. (A) Microscopic observation of experimental lung metastases from untreated, GGA pretreated, hyperthermia pretreated, and GGA plus hyperthermia pretreated groups with the fluorescent dye PKH26 using a fluorescent microscope. (B) Statistical results of microscopic findings of experimental lung metastases by colon26 cells with PKH26. We counted the number of microscopic PKH26-positive cells 24 h after cancer cell injection. We estimated five views with 200× magnification for each group. Data are presented as the mean ± SEM of five mice. *P < 0.001 compared with the control, #P < 0.001 compared with GGA group and hyperthermia group.
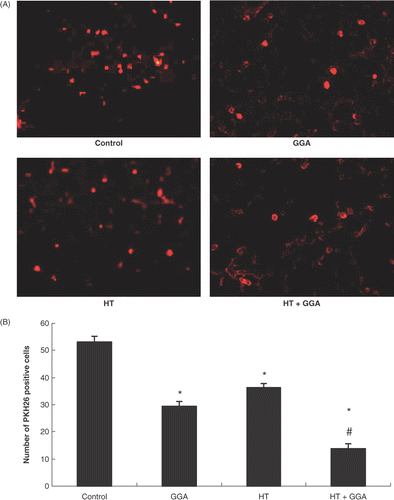
Discussion
This study suggests that hyperthermia decreases metastatic potential, probably by the induction of Hsp70. The present results that the GGA combined with hyperthermia group showed the highest level expression of the heat shock protein Hsp70 suggests that GGA actually increases the Hsp70 induction by hyperthermia. Hsp70 is known to show cytoprotective effects as a molecular chaperone Citation23–26. The present cell viability studies revealed that although hyperthermia shows a significant cytotoxic effect against colon26 cells, treatment of GGA combined with hyperthermia cancels this cytotoxic effect. On the other hand, it is reported that Hsp70 enhances the anti-cancer effects of hyperthermia and immunotherapy Citation27, Citation28. Although the role of Hsp70 in these cancer therapies is relatively well known, the present knowledge on the detailed mechanisms by which Hsp70 mediated these cancer therapies remains incomplete. GGA is the inducer of various heat shock proteins, especially Hsp70. Previous studies from our laboratory have demonstrated that hyperthermia performed twice a week inhibited the tumor growth in a mouse subcutaneous model and that the combination of GGA with hyperthermia increased the antitumor effects of hyperthermia and induced the expression of Hsp70, which stabilized 24 hours after exposure to GGA and hyperthermia. These effects seem to be due to the inhibition of NF-κ-B activation in cancer cells by Hsp70. Previous studies from our laboratory have also demonstrated that the inhibition of NF-κ-B significantly inhibited the tumor growth in a subcutaneous tumor model and significantly attenuated the lung metastatic nodules in a mouse experimental metastasis model. Thus, it has been postulated that Hsp70 induced by GGA and hyperthermia inhibit tumor growth and lung metastatic nodules via the blocking of NF-κ-B activation of cancer cells. In this study, we generated subcutaneous tumor models from each of the pretreated cancer cell groups and found that tumor growth induced by GGA plus hyperthermia pretreated cells was similar to that induced by control cells, GGA pretreated cells, and hyperthermia pretreated cells. On the other hand, in the experimental metastasis models generated by injecting each of the pretreated colon26 cell groups via the tail vein, the GGA plus hyperthermia pretreatment of colon26 cells showed significant suppression of lung metastatic nodules as compared with the other pretreatment groups or the control. Taken together with the previous reports, this study strongly suggests that transient induction of Hsp70 by GGA plus hyperthermia could not inhibit the proliferation of cancer cells, but could inhibit the metastasis. This scenario is supported by the results of our viability assay. We found that the each group of pretreated cells maintains the proliferation potency, shown by the results of the subcutaneous tumor model and cell viability with WST-1. Otherwise, the suppression of experimental metastasis was induced by GGA and hyperthermia treatment. In the next series of experiments we examined whether the expression of Hsp70 is related to the suppression of lung metastatic nodules. To clarify this point, we further investigated whether the Hsp70 induced by GGA and/or hyperthermia suppresses the binding activity of NF-κ-B in cancer cells.
We found suppression of the binding activity of NF-κ-B by the treatment of GGA plus hyperthermia as compared with the control cells. In addition, we stimulated colon26 cells with TNF-α after each pretreatment and measured the binding activity of NF-κ-B. By stimulating each group of pretreated cells with TNF-α, NF-κ-B in colon26 cells was strongly activated. However, GGA plus hyperthermia treatment attenuated the binding activity of NF-κ-B induced by TNF-α as compared with other treatments. Therefore, it is concluded that the expression of Hsp70 by GGA plus hyperthermia treatment induced the suppression of the binding activity of NF-κ-B, by which GGA plus hyperthermia could inhibit the lung metastatic nodules. However, the expression of Hsp70 by GGA plus hyperthermia treatment was transient, as was the inhibition of NF-κ-B (data not shown). Thus, GGA plus hyperthermia could not inhibit the subcutaneous tumor growth. This conclusion is supported by other studies indicating that Hsp70 inhibited the activation of NF-κ-B through the inhibition of I-κ-B Citation29–34. However, the data presented here do not exclude the possibility that other heat shock proteins or other factors inhibit the metastases and the activation of NF-κ-B, which is a limitation of this study.
Metastasis of cancer cells has several phases, such as defection from the original tumor, invasion into the vessel channel, and adhesion and breakout in the metastasis organ. In our experimental metastasis model, which only approximates clinical metastasis, the cancer cells were thought to be in the phase after defection from the original tumor, so we estimated the cancer cells that adhered to the vessels of the lungs. We stained the treated cells with PKH26 and generated the lung metastatic nodules by injecting these cells via the tail vein; 24 h after injection we examined the number of experimental metastatic cells with a fluorescent microscope. We found a decrease in the number of experimental metastatic PKH26-positive cells by cells treated with GGA plus hyperthermia as compared with the control cells. These results indicated that GGA plus hyperthermia inhibited the adhesion of colon26 cancer cells to lung vessels. Previous studies from our laboratory and other groups have demonstrated that hyperthermia attenuates the expression of endothelial adhesion molecules on endothelial cells Citation16. In this study, hyperthermia was performed on cancer cells but not on lung endothelial cells. However, it is possible that hyperthermia attenuates the expression of adhesion molecules on cancer cells. Therefore, further detailed examinations are required to evaluate this point. In addition, the present results do not eliminate the involvement of other mechanisms such as immune responses immediately after the cancer cells adhere to lung vessels. It has been reported recently that stimulation of the immune system might also contribute to the therapeutic effects of hyperthermia Citation35–37.
In summary, GGA plus hyperthermia suppressed the NF-κ-B binding activity and inhibited the metastases of colon26 cells to the lungs but did not inhibit the proliferation potency of colon26 cells. This inhibitory effect was associated with the expression of Hsp70. We have therefore concluded that hyperthermia decreases metastatic potential, probably by the induction of Hsp70. In clinical practice, it is suggested that Hsp70 must be induced in tumor tissue by hyperthermia and that induction may be increased when GGA is combined with hyperthermia. In general, cancer causes distant metastasis from a primary tumor during chemotherapy and radiotherapy or naturally. In such a case, hyperthermia might be useful for preventing distant metastasis.
Declaration of interest: The authors report no conflicts of interest. The authors alone are responsible for the content and writing of the paper.
References
- Barnes PJ, Karin M. Nuclear factor-κ B: A pivotal transcription factor in chronic inflammatory diseases. N Engl J Med 1997; 336: 1066–1071
- Aggarwal BB. Nuclear factor-κ B: The enemy within. Cancer Cell 2004; 6: 203–208
- Karin M, Cao Y, Greten FR, Li ZW. NF-κ B in cancer: From innocent bystander to major culprit. Nat Rev Cancer 2002; 2: 301–310
- Shishodia S, Aggarwal BB. Nuclear factor-κ B activation mediates cellular transformation, proliferation, invasion angiogenesis and metastasis of cancer. Cancer Treat Res 2004; 119: 139–173
- Allen C, Duffy S, Teknos T, Islam M, Chen Z, Albert PS, Wolf G, Van Waes C. Nuclear factor-κ B-related serum factors as longitudinal biomarkers of response and survival in advanced oropharyngeal carcinoma. Clin Cancer Res 2007; 13: 3182–3190
- Han JC, Zhang KL, Chen XY, Jiang HF, Kong QY, Sun Y, Wu ML, Huang L, Li H, Liu J. Expression of seven gastric cancer-associated genes and its relevance for WNT, NF-κ B and STAT3 signaling. APMIS 2007; 115: 1331–1343
- Stathopoulos GT, Kollintza A, Moschos C, Psallidas I, Sherrill TP, Pitsinos EN, Vassiliou S, Karatza M, Papiris SA, Graf D, et al. Tumor necrosis factor-alpha promotes malignant pleural effusion. Cancer Res 2007; 67: 9825–9834
- Levine L, Lucci JA, Pazdrak B, Cheng JZ, Guo YS, Townsend CM, Jr, Hellmich MR. Bombesin stimulates nuclear factor κ B activation and expression of proangiogenic factors in prostate cancer cells. Cancer Res 2003; 63: 3495–3502
- Rahman KM, Ali S, Aboukameel A, Sarkar SH, Wang Z, Philip PA, Sakr WA, Raz A. Inactivation of NF-κ B by 3,3′-diindolylmethane contributes to increased apoptosis induced by chemotherapeutic agent in breast cancer cells. Mol Cancer Ther 2007; 6: 2757–2765
- Ahn KS, Sethi G, Aggarwal BB. Simvastatin potentiates TNF-alpha-induced apoptosis through the down-regulation of NF-κ B-dependent antiapoptotic gene products: Role of I κ-B alpha kinase and TGF-beta-activated kinase-1. J Immunol 2007; 178: 2507–2516
- Takada Y, Gillenwater A, Ichikawa H, Aggarwal BB. Suberoylanilide hydroxamic acid potentiates apoptosis, inhibits invasion, and abolishes osteoclastogenesis by suppressing nuclear factor-κ B activation. J Biol Chem 2006; 281: 5612–5622
- Yemelyanov A, Gasparian A, Lindholm P, Dang L, Pierce JW, Kisseljov F, Karseladze A, Budunova I. Effects of IKK inhibitor PS1145 on NF-κ B function, proliferation, apoptosis and invasion activity in prostate carcinoma cells. Oncogene 2006; 25: 387–398
- Xu C, Shen G, Chen C, Gelinas C, Kong AN. Suppression of NF-κ B and NF-κ B-regulated gene expression by sulforaphane and peitc through I κ B alpha, IKK pathway in human prostate cancer pc-3 cells. Oncogene 2005; 24: 4486–4495
- Javelaud D, Poupon MF, Wietzerbin J, Besancon F. Inhibition of constitutive NF-κ B activity suppresses tumorigenicity of Ewing sarcoma EW7 cells. Int J Cancer 2002; 98: 193–198
- Kokura S, Yoshida N, Ueda M, Imamoto E, Ishikawa T, Takagi T, Naito Y, Okanoue T, Yoshikawa T. Hyperthermia enhances tumor necrosis factor alpha-induced apoptosis of a human gastric cancer cell line. Cancer Lett 2003; 201: 89–96
- Nakabe N, Kokura S, Shimozawa M, Katada K, Sakamoto N, Ishikawa T, Handa O, Takagi T, Naito Y, Yoshida N, et al. Hyperthermia attenuates TNF-alpha-induced up regulation of endothelial cell adhesion molecules in human arterial endothelial cells. Int J Hyperthermia 2007; 23: 217–224
- Ran R, Lu A, Zhang L, Tang Y, Zhu H, Xu H, Feng Y, Han C, Zhou G, Rigby AC. Sharp FR. Hsp70 promotes TNF-mediated apoptosis by binding IKK gamma and impairing NF-κ B survival signaling. Genes Dev 2004; 18: 1466–1481
- Adachi S, Kokura S, Okuda T, Hattori T, Takagi T, Handa O, Naito Y, Yoshida N, Yoshikawa T. HSP70-inducing drug, Geranylgeranylacetone, enhances anti-tumor effect of whole body hyperthermia in mice subcutaneous tumor models of colon cancer. Gastroenterology 2006; 130: A–583
- Okayama T, Kokura S, Okuda T, Adachi S, Hattori T, Nakabe N, Sakamoto N, Takagi T, Handa O, Naito Y, et al. Antitumor effect of whole body hyperthermia combined with Geranylgeranylacetone in mice pulmonary metastasis models of colon cancer. Gastroenterology 2006; 130: A679
- Murakami M, Oketani K, Fujisaki H, Wakabayashi T, Ohgo T. Antiulcer effect of geranylgeranylacetone, a new acyclic polyisoprenoid on experimentally induced gastric and duodenal ulcers in rats. Arzneimittelforschung 1981; 31: 799–804
- Hirakawa T, Rokutan K, Nikawa T, Kishi K. Geranylgeranylacetone induces heat shock proteins in cultured guinea pig gastric mucosal cells and rat gastric mucosa. Gastroenterology 1996; 111: 345–57
- Nakagawa R, Motoki K, Ueno H, Iijima R, Nakamura H, Kobayashi E, Shimosaka A, Koezuka Y. Treatment of hepatic metastasis of the colon26 adenocarcinoma with an alpha-galactosylceramide, KRN7000. Cancer Res 1998; 58: 1202–1207
- Odashima M, Otaka M, Matsuhashi T, Jin M, Horikawa Y, Ohba R, Itoh H, Watanabe S. Mechanical strain stress suppresses expression of Hsp70 and wound restoration in gastric mucosal cells. Dig Dis Sci 2007; 52: 3087–3091
- Franklin TB, Krueger-Naug AM, Clarke DB, Arrigo AP, Currie RW. The role of heat shock proteins Hsp70 and Hsp27 in cellular protection of the central nervous system. Int J Hyperthermia 2005; 21: 379–392
- Yeo M, Park HK, Kim DK, Cho SW, Kim YS, Cho SY, Paik YK, Hahm KB. Restoration of heat shock protein 70 suppresses gastric mucosal inducible nitric oxide synthase expression induced by Helicobacter pylori. Proteomics 2004; 4: 3335–3342
- Barnes JA, Dix DJ, Collins BW, Luft C, Allen JW. Expression of inducible Hsp70 enhances the proliferation of MCF-7 breast cancer cells and protects against the cytotoxic effects of hyperthermia. Cell Stress Chaperones 2001; 6: 316–325
- Kawai N, Ito A, Nakahara Y, Futakuchi M, Shirai T, Honda H, Kobayashi T, Kohri K. Anticancer effect of hyperthermia on prostate cancer mediated by magnetite cationic liposomes and immune-response induction in transplanted syngeneic rats. Prostate 2005; 64: 373–381
- Suzuki M, Shinkai M, Honda H, Kobayashi T. Anticancer effect and immune induction by hyperthermia of malignant melanoma using magnetite cationic liposomes. Melanoma Res 2003; 13: 129–135
- Weiss YG, Bromberg Z, Raj N, Raphael J, Goloubinoff P, Ben-Neriah Y, Deutschman CS. Enhanced heat shock protein 70 expression alters proteasomal degradation of I κ B kinase in experimental acute respiratory distress syndrome. Crit Care Med 2007; 35: 2128–2138
- Savion S, Sofer M, Brengauz-Breitmann M, Fein A, Torchinsky A, Toder V. NF-κ B regulates the response of embryonic cells to heat shock. Int J Hyperthermia 2007; 23: 277–286
- King TA, Ghazaleh RA, Juhn SK, Adams GL, Ondrey FG. Induction of heat shock protein 70 inhibits NF-κ-B in squamous cell carcinoma. Otolaryngol Head Neck Surg 2005; 133: 70–79
- Lee KH, Lee CT, Kim YW, Han SK, Shim YS, Yoo CG. Heat shock protein 70 negatively regulates the heat-shock-induced suppression of the I κ B/NF-κ B cascade by facilitating I κ B kinase renaturation and blocking its further denaturation. Exp Cell Res 2005; 307: 276–284
- Chen Y, Arrigo AP, Currie RW. Heat shock treatment suppresses angiotensin II-induced activation of NF-κ B pathway and heart inflammation: A role for IKK depletion by heat shock?. Am J Physiol Heart Circ Physiol 2004; 287: H1104–1114
- Heneka MT, Gavrilyuk V, Landreth GE, O'Banion MK, Weinberg G, Feinstein DL. Noradrenergic depletion increases inflammatory responses in brain: Effects on I κ B and Hsp70 expression. J Neurochem 2003; 85: 387–398
- Milani V, Noessner E, Ghose S, Kuppner M, Ahrens B, Scharner A, Gastpar R, Issels RD. Heat shock protein 70: Role in antigen presentation and immune stimulation. Int J Hyperthermia 2002; 18: 563–575
- Ostberg JR, Patel R, Repasky EA. Regulation of immune activity by mild (fever-range) whole body hyperthermia: Effects on epidermal Langerhans cells. Cell Stress Chaperones 2000; 5: 458–461
- Ostberg JR, Repasky EA. Emerging evidence indicates that physiologically relevant thermal stress regulates dendritic cell function. Cancer Immunol Immunother 2006; 55: 292–298