Abstract
NAD(P)H:quinone oxidoreductase (NQO1) mediates cell death caused by the novel anti‐cancer drug β‐lapachone (β‐lap). Therefore, β‐lap sensitivity of cells is positively related to the level of cellular NQO1. Heat shock up‐regulates NQO1 expression in cancer cells, thereby enhancing the clonogenic cell death caused by β‐lap. The mechanisms by which heat shock elevates NQO1 expression were investigated in the present study using human A549 lung cancer cells and human MDA‐MB‐231 breast cancer cells. When MDA‐MB‐231(NQO1+) cells stably transfected with NQO1 were heated at 42°C for 1 h the expression of NQO1 and the sensitivity of the cells to β‐lap progressively increased during the 24–48 h post‐heating period. Heating increased NQO1 transcription by cis‐acting elements such as xenobiotic response element and antioxidant response element located in the NQO1 gene promoter region. The turnover of NQO1 protein in heated cells was much slower than in unheated cells. NQO1 and heat shock protein 70 (Hsp70) co‐precipitated and co‐localised in cells before and after heating, demonstrating the close association of these two proteins in the cells. These results suggest that NQO1 is stabilised by the Hsp70 molecular chaperone. It is concluded that the prolonged increase in NQO1 expression after heat shock is due to increased NQO1 transcription, and also increased Hsp70‐mediated NQO1 stabilisation.
Introduction
NAD(P)H:quinone oxidoreductase (NQO1), an obligatory two‐electron reductase, detoxifies various harmful quinones by catalysing two‐electron reduction of the quinones to hydroquinones using NADH or NAD(P)H as the electron source Citation[1–4]. The hydroquinones are then conjugated with glutathione, glucuronic acid or other moieties, and are removed from the cells Citation[1]. In this respect, the chemo‐preventive activity of dimethyl fumarate found in vegetables and fruits has been attributed to their ability to up‐regulate NQO1 and other phase II detoxifying enzymes in cells Citation[3–7]. On the other hand, certain quinone‐containing compounds become powerful anti‐cancer forms when they are two‐electron reduced through mediation by NQO1. For example, NQO1 activates mitomycin C (MMC) to a hydroquinone form, which is far more potent than the parent MMC as an alkylating agent Citation[1], Citation[13–19]. In recent years, several new NQO1‐dependent anti‐cancer compounds have been developed such as 3‐hydroxy‐5‐aziridinyl‐1‐methyl-2 [indol‐4,7‐dione]‐prop‐β‐en‐α‐a‐o10 (EO9) Citation[1], Citation[10], Citation[12]; 2,5‐diaziridinyl-3-3[hydroxymethyl]‐6‐methyl-1,4‐benzoquinone (RHI) Citation[1], Citation[11]; and 3,4‐dihydro‐2,2‐dimethyl-2H‐naphthol[1,2-b]pyran‐5,6‐dione (β‐lapachone, β‐lap) Citation[13–19].
β‐lap, originally obtained from the bark of the Lapacho tree in South America, possesses divergent pharmacological activities including a potent anti‐cancer effect Citation[16–19]. We as well as other investigators have demonstrated that the β‐lap‐induced apoptosis and clonogenic death of cancer cells are positively related to NQO1 expression in the target cells Citation[13–15], Citation[22–26]. Indeed, dicoumarol, an inhibitor of NQO1 Citation[22–26], and small interfering RNA (siRNA)‐NQO1 Citation[24] effectively reduce the cytotoxicity of β‐lap. Furthermore, stable transfection of NQO1‐expressing plasmid into cells devoid of NQO1 increases the cellular sensitivity to β‐lap Citation[13]. NQO1 has been suggested to cause a futile redox cycle between parent β‐lap and its two‐electron reduced hydroquinone form, thereby causing generation of reactive oxygen species (ROS), Ca2+‐dependent DNA damage, hyperactivation of poly ADP ribose polymerase‐1, and decrease in the NAD+ and ATP pools, which ultimately leads to cell death Citation[13–15], Citation[15].
Importantly, the level of NQO1 in various human tumours is significantly higher than that in adjacent normal tissues Citation[15], Citation[22–24]. For example, NQO1 gene expression in human hepatocarcinoma and hepatoblastoma is 20–50-fold and 50-fold higher, respectively, than that in normal liver tissues Citation[29]. This fact points to the possibility that cancer cells can be preferentially killed, relative to normal cells, by agents whose cytotoxicity depends on NQO1 activity. β‐lap not only kills cancer cells, but also sensitises cells to radiation by inhibiting repair of radiation‐induced DNA damage Citation[22–24], Citation[22–24]. We have reported that an exposure of cancer cells to ionising radiation at clinically relevant doses (e.g., 2 Gy) significantly up‐regulates the NQO1 level, thereby increasing the sensitivity of the cells to β‐lap treatment Citation[22–24]. We have also recently reported that heating cancer cells for 1 h at mild temperatures (e.g. 41°–42°C) causes a long‐lasting elevation of NQO1 expression and sensitises cells to β‐lap in vitro Citation[25], Citation[26]. Furthermore, FSaII tumours of mice or human tumour xenografts could be markedly sensitised to β‐lap treatment by heating the tumours 24 h prior to β‐lap treatment Citation[25], Citation[26]. These observations demonstrated that regional or local treatment of tumours with established cancer treatment modalities such as radiotherapy or hyperthermia may up‐regulate NQO1 in the tumours and selectively sensitise tumour cells to β‐lap. Phase I/II clinical trials are in progress to assess the feasibility of using β‐lap alone or in combination with other chemotherapeutic drugs against various human cancers Citation[34]. The means to improve the delivery of β‐lap to tumour are being investigated Citation[35], Citation[36].
The human NQO1 gene promoter region contains several essential cis‐elements including xenobiotic response element (XRE) and antioxidant response element (ARE) Citation[1], Citation[37]. The increased expression of the NQO1 gene by oxidative stress, antioxidants, heavy metals, ionising radiation and hypoxia has been reported to be regulated by ARE Citation[31], Citation[22–25], which contains several receptors for several nuclear transcription factors such as Nrf2 and negative transcription factors such as Bach1 Citation[41]. Whether and how heat shock activates the NQO1 gene has not yet been elucidated. It is well known that heat stress rapidly increases cytoprotective heat shock proteins (Hsps), including Hsp40, Hsp70 and Hsp90, which function as molecular chaperones, and thus stabilises various proteins in the cells. In this respect, NQO1 has been reported to be stabilised by a molecular chaperone Hsp70 Citation[5], Citation[42]. The possibility that the prolonged expression of NQO1 protein in heated cells, as alluded to above, could be due to protection of NQO1 protein by Hsp70 was investigated in the present study. Here, we report for the first time that the prolonged increase in NQO1 protein level in cancer cells after heat shock is caused by increased transcription for NQO1 protein, and also by increased Hsp70-induced stabilisation of NQO1.
Material and methods
Cell lines
Human A549 lung carcinoma cells were purchased from the American Type Culture Collection (Manassas, VA). Human MDA‐MB‐231 (NQO1−) breast carcinoma cells deficient of NQO1, and subline MDA‐MB‐231 (NQO1+) cells, stably transfected with nqo1 Citation[13], were obtained from Dr David A. Boothman (University of Texas Southwestern Medical Center, Dallas, TX). The A549 cells and MDA‐MB‐231 cells were cultured in Dulbecco's modified eagle medium (Invitrogen, Carlsbad, CA), supplemented with 10% (v/v) fetal bovine serum (Invitrogen), and 10 units/mL of penicillin/streptomycin (Invitrogen). Cells were grown at 37°C in an atmosphere of 95% O2 and 5% CO2. All experiments were conducted using cells in exponential growth phase Citation[13], Citation[25].
Clonogenic survival assay
Clonogenic survival of cells after β‐lap treatment was determined as described previously. After treating with β‐lap, cells were rinsed and cultured with complete media for 10–14 days. The colonies formed were fixed with a mixture of methanol and acetic acid (10:1 v/v) and stained with 1% crystal violet in 20% ethanol. The number of colonies containing more than 50 cells was counted, and the survivals of clonogenic cells were calculated Citation[22–25].
Cloning of NQO1 upstream promoter region
Fragments containing the upstream promoter region of human NQO1 were isolated from a human genomic library (Promega, Madison, WI) by polymerase chain reaction (PCR) strategy designed to introduce specific enzyme sites (KpnI/XhoI) within the proximal promoter region using the following oligomers: NQO1‐p1, 5′‐ggtaccGCCACCATCAAGTCGCTCTC‐3′; NQO1‐p2, 5′‐ggtaccGATCTCGAC CCACTGCAACC‐3′; NQO1‐p3, 5′‐ggtaccCTTGAGGAGCAGGGGTGGTG‐3′; NQO1‐p4, 5′‐ggtaccCTCTTGCACCTCAGGGCACAG‐3′; NQO1‐p5, 5′‐ggtaccCCT TGTGGCTGTCCCCTC‐3′; and NQO1‐pRev, 5′‐ctcgagGACCATGGCTCTGGTGCAGT C‐3′. The PCR products of heterologous human NQO1 promoter were cloned into pGEM‐T (Promega) and all fragments were verified by sequencing (Macrogen, Seoul, Korea) Citation[43], Citation[44].
Promoter assay
Human wild‐type NQO1 promoter regions were subcloned into the pGL3 expression vectors at the KpnI/XhoI restriction site. Plasmids pGL3‐NQO1p1 (−3223/+117), ‐NQO1p2 (−950/+117), ‐NQO1p3 (−617/+117), ‐NQO1p4 (−328/+117), and ‐NQO1p5 (−76/+117) were generated for the luciferase assay. A549 cells and MDA‐MB231 (NQO1+) cells at 80% confluence were transfected with the promoter‐luciferase construct using Lipofectamin LTX reagent (Invitrogen) according to the manufacturer's protocol. The pRL‐SV40 Renilla reporter vector was also used as an internal control. The transfected cells were incubated overnight under regular culture conditions, and heated at 42°C for 1 h. Cells were lysed, and Renilla and firefly luciferase activities were measured with the Dual‐Luciferase® reporter assay system (Promega) and a Model TD‐20/20 luminometer (Turner Designs, Sunnyvale, CA). Luciferase activities were normalised to internal control Renilla luminescence Citation[45–47]. All transfections experiments were repeated three times.
Reverse transcriptase‐PCR (RT‐PCR)
First‐strand cDNA from 1 μg of total RNA was generated using Superscript III reverse transcriptase (Invitrogen). PCR was performed with 25 μL of a reaction mixture consisting of 0.6 μM of each primer, 200 μM of each dNTP, 5 units of Tfi DNA polymerase (Invitrogen), and cDNA using an iCycler Thermal Cycler (Bio‐Rad). PCR reaction mixtures were subjected to 30 cycles of amplification consisting of 30 s at 94°C, 30 s at 55°C, and 30 s at 72°C. The final extension was preformed for 10 min at 72°C. The following primers were synthesised for RT‐PCR: NQO1 forward 5′‐GAGGACCTCCTTCAACTATG−3′; NQO1 reverse 5′‐CCTTTGTCATACATGGC AGC−3′; β‐actin forward 5′‐ATCAAG ATCCTGACCGA GCG−3′; β‐actin reverse 5′‐TACTTGCGCTCAGGAGGAGC−3′. The PCR products were separated by electrophoresis on 2% agarose gels Citation[48], Citation[49]. PCR band intensities were measured by Scion Image (Scion, Frederick, Maryland).
Western blot analysis
To determine the cellular levels of NQO1 and Hsp70 proteins, cell lysates were prepared with solubilising buffer (1% Triton X-100, 0.1% sodium dodecyl sulphate (SDS), 20 mM Tris‐HCL, 150 mM NaCl, 1 mM EDTA, 1 mM sodium ortho‐vanadate, 1 mM sodium fluoride, 2 mM phenylmethanesulphonylfluoride, 10 mM iodoacetamide, 10 μg/mL aprotinin, 10 μg/mL leupeptin). Equal amounts of protein (20 μg) from total cell extracts were resolved by 10% SDS–polyacrylamide gel electrophoresis (SDS‐PAGE), and transferred to a Hybond‐P membrane (Amersham Life Sciences, Arlington Heights, IL). Each membrane was then blocked with 3% non‐fat dry milk and labelled with either of the following primary antibodies: anti‐NQO1 antibody (Zymed, Carlsbad, CA), anti‐Hsp70 (Santa Cruz Biotechnology, Santa Cruz, CA), or anti‐β‐actin antibody (Sigma‐Aldrich, St Louis, MO). Each blot was then labelled with horseradish peroxidase (HRP)‐conjugated anti‐mouse or anti‐goat IgG secondary antibody (GE Healthcare, Little Chalfont, UK), and the immunoreactive bands were visualised using chemiluminescence (Pierce Biotechnology, Rockford, IL) []. Western blots were scanned, and band intensities were measured using either Scion Image or ImageJ 1.41 software (National Institutes of Health, Bethesda, MD).
Immunoprecipitation of NQO1 and Hsp70
Cell lysates prepared as described above were mixed with 10 µL of mouse anti‐NQO1 monoclonal antibody (Zymed) or 10 µL of mouse anti‐Hsp70 antibody (Santa Cruz Biotechnology). The mixtures were gently rotated at 4°C for 16 h, and protein‐antibody complexes were precipitated using a protein G immunoprecipitation kit (Sigma‐Aldrich) according to the manufacturer's protocol. The immunoprecipitated proteins were then analysed using Western blot analysis Citation[50], Citation[51].
NQO1 mRNA and NQO1 protein turnover rates
The turnover rates of NQO1 mRNA and NQO1 protein in heated cells were investigated. MDA‐MB‐231 (NQO1+) cells and A549 cells were heated at 42°C for 1 h and incubated at 37°C for 4 h in regular media to allow for expression of heat‐induced changes in the levels of NQO1 mRNA and NQO1 protein. The cells were then incubated with either 4 μg/mL actinomycin D (Act D, Sigma‐Aldrich) or 50 µg/mL cycloheximide (CHX, Sigma‐Aldrich) at 37°C to inhibit synthesis of new NQO1 mRNA or expression of new NQO1 protein. Cells were harvested after incubating with the inhibitors for various lengths of time and the quantities of NQO1 mRNA and protein were analysed using RT‐PCR and immunoblotting, respectively Citation[44], Citation[52].
Cellular localisation of NQO1 and Hsp70
Cellular localisation of NQO1 and Hsp70 was determined by immuno‐fluorescence staining. MB‐231 (NQO1+) cells grown on cover glasses were rinsed with phosphate buffered saline (PBS), and fixed with 3.7% paraformaldehyde. After blocking with 1% bovine serum albumin, the cells were incubated with rabbit anti‐NQO1 antibody and mouse anti‐Hsp70 antibody. Fluorescein isothiocynate (FITC)‐conjugated anti‐rabbit secondary antibody and Texas‐Red conjugated anti‐mouse secondary antibody (Jackson Immuno Research, West Grove, PA) were used for visualisation of NQO1 and Hsp70, respectively. A Nikon C1 plus laser scanning TE2000‐E confocal microscope (Nikon, Tokyo, Japan) was used for the image analyses Citation[22], Citation[24], Citation[25].
Hsp70 siRNA transfection
Small interfering RNA (siRNA) interference of Hsp70 was studied using 21‐bp (including a 2‐deoxynucleotide overhang) siRNA duplexes purchased from Bioneer (Daejeon, South Korea). The sense strand nucleotide sequence for the Hsp70 siRNA was CAUUACCUUUUUCGAGAGUdTdT. A control siRNA specific to the GFP DNA sequence CCUACGCCACCAAUUUCGUdTdT was used as a negative control. The MDA‐MB‐231 (NQO1+) cells were seeded with 10% FBS media free of antibiotics, and incubated overnight. The cells were then incubated for 48 h with Hsp70 siRNA or negative control siRNA using Lipofectamine 2000 reagent (Invitrogen) following the manufacturer's instructions. The transfected MDA‐MB‐231 (NQO1+) cells were then heated at 42°C for 1 h, incubated at 37°C for varying lengths of times in normal growth media, and the expressions of NQO1 and Hsp70 proteins were analysed by Western blot Citation[53], Citation[54].
Results
NQO1 expression and cell death caused by β‐lap
shows the clonogenic death of MDA‐MB‐231(NQO1+) cells and MDA‐MB‐231(NQO1−) cells treated with varying concentrations of β‐lap for 4 h at 37°C. NQO1 expression in the MDA‐MB‐231(NQO1+) cells was high but was negligible in MDA‐MB‐231(NQO1−) cells (, inset). The clonogenic survival of MDA‐MB‐231(NQO1+) cells exponentially decreased as a function of β‐lap dose, with only 0.6% of the cell population surviving when treated with 10 µM β‐lap for 4 h. On the contrary, as much as 80% of MDA‐MB‐231 (NQO1−) cells survived the 4–h treatment with 10 µM β‐lap.
Figure 1. NQO1 expression and cell death caused by β-lap. (A) Effects of β-lap on clonogenic survival of MDA-MB-231(NQO1+) and MDA-MB-231(NQO1−) cells. As shown in the inset, NQO1 is over-expressed in MDA-MB-231(NQO1+) cells, while NQO1 expression in MDA-MB-231(NQO1−) cells is negligible. Cells were treated with different concentrations of β-lap for 4 h at 37°C, washed, cultured for 10–14 days, and the colonies were counted. An average of seven experiments ± SE is shown. (B) Effects of preheating on the sensitivity of MDA-MB-231(NQO1+) and MDA-MB-231(NQO1−) cells to β-lap. Cells were heated at 42°C for 1 h, incubated at 37°C for varying lengths of time, and treated with 5 µM β-lap for 4 h. After β-lap treatment, cells were washed, cultured for 10–14 days, and the colonies were counted. An average of six experiments ± SE is shown.
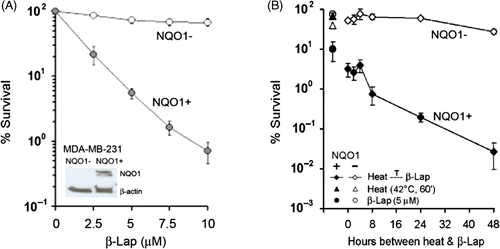
We previously reported that heating causes long‐lasting up‐regulation of NQO1 in cancer cells, so that the cellular sensitivity to β‐lap progressively increases after heating Citation[25]. As shown in , the survival of MDA‐MB‐231 (NQO1+) cells decreased to 4% when the cells were heated at 42°C for 1 h and immediately treated with 5 µM β‐lap for 4 h at 37°C. When MDA‐MB‐231 (NQO1+) cells were heated and then treated with β‐lap 24 or 48 h later, the % survival of the cells decreased to 0.2% and 0.03%, respectively. In contrast, MDA‐MB‐231 (NQO1−) cells were rather resistant to β‐lap, and, moreover, sensitivity of MDA‐MB‐231 (NQO1−) cells to β‐lap increased only slightly after heating.
Effect of heat shock on expression of NQO1 mRNA and NQO1 protein production
The expressions of NQO1 mRNA and NQO1 protein before and after heating at 42°C for 1 h in A549 cells and MDA‐MB 231(NQO1+) cells are shown in . In A549 cells, the expression of NQO1 mRNA increased 1–8 h after heating and then declined to the control level (). NQO1 mRNA expression in MDA‐MB 231(NQO1+) cells also increased for 8 h after heating. NQO1 protein level progressively increased for 24 h after heating in both A549 and MDA‐MB 231 (NQO1+) cells (). The expression of Hsp70 protein, used as a marker of heat treatment, also progressively increased after heating in both A549 and MDA‐MB 231(NQO1+) cells.
Figure 2. Expression of NQO1 mRNA and NQO1 protein production upon heat treatment. A549 and MDA-MB 231 cells (NQO1+) were heated at 42°C for 1 h, incubated at 37°C, and harvested at the times indicated. (A) The expression of NQO1 mRNA was analysed by RT-PCR. (B) Protein expression was determined by Western blot. Hsp70 is shown as a relevant marker for heat treatment. Each experiment was independently performed three times.
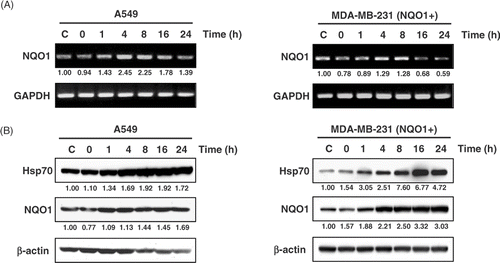
Effect of heat shock on NQO1 promoter activity
A series of deletions spanning up to −3223 of the 5′ upstream flanking region of human NQO1 were created in luciferase gene‐containing plasmids and amplified with PCR, as shown in . Both A549 and MDA‐MB 231 (NQO1+) cells transfected with the promoter‐luciferase constructs were heated at 42°C for 1 h, lysed 0–8 h later, and the luciferase activity of the lysates was determined. shows the luciferase activities normalised for internal control Renilla luminescence for each promoter deletion. In the unheated control A549 cells, the luciferase activity of NQO1p2 (−950/+117), which contained the cis‐acting XRE, ARE and AP‐2, was higher than that of other promoter deletions. For example, before heating, the luciferase activity of NQO1p2 (−950/+117) was 32‐fold higher than that of NQO1p5 (−76/+177). The luciferase activity of NQO1p3 (−617/+117), which contains ARE and AP2 regions but lacks XRE region, was slightly lower than that of NQO1p2 (−950/+177), which contains the XRE, ARE and AP2 regions. These observations indicated the relative unimportance of XRE and AP2. In unheated MDA‐MB‐231(NQO1+) cells, the luciferase activities of NQO1p2 (1617/+117) and NQO1p3 (−76/+177) were similar, again indicative of the unimportance of XRE on NQO1 transcription, relative to that of ARE in MDA‐MB‐231(NQO1+) cells. In both A549 and MDA‐MB‐231 (NQO1+) cells, the promoter activity of NQO1p1, NQO1p2 and NQO1p3 significantly increased immediately and 1 h after heating, and then returned to control levels. The promoter activities of NQO1p4 and NQO1p5 were negligible before heating and remained unchanged after heating.
Figure 3. Changes of NQO1 promoter activities in A549 and MDA-MB 231 cells by heat treatment. (A) NQO1 promoter-luciferase constructs were generated for transfection. Upstream promoter regions were generated by PCR. (B) Relative luciferase activities from various NQO1 promoter deletions in A549 and MDA-MB-231 cells. Five micrograms of test plasmid (pGL3-NQOp) and 1 µg of pRL-SV40 were co-transfected into A549 and MDA-MB 231 (NQO1+) cells. After 18 h incubation, the transfected cells were heated at 42°C for 1 h, and harvested for dual luciferase reporter assay. Data are expressed as relative luciferase activity to those of the construct, pGL3-NQO1p5. Promoter activity values represent the mean of three independent experiments.
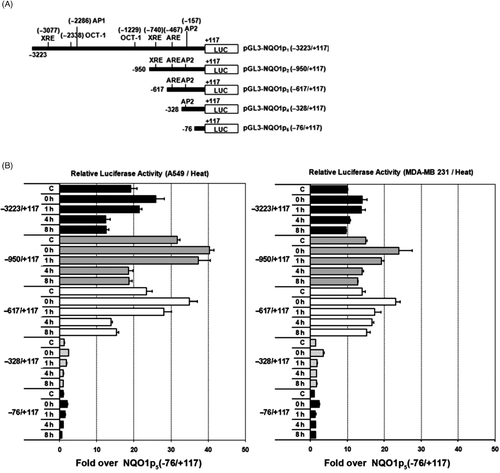
Effect of heat shock on turnover of NQO1 mRNA and NQO1 protein
Whether the heat‐induced increase in NQO1 expression is due to an elevation of NQO1 transcription or to an increase in NQO1 protein level or stability was investigated. shows the changes in NQOI mRNA level in A549 and MDA‐MB‐231 (NQO1+) cells heated for 1 h at 42°C, incubated for 4 h at 37°C, and then treated with 4 µg/mL of the transcription inhibitor Act D for 1–24 h. In both A549 and MDA‐MB‐231(NQO1+) cells, the NQO1 mRNA level gradually declined after 4–8 h treatment with Act D, regardless of heating. The rate of decrease in NQO1 mRNA upon incubation with Act D was similar in heated and unheated cells. When the synthesis of NQO1 protein was blocked from 4 h after heating at 42°C for 1 h with 50 µg/mL CHX, NQO1 protein levels remained unchanged for 24 h in both A549 and MDA‐MB‐231(NQO1+) cells. However, NQO1 protein level in unheated cells decreased when the cells were incubated with CHX for 8 h or longer. The prolonged increase in the NQO1 protein in heated cells as compared with unheated cells after the inhibited synthesis of new protein indicated that NQO1 protein was stabilised in heated cells.
Figure 4. Effects of Act D and CHX on NQO1 mRNA and protein expression, respectively, in A549 and MDA-MB 231 (NQO1+) cells. Cells were heated at 42°C for 1 h, incubated at 37°C for 4 h, and treated with 4 μg/mL Act D or 50 μg/mL CHX for the indicated times. (A) NQO1 mRNA expression was determined by RT-PCR. (B) NQO1 protein expression was determined by Western blot.
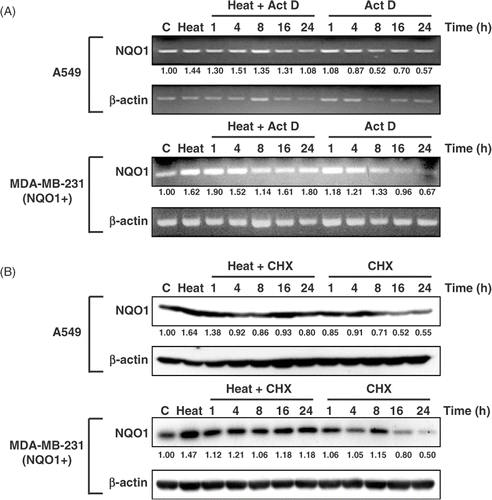
Effect of siHsp70 on stability of NQO1 protein
The effect of heating on the expressions of Hsp70 and NQO1 protein in the MDA‐MB‐231(NQO1+) cells transfected with Hsp70 siRNA or control siRNA are shown in . In the cells transfected with control siRNA, the expressions of both Hsp70 and NQO1 progressively increased for 24 h after heating. Importantly, in the cells transfected with Hsp70 siRNA, the expressions of both Hsp70 and NQO1 protein was markedly suppressed even before heating and both Hsp70 protein and NQO1 proteins remained unchanged after heating. Therefore, the prolonged increase in NQO1 protein in heated cell may be attributed to the chaperone effect of Hsp70, which is also up‐regulated by the heating.
Figure 5. Co-immunoprecipitation and localisation of NQO1 and Hsp70. (A) A549 and MDA-MB 231 cells (NQO1+) were harvested at 8 h after heating at 42°C for 1 h. Whole cell lysates were immunoprecipitated with mouse anti-Hsp70 antibody or mouse anti-NQO1 antibody. The precipitates were analysed by SDS-PAGE and blotted onto polyvinylidene fluoride membranes. The membranes were probed with rabbit anti-NQO1 antibody, or rabbit anti-Hsp70 antibody and binding was revealed by enhanced chemiluminescence. (B) A549 cell lysate was precipitated with anti-Hsp90 antibody or anti-Hsp40 antibody as previously described. (C) MDA-MB-231 (NQO1+) cells were transfected with siHsp70 or control siRNA and incubated 48h. Then the cells were heated at 42°C for 1 h and incubated for various times at 37°C. Expression of NQO1 and Hsp70 protein were analysed by Western blot. (D) Cells were heated at 42°C for 1 h, incubated for 8 h at 37°C, and then labelled for NQO1 (red) and Hsp70 (green) using FITC-conjugated anti-rabbit secondary antibody and Texas-Red conjugated anti-mouse secondary antibody, respectively. Labelled cells were examined using confocal microscopy.
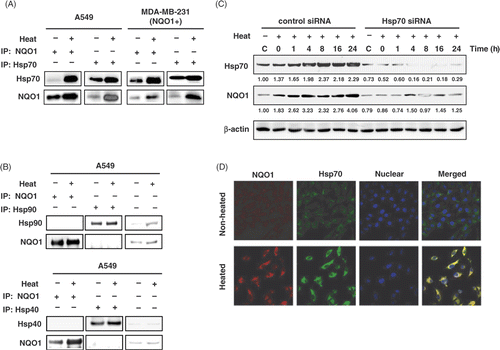
Interaction between NQO1 and Hsp70
As expected, heating increased Hsp70 expression in A549 and MDA-MB-231(NQO1+) cells (). Experiments were conducted to reveal whether the increase in NQO1 protein stability in heated cells () was related to the known ability of Hsp70 to stabilise or protect various proteins. Immunoprecipitates for NQO1 or Hsp70 contained both proteins before and 8 h after heating (), demonstrating the strong association of NQO1 and Hsp70 in both A549 and MDA-MB-231 (NQO1+) cells. Unlike Hsp70, neither Hsp40 nor Hsp90 co-precipitated with NQO1 protein (), indicating that Hsp40 and Hsp90 were not associated with NQO1 in A549 cells. The expression of both Hsp40 and Hsp90 increased 8 h after heating along with the increase in NQO1 expression in A549 cells. Confocal fluorescent microscopy revealed the co-localisation of NQO1 and Hsp70 in A549 cells, and a significantly increased expression of both NQO1 and Hsp70 8 h after heating ().
Discussion
In the present study, the NQO1 protein level in human A549 lung cancer cells and human MDA-MB-231(NQO1+) breast cancer cells significantly increased following heating at 42°C for 1 h. The prolonged increase in NQO1 protein level after heating enhanced the effect of β-lap to cause clonogenic cell death. The increase in NQO1 protein level after heating was due, in part, to increase in transcription of NQO1 at the anti-oxidant response element (ARE) in the NQO1 gene promoter region, and, in part, to stabilisation of the NQO1 protein by Hsp70.
MDA-MB 231 (NQO1+) cells were extremely sensitive to β-lap treatment as compared with MDA-MB 231 (NQO1-) cells (), confirming previous observations that β-lap cytotoxicity is positively related to the expression of NQO1 Citation[13–15], Citation[13–15]. NQO1 has been reported to mediate futile cycling between oxidised and two-electron reduced forms of β-lap, thereby triggering death signals resulting in DNA damage, apoptosis and clonogenic death in the cells Citation[13–15], Citation[20], Citation[21]. Ionising radiation up-regulates NQO1 expression in cancer cells, which, in turn, increases the sensitivity of the cells to β-lap Citation[22–24], Citation[55]. We have reported that heating at mild temperatures (i.e. 41–42°C) also elevates NQO1 expression in various human cancer cells including A549 lung cancer cells, thereby increasing β‐lap‐induced cell death Citation[25], Citation[26]. In good agreement with these previous observations, heating at 42°C for 1 h increased NQO1 expression in MDA‐MB 231(NQO1+) cells, and enhanced β‐lap‐induced clonogenic death in the present study ().
Although the mechanisms by which the NQO1 gene is activated by various stresses have been revealed, the mechanism underlying the up‐regulation of NQO1 expression by heat shock has not been elucidated. As shown in , heating at 42°C for 1 h elevated the promoter activity in the deletion containing cis‐elements XRE and ARE. The increased promoter activity in the deletion containing only ARE was not much lower than that in the deletion containing both XRE and ARE, demonstrating that ARE plays the major role in the heat‐induced up‐regulation of NQO1. In this respect, it has been reported that XRE cross‐reacts with ARE and participates in Nrf2‐dependent signalling in NQO1 induction Citation[44].
ActD‐mediated inhibition of transcription reduced the expression of NQO1 mRNA in both unheated and heated cells (). On the other hand, inhibition of NQO1 protein synthesis at the translational level with CHX resulted in the gradual decline in NQO1 protein in unheated cells, but not in heated cells. These results indicated that NQO1 protein is stabilised and, thus, the degradation of the NQO1 protein is decelerated in heated cells. Hsp70 has been known to bind with various proteins and corrects folding, thereby preventing degradation of the target proteins Citation[42]. As shown in , NQO1 and Hsp70 were co‐precipitated by anti‐NQO1 antibody or anti‐Hsp70 antibody, demonstrating that the two proteins are strongly associated in the tumour cells before and after heating. Moreover, the expressions of both proteins markedly increased by heating in both A549 cells and MDA‐MB 231(NQO1+) cells ( and ). Furthermore, transfection of cells with siHsp70 RNA suppressed not only the Hsp70 expression but also NQO1 expression before and after heating (). Confocal microscopy () demonstrated the co‐localisation of NQO1 and Hsp70 in the cytosol before heating, and the increased expression of both proteins in the cytosol after heating. Taken together, the observations indicate that the stabilisation of NQO1 by the Hsp70 molecular chaperone may also account for the prolonged increase in production of NQO1 protein after heating.
In summary, the anti‐cancer effects of β‐lap and NQO1 expression are positively related. Heating causes prolonged elevation of NQO1 protein level, thereby increasing the sensitivity of the cells to β‐lap. The prolonged increase in the level of NQO1 protein after heating may be attributed to two distinctive mechanisms. Firstly, heating increases the transcription of NQO1 by increasing the promoter activity mainly at AER cis‐element in the NQO1 gene promoter region. Secondly, degradation of NQO1 protein is decelerated by virtue of Hsp70‐induced stabilisation of NQO1 protein. The results of the present study as well as our previous observation unequivocally demonstrated that heating cancer cells at 41°–42°C causes long‐lasting elevation of NQO1 expression, thereby markedly increasing the response of the cells to the cytotoxic effect of β‐lap. In this aspect, it should be re‐emphasised that the expression of NQO1 in various tumours is already greater than that in adjacent normal tissues Citation[15], Citation[27–31]. These facts may be exploited to selectively target tumours by combining mild heating and β-lap. Experiments are in progress in our laboratory to determine the effect of heating on NQO1 expression in various human tumour cell xenografts and the response of the in vivo tumours to the combined effect of heating and β-lap.
Acknowledgements
This work was supported by grants from the National R&D Programme for Cancer Control, the Ministry of Health & Welfare of the Republic of Korea (0620340-1), National Nuclear Technology Programme (2007) from KOSEF (2009-0062222), the Korea Health 21 R&D Project (A062254), and the National Institutes of Health of the United States (RO1 CA116721).
Declaration of interest: The authors report no conflicts of interest. The authors alone are responsible for the content and writing of the paper.
References
- Begleiter A, Fourie J. Induction of NQO1 in cancer cells. Methods Enzymol 2004; 382: 320–351
- Joseph P, Jaiswal AK. NAD(P)H:quinone oxidoreductase1 (DT diaphorase) specifically prevents the formation of benzo[a]pyrene quinone-DNA adducts generated by cytochrome P4501A1 and P450 reductase. Proc Natl Acad Sci USA 1994; 91: 8413–8417
- Ross D, Siegel D. NAD(P)H:quinone oxidoreductase 1 (NQO1, DT-diaphorase), functions and pharmacogenetics. Methods Enzymol 2004; 382: 115–144
- Talalay P, Dinkova-Kostova AT. Role of nicotinamide quinone oxidoreductase 1 (NQO1) in protection against toxicity of electrophiles and reactive oxygen intermediates. Methods Enzymol 2004; 382: 355–364
- Rauth AM, Goldberg Z, Misra V. DT-diaphorase: Possible roles in cancer chemotherapy and carcinogenesis. Oncol Res 1997; 9: 339–349
- Robertson N, Stratford IJ, Houlbrook S, Carmichael J, Adams GE. The sensitivity of human tumour cells to quinone bioreductive drugs: What role for DT-diaphorase?. Biochem Pharmacol 1992; 44: 409–412
- Begleiter A, Leith MK, Thliveris JA, Digby T. Dietary induction of NQO1 increases the antitumour activity of mitomycin C in human colon tumours in vivo. Br J Cancer 2004; 91: 1624–1631
- Siegel D, Beall H, Senekowitsch C, et al. Bioreductive activation of mitomycin C by DT-diaphorase. Biochemistry 1992; 31: 7879–7885
- Keyes SR, Fracasso PM, Heimbrook DC, et al. Role of NADPH:cytochrome c reductase and DT-diaphorase in the biotransformation of mitomycin C1. Cancer Res 1984; 44: 5638–5643
- Loadman PM, Bibby MC, Phillips RM. Pharmacological approach towards the development of indolequinone bioreductive drugs based on the clinically inactive agent EO9. Br J Pharmacol 2002; 137: 701–709
- Winski SL, Swann E, Hargreaves RH, et al. Relationship between NAD(P)H:quinone oxidoreductase 1 (NQO1) levels in a series of stably transfected cell lines and susceptibility to antitumor quinones. Biochem Pharmacol 2001; 61: 1509–1516
- Fitzsimmons SA, Workman P, Grever M, et al. Reductase enzyme expression across the National Cancer Institute Tumor cell line panel: Correlation with sensitivity to mitomycin C and EO9. J Natl Cancer Inst 1996; 88: 259–269
- Pink JJ, Planchon SM, Tagliarino C, et al. NAD(P)H:Quinone oxidoreductase activity is the principal determinant of beta-lapachone cytotoxicity. J Biol Chem 2000; 275: 5416–5424
- Planchon SM, Pink JJ, Tagliarino C, et al. beta-Lapachone-induced apoptosis in human prostate cancer cells: Involvement of NQO1/xip3. Exp Cell Res 2001; 267: 95–106
- Bey EA, Bentle MS, Reinicke KE, et al. An NQO1- and PARP-1-mediated cell death pathway induced in non-small-cell lung cancer cells by beta-lapachone. Proc Natl Acad Sci USA 2007; 104: 11832–11837
- Cruz FS, Docampo R, Boveris A. Generation of superoxide anions and hydrogen peroxide from beta-lapachone in bacteria. Antimicrob Agents Chemother 1978; 14: 630–633
- Docampo R, Cruz FS, Boveris A, Muniz RP, Esquivel DM. beta-Lapachone enhancement of lipid peroxidation and superoxide anion and hydrogen peroxide formation by sarcoma 180 ascites tumor cells. Biochem Pharmacol 1979; 28: 723–728
- Pardee AB, Li YZ, Li CJ. Cancer therapy with beta-lapachone. Curr Cancer Drug Targets 2002; 2: 27–242
- Li Y, Sun X, LaMont JT, Pardee AB, Li CJ. Selective killing of cancer cells by beta -lapachone: Direct checkpoint activation as a strategy against cancer. Proc Natl Acad Sci USA 2003; 100: 2674–2678
- Tagliarino C, Pink JJ, Dubyak GR, Nieminen AL, Boothman DA. Calcium is a key signaling molecule in beta-lapachone-mediated cell death. J Biol Chem 2001; 276: 19150–19159
- Bentle MS, Reinicke KE, Bey EA, Spitz DR, Boothman DA. Calcium-dependent modulation of poly(ADP‐ribose) polymerase‐1 alters cellular metabolism and DNA repair. J Biol Chem 2006; 281: 33684–33696
- Park HJ, Ahn KJ, Ahn SD, et al. Susceptibility of cancer cells to beta-lapachone is enhanced by ionizing radiation. Int J Radiat Oncol Biol Phys 2005; 61: 212–219
- Suzuki M, Amano M, Choi J, et al. Synergistic effects of radiation and beta‐lapachone in DU-145 human prostate cancer cells in vitro. Radiat Res 2006; 165: 525–531
- Choi EK, Terai K, Ji IM, et al. Upregulation of NAD(P)H:quinone oxidoreductase by radiation potentiates the effect of bioreductive beta-lapachone on cancer cells. Neoplasia 2007; 9: 634–642
- Park HJ, Choi EK, Choi J, et al. Heat-induced up-regulation of NAD(P)H:quinone oxidoreductase potentiates anticancer effects of beta-lapachone. Clin Cancer Res 2005; 11: 8866−8871
- Song CW, Chae JJ, Choi EK, et al. Anti-cancer effect of bio-reductive drug beta-lapachon is enhanced by activating NQO1 with heat shock. Int J Hyperthermia 2008; 24: 161–169
- Siegel D, Franklin WA, Ross D. Immunohistochemical detection of NAD(P)H:quinone oxidoreductase in human lung and lung tumors. Clin Cancer Res 1998; 4: 2065–2070
- Belinsky M, Jaiswal AK. NAD(P)H:quinone oxidoreductase1 (DT-diaphorase) expression in normal and tumor tissues. Cancer Metastasis Rev 1993; 12: 103–117
- Cresteil T, Jaiswal AK. High levels of expression of the NAD(P)H:quinone oxidoreductase (NQO1) gene in tumor cells compared to normal cells of the same origin. Biochem Pharmacol 1991; 42: 1021–1027
- Beall HD, Murphy AM, Siegel D, et al. Nicotinamide adenine dinucleotide (phosphate): quinone oxidoreductase (DT-diaphorase) as a target for bioreductive antitumor quinones: Quinone cytotoxicity and selectivity in human lung and breast cancer cell lines. Mol Pharmacol 1995; 48: 499–504
- Kepa JK, Ross D. Differential expression of the antioxidant response element within the hNQO1 promoter in NSCLC versus SCLC. Biochem Biophys Res Commun 2003; 311: 446–453
- Boothman DA, Trask DK, Pardee AB. Inhibition of potentially lethal DNA damage repair in human tumor cells by beta-lapachone, an activator of topoisomerase I. Cancer Res 1989; 49: 605–612
- Bentle MS, Reinicke KE, Dong Y, Bey EA, Boothman DA. Nonhomologous end joining is essential for cellular resistance to the novel antitumor agent, beta-lapachone. Cancer Res 2007; 67: 6936–6945
- Khong HT, Dreisbach L, Kindler HL, et al. A phase 2 study of ARQ 501 in combination with gemcitabine in adult patients with treatment naïve, unresectable pancreatic adenocarcinoma. ASCO Annual Meeting Proceedings Part I. J Clin Oncol 2007; 25: 15017
- Blanco E, Bey EA, Dong Y, et al. Beta-lapachone-containing PEG-PLA polymer micelles as novel nanotherapeutics against NQO1-overexpressing tumor cells. J Control Release 2007; 122: 365–374
- Dong Y, Chin SF, Blanco E, et al. Intratumoral delivery of beta-lapachone via polymer implants for prostate cancer therapy. Clin Cancer Res 2009; 15: 131–139
- Long DJ, II, Gaikwad A, Multani A, et al. Disruption of the NAD(P)H:quinone oxidoreductase 1 (NQO1) gene in mice causes myelogenous hyperplasia. Cancer Res 2002; 62: 3030–3036
- Dhakshinamoorthy S, Jaiswal AK. Functional characterization and role of INrf2 in antioxidant response element-mediated expression and antioxidant induction of NAD(P)H:quinone oxidoreductase1 gene. Oncogene 2001; 20: 3906–3917
- Nioi P, Hayes JD. Contribution of NAD(P)H:quinone oxidoreductase 1 to protection against carcinogenesis, and regulation of its gene by the Nrf2 basic-region leucine zipper and the arylhydrocarbon receptor basic helix-loop-helix transcription factors. Mutat Res 2004; 555: 149–171
- Jaiswal AK. Nrf2 signaling in coordinated activation of antioxidant gene expression. Free Radic Biol Med 2004; 36: 1199–1207
- Xie T, Belinsky M, Xu Y, Jaiswal AK. ARE- and TRE-mediated regulation of gene expression. Response to xenobiotics and antioxidants. J Biol Chem 1995; 270: 6894–6900
- Anwar A, Siegel D, Kepa JK, Ross D. Interaction of the molecular chaperone Hsp70 with human NAD(P)H:quinone oxidoreductase 1. J Biol Chem 2002; 277: 14060–14067
- Elegbeleye OO. Cavernous sinus thrombosis in Nigerians. West Indian Med J 1975; 24: 94–96
- Ma Q, Kinneer K, Bi Y, Chan JY, Kan YW. Induction of murine NAD(P)H:quinone oxidoreductase by 2,3,7,8-tetrachlorodibenzo-p-dioxin requires the CNC (cap ‘n’ collar) basic leucine zipper transcription factor Nrf2 (nuclear factor erythroid 2-related factor 2): cross-interaction between AhR (aryl hydrocarbon receptor) and Nrf2 signal transduction. Biochem J 2004; 377: 205–213
- Reddy AJ, Christie JD, Aplenc R, et al. Association of Human Nad(P)H:Quinone Oxidoreductase 1 (Nqo1) Polymorphism with Development of Acute Lung Injury. J Cell Mol Med 2008
- Tirumalai R, Rajesh Kumar T, Mai KH, Biswal S. Acrolein causes transcriptional induction of phase II genes by activation of Nrf2 in human lung type II epithelial (A549) cells. Toxicol Lett 2002; 132: 27–36
- Dhakshinamoorthy S, Jaiswal AK. c-Maf negatively regulates ARE-mediated detoxifying enzyme genes expression and anti-oxidant induction. Oncogene 2002; 21: 5301–5312
- Yang XY, Yang TT, Schubert W, Factor SM, Chow CW. Dosage-dependent transcriptional regulation by the calcineurin/NFAT signaling in developing myocardium transition. Dev Biol 2007; 303: 825–837
- Kolesar JM, Rizzo JD, Kuhn JG. Quantitative analysis of NQO1 gene expression by RT-PCR and CE-LIF. J Capillary Electrophor 1995; 2: 287–290
- Fritsche E, Schafer C, Calles C, et al. Lightening up the UV response by identification of the arylhydrocarbon receptor as a cytoplasmatic target for ultraviolet B radiation. Proc Natl Acad Sci USA 2007; 104: 8851–8856
- Pontrelli P, Ursi M, Ranieri E, et al. CD40L proinflammatory and profibrotic effects on proximal tubular epithelial cells: Role of NF-kappaB and lyn. J Am Soc Nephrol 2006; 17: 627–636
- Kolesar JM, Rizzo JD, Kuhn JG. Quantitative analysis of NQO1 gene expression by RT-PCR and CE-LIF. J Capillary Electrophor 1995; 2: 287–290
- Haemi Lee, Hee-Jin Kwak, Il-taeg Cho, Seok Hee Park, Chang-Hun Lee. S1219 residue of 53BP1 is phosphorylated by ATM kinase upon DNA damage and required for proper execution of DNA damage response. Biochem Biophys Res Comm 2009; 378: 32–36
- Jin Hee Kim, Tae Heung Kang, Kyung Hee Noh, et al. Enhancement of dendritic cell-based vaccine potency by anti-apoptotic siRNAs targeting key pro-apoptotic proteins in cytotoxic CD8+ T cell-mediated cell death. Immunol Lett 2009; 122: 58–67
- Korashy HM, El-Kadi AO. Transcriptional regulation of the NAD(P)H:quinone oxidoreductase 1 and glutathione S-transferase ya genes by mercury, lead, and copper. Drug Metab Dispos 2006; 34: 152–165