Abstract
Approximately 30% of early stage lung cancer patients are not surgical candidates due to medical co-morbidities, poor cardiopulmonary function and advanced age. These patients are traditionally offered chemotherapy and radiation, which have shown relatively modest improvements in mortality. For over a decade, percutaneous image-guided ablation has emerged as a safe, cost-effective, minimally invasive treatment alternative for patients who would otherwise not qualify for surgery. Although radiofrequency ablation (RFA) is currently the most extensively studied and widely utilised technique in the treatment of lung malignancies, there is a growing body of evidence that microwave ablation (MWA) has several unique benefits over RFA and cryoablation in the lung. This article reviews our institution’s clinical experiences in the treatment of lung malignancies with MWA including patient selection, procedural technique, imaging follow-up, treatment outcomes and comparison of ablation techniques.
Introduction
Lung cancer has recently become the leading cause of death for men and women in the USA, with an estimated incidence of 224,390 diagnoses and 158,080 deaths in 2016 [Citation1]. The lungs are also a common site for metastasis for a wide range of common primary malignancies including renal cell carcinoma, colorectal carcinoma and breast carcinoma. Although surgical lobectomy remains the standard of care and the best treatment option for improving chances of disease-free survival, only about 30% of patients are considered surgical candidates due to medical co-morbidities, poor cardiopulmonary function and advanced age [Citation2]. Patients deemed to be poor surgical candidates are traditionally offered chemotherapy and radiation utilising external beam or stereotactic body radiotherapy (SBRT). These therapies have only shown modest improvements in mortality with an average 3-year survival rate of 56.6% in patients with stage I lung cancer treated with SBRT [Citation3].
For over a decade percutaneous image-guided ablation has emerged as a safe, cost-effective, minimally invasive treatment alternative for patients who would otherwise not qualify for surgery. Ablation therapy utilises either thermal or electrical energy to allow for direct and controlled destruction of solid neoplasms in many organ systems including the lung. These therapies can be performed under conscious sedation and in an outpatient setting, which allows for quicker discharge and recovery times as compared to surgical resection [Citation4]. Additionally, there is evidence that tumour ablation has additive benefits when performed in conjunction with chemotherapy and/or external beam radiation due to the effects of cytoreduction [Citation5,Citation6]. Although radiofrequency ablation (RFA) is currently the most extensively studied and utilised technique in the treatment of lung neoplasms, this article will focus on our clinical experiences and outcomes in patients treated with microwave ablation (MWA). Over the past several years there has been a growing body of evidence that MWA has several unique benefits over RFA and cryoablation in the treatment of pulmonary malignancy, which we will discuss in further detail.
Background
MWA is a relatively new ablation technique, which utilises electromagnetic waves in the microwave energy spectrum (300 MHz to 300 GHz) to produce tissue-heating effects. The basic microwave system consists of three main components including the generator, the power distribution system and antennas. Depending on the manufacturer, the generator frequencies are either 915 MHz or 2.45 GHz, which is distributed via coaxial transmission cable to a microwave antenna. These high frequency electromagnetic waves induce rapid rotation of water molecules, which are forced to continuously realign with the alternating electric field due to their polarity. The kinetic energy of these water molecules causes frictional heat that is then transmitted to adjacent tissues. Temperature is actively measured during the procedure via thermocouple device or internal vendor-specific antenna designs as a surrogate measure of this molecular kinetic energy. The targeted lesion must be heated in excess of 60 °C in order to achieve immediate coagulation necrosis and cell death [Citation7].
There are currently seven MWA systems commercially available in the USA. The systems use either a 915-MHz generator (Evident Medtronic, Boulder, CO, MicrothermX Perseon Medical, Salt Lake City, UT, Avecure, Medwaves, San Diego, CA) or a 2450-MHz generator (Certus 140, Neuwave, Madison, WI, Amica, Hospital Service, Rome, Italy, Acculis MTA, Angiodynamics, Latham, NY, or Emprint Medtronic). The MW antennae used are straight applicators with active tips ranging in lengths from 0.6 to 4.0 cm. Six of the seven available systems feature internal cooling mechanisms which serve to increase power handling through a smaller diameter antennae while reducing the risk of skin burns due to shaft heating. These active cooling components have enabled the delivery of higher powers for longer times, and in turn, production of larger ablation zones [Citation8]. One study comparing cooled with non-cooled shaft antennas in a cohort of 1136 patients showed that the use of the cooled-shaft antenna led to fewer treatment sessions and fewer major procedural complications [Citation9].
Patient selection
The goals of thermal ablation of lung malignancies are to improve disease-free survival in patients who are otherwise not surgical candidates, and to provide palliation of tumour-related symptoms in patients with more advanced disease. The first goal is most optimally achieved in patients with favourably located, oligonodular, early stage (1A or 1B) lung cancer without hilar, mediastinal nodal or extrathoracic involvement () [Citation10]. At present there are no guidelines regarding the maximum number of pulmonary lesions that can be treated. Ablation can also be utilised for management of local recurrence within a previously radiated site [Citation11]. However, patients who have had prior radiation may be at increased risk theoretically for larger ablation zones and possible vascular injury due to radiation-associated vasculopathy.
Figure 1. An 80-year-old woman with cardiovascular disease (previous coronary artery bypass graft surgery) and severe emphysema from 40 packs per year smoking history developed an early stage non-small cell lung cancer and was referred for tumour ablation due to her high operative risk. (A) Axial CT image in lung windows shows the 1.4 cm left upper lobe NSCLC (arrow). (B) Axial fluorodeoxyglucose PET/CT fusion image shows intense standard uptake value (SUV) of 6.8 (arrow) without evidence of regional or distant spread (Stage IA). (C) Axial image during the microwave ablation shows satisfactory placement of the antenna within the centre of the mass parallel to the long axis (arrow) and (D) after 10 min of heating with 65 W a circumferential ’ground glass halo’ appears indicative of thermal damage to the mass and adjacent normal lung; (E) 1-month and (F) 6-month follow-up CT scans show the baseline thermal scar with antenna tract still visible (arrow) and expected involutional changes at 6 months.
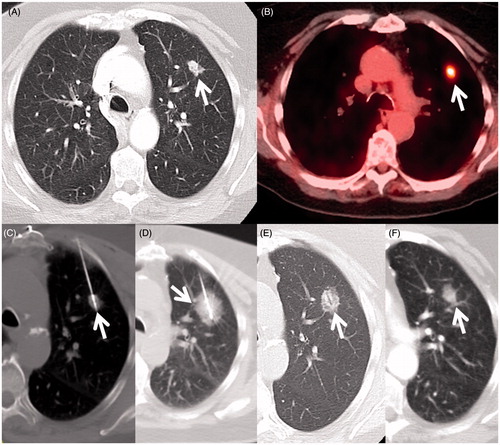
Patients referred for lung tumour ablation often have limited pulmonary function with minimal reserve. However, there are generally no lower limits on forced expiratory volume in 1 s (FEV1) or diffusion capacity of lungs for carbon monoxide (DLCO). Patients must otherwise be stable and healthy enough to tolerate a CT-guided intervention, which involves lying flat on a CT gantry for a period of time. Contraindications include severe underlying interstitial lung disease such as pulmonary fibrosis and severe emphysema given that exacerbation can result in further compromise of lung function leading to respiratory failure and possibly death [Citation12]. Typically, patients with life expectancies of less than 1 year are not considered good candidates for ablation [Citation13].
Procedure
The work up for microwave ablation is generally the same as for other methods of percutaneous tumour ablation. This requires a detailed pre-procedural medical evaluation which involves taking a history, performing physical examination, and obtaining appropriate laboratory studies. It is important for the specialist to review the pre-procedural imaging with the patient to aid in discussion of the treatment plan and to set expectations. Patients must be counselled regarding possible risks including post-ablation syndrome, which is an inflammatory response caused by circulating tumour necrosis factor and associated with fever, malaise, and anorexia. Additional risks include mild to moderate pain, fevers, haemorrhage, haemoptysis, pneumothorax (potentially requiring chest tube and hospital admission), broncho-pleural fistula (potentially requiring treatment with chest tube suction, Heimlich valves, chemical or mechanical pleurodesis, surgery, or intrabronchial valves [Citation14]), acute respiratory distress syndrome, reactive pleural effusion (usually self-limited), damage to other structures near the ablation zone, infection and death. However, it should be stressed to patients that the likelihood of a severe complication is very rare.
Patients are instructed to fast overnight prior to the ablation in order to reduce the risk of sedation-induced nausea and the potential for aspiration. Patients should typically continue their current medications the morning of the procedure including antihypertensives and other cardiac medications, which can be taken with a small amount of water. Insulin-dependent diabetics are advised to administer only half of their typical morning dose of insulin. Caution should also be taken in patients with implantable cardiac devices such as pacemakers or defibrillators as these devices are susceptible to electrical interference from the typical energy spectrums used for both MWA and RFA [Citation15]. Consultation with the patient’s cardiac electrophysiologist is recommended before the procedure in order to set the pacemaker to automatic pacing (by placing a magnet over the top) and turning off defibrillation mode during the procedure. Post-procedurally, these devices should be interrogated and re-programmed as necessary. External pacing leads and an external defibrillator should be present for prompt treatment of clinically significant arrhythmia if one should arise during the ablation.
On the day of the procedure we perform another focused physical examination and an intravenous line is placed. Patients are brought into the CT-fluoroscopy suite and prepped by technical staff. Most patients are able to tolerate thermal ablation with intravenous conscious sedation utilising midazolam and fentanyl. However, general anaesthesia can also be used in the pediatric population or under appropriate clinical circumstances. Patients are monitored throughout the procedure with monitoring of vital signs, electrocardiography, and continuous pulse oximetry. Initial cross-sectional scout CT images are obtained, from which the skin entry site(s) are determined. Horizontal and vertical laser lights emitted from the CT gantry correspond to x- and y-axis points obtained from a grid on the computer screen. These lines are used to extrapolate correct entry sites on the patient’s skin.
The skin surface is prepped and draped in a sterile fashion before injection of local buffered 1% lidocaine anaesthesia, both intradermally and to the level of the pleura. Approximately 20–30 mL of lidocaine is instilled in the extrapleural location to minimise pain when the applicator(s) are inserted as well as to protect the chest wall for lesions located close to the parietal pleura (). CT-fluoroscopy is then initiated and images are obtained with a spinal needle in place to determine the appropriate trajectory. A scalpel blade is used to make a 1-cm deep skin incision at the entry site. There are several other techniques that have been published regarding methods to reduce pain and complications when performing thoracic tumour ablation including performing a paravertebral nerve block with a long-acting local anaesthetic [Citation16]. Additional techniques including hydrodissection and artificial pneumothorax can be helpful for minimising risk while treating tumours near sensitive structures such as the phrenic nerve, major blood vessels, or cartilage. Solomon et al. [Citation17] described the use of a needle with a spring-loaded blunt-tipped obturator as a means to introduce air into the pleural space, thus creating a protective pneumothorax to displace tumour tissue from adjacent vulnerable mediastinal and chest wall structures [Citation17]. However, treatment of nodules abutting the heart, oesophagus or major airways that cannot be separated with techniques such as hydrodissection or artificial pneumothorax would be contraindicated.
Figure 2. A 70-year-old woman with a 45 packs per year smoking history and previous lung cancer surgery on the left had a mass in the right lung discovered on chest X-ray. (A) Axial CT scan shows a 2.7-cm elliptical mass with central cavitation abutting the visceral pleural surface (arrow). (B) Axial FDG PET image shows intense uptake with standard uptake value of 10.9 (arrow) with no regional or distant disease (Stage IB). Biopsy confirmed squamous cell carcinoma and she was referred for ablation due to her high operative risk. (C) Axial image during the microwave ablation shows satisfactory placement of the antenna within the centre of the mass parallel to the long axis (arrow) and (D) after 10 min of heating with 65 W a circumferential ground glass halo appears; (E) 10- and (F) 14-month follow-up CT scans show a small growing nodule adjacent to the ablation zone consistent with recurrence (arrow). The patient returned for retreatment. (G) Axial CT image shows MW antenna in the recurrence. Extrapleural lidocaine was used to protect the chest wall (small arrows) (H) Axial CT image 30 months after initial ablation shows an air filled cavity and no recurrence at the treatment site (arrow).
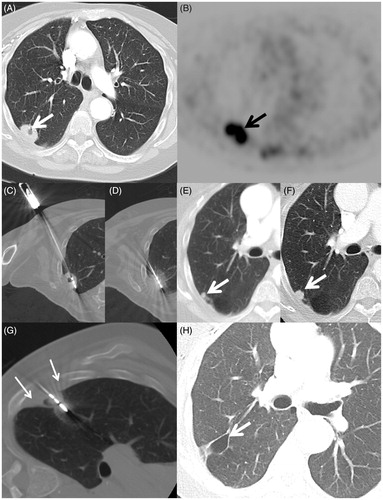
Under CT-fluoroscopic guidance, the exposed non-insulated portion of the microwave antenna(e) are placed directly through the skin and pleura into the distal margin of the target lesion with the intended goal of creating a zone of tissue necrosis that encompasses both the tumour and a margin of normal parenchyma immediately surrounding it. This zone of tissue necrosis is estimated based upon the immediate development of a peripheral ground glass halo surrounding the lesion. However, it is important to recognise that the ground glass halo overestimates the zone of coagulative necrosis by approximately 4 mm [Citation18]. Ideally, a 1-cm ground glass halo should be visualised for the treatment of pulmonary metastases under 2 cm in size [Citation19]. For primary lung cancer a ground glass halo of 1.5 cm has been reported to reduce local recurrence in patients treated with radiofrequency ablation [Citation19]. For tumours larger than 3 cm, we generally recommend using between 2–3 antennae placed approximately 1–2 cm apart to ensure an adequate zone of thermo-coagulation.
However, the higher temperatures generated during microwave ablation, especially with higher frequency probes (i.e. 2450 MHz), may theoretically lead to an increased bronchopleural fistula rate relative to radiofrequency ablation. Therefore, an indirect approach is generally preferred, leaving at least 2 cm of normal aerated lung between the lesion and the visceral pleural surface [Citation20]. Along these same lines, tract ablation of the lung with either modality is ill advised given the risk of bronchopleural fistula formation. Saline can also be injected into cavitary lung nodules to theoretically improve treatment with microwave ablation, a technique we have successfully used at our institution. However, at present there is no data to support this practice, nor large published studies comparing the efficacy of microwave ablation for the treatment of solid versus cavitary neoplasms.
During the ablation, intratumoural temperatures are measured with either a separately placed thermocouple device or via internal probe (depending on the specific manufacturer design) with the goal of achieving temperatures lethal to target tissues (60–100 °C) [Citation7]. Unit power output and heating times also vary by manufacturer. As opposed to RFA, MWA does not require an electrical current, thereby eliminating the need for grounding pads. Overall, the expected size and shape of the ablation zone depends on the active tip length, number and positioning of antennae as well as the presence of ‘heat sinks’ caused by nearby structures such as blood vessels that dissipate heat produced at the antennas active tip. Our experiences have shown that nodules abutting major vessels (>4 mm) are unlikely to be completely ablated due to thermal sinks and these may be better treated with external beam radiotherapy techniques.
After the target tumour is treated the ablation probe is removed and CT fluoroscopic images are obtained to evaluate technical success and to rule out the presence of a pneumothorax. Patients are then observed for approximately 2–4 h afterwards with a repeat chest radiograph obtained prior to discharge to confirm the absence of pneumothorax. If and when they occur, most pneumothoraces are generally small and asymptomatic and can be monitored with serial chest radiography. Large and/or symptomatic pneumothoraces require chest tube placement and wall suction to evacuate the air leak. Repeat chest radiographs should be used to confirm resolution of the air leak prior to discharge. If a persistent air leak is identified, those patients can be treated with a Heimlich valve prior to discharge with instructions to follow up in 24 h for a repeat chest radiograph. Patients who are otherwise unable to manage a chest tube as an outpatient or those with significant levels of pain are admitted.
Follow-up
It is important to understand the expected evolution of imaging findings in patients treated with microwave ablation therapy in order to accurately assess the efficacy of therapy and for the early detection of local and/or distant recurrence. However, at present there is currently no established consensus on which imaging modality or time interval post-ablation best detects local recurrence or treatment failure. Many authors have recommended initially following patients with contrast-enhanced CT followed by alternating intervals of either contrast-enhanced CT or positron emission tomography (PET)/CT [Citation21]. At our institution we obtain a 1-month post-ablation contrast enhanced CT as a baseline and then follow every 3 months for 2 years and then every 6 months. Fludeoxyglucose (FDG)-PET/CT is used when imaging features suggest new disease at the treatment site or elsewhere. Yet regardless of modality, the use of extracellular contrast agents are crucial in differentiating between areas of non-enhancement compatible with tumour necrosis and enhancement within viable tumour cells [Citation22]. Additionally, most of the available literature focuses on the imaging appearance of tumours following radiofrequency ablation, as it is the more established technique. However, given the similar pathological end result of coagulation necrosis, tumours treated with microwave ablation can be expected to follow similar imaging patterns.
CT imaging performed immediately following microwave lung ablation will demonstrate a hazy rim of ground-glass opacity within and around the ablation zone, also penetrated by well-defined antenna tracks. On the initial follow-up scan, complete or near complete resolution of enhancement within the ablation zone, presumably due to disruption of the local microcirculation, has been considered to be indicative of successful MWA [Citation23]. Localised pleural thickening is another common post-treatment finding (34%) in tumours abutting the visceral pleura [Citation23]. Nodular or central enhancement >10 mm and/or >15 HU is concerning for recurrent or incompletely ablated disease () [Citation24]. However, it is important to note that a benign thin smooth region of peripheral enhancement beyond the ablation zone can persist up to 6 months after treatment [Citation25]. FDG PET/CT is also useful in the follow-up evaluation with a decrease or complete absence of PET activity (photopenia) suggestive of necrosis with no residual tumour. [Citation26] Akeboshi et al. demonstrated that PET was more sensitive than contrast-enhanced CT to detect early tumour progression [Citation27].
At the following 1-, 3- and 6-month intervals, the ablation zone can be expected to increase in size as a result of thermal changes to the surrounding lung parenchyma. This initial 6-month period of growth is then followed by consolidation due to involution and parenchymal scar formation causing a gradual reduction in lesion diameter ( and ) [Citation25]. Cavitary changes prior to ablation zone involution have also been described as a positive prognostic sign with a significant inverse relationship to cancer-specific mortality in the first 2 months [Citation23].
Clinical outcomes
Thermal ablation should be considered as a viable treatment option for patients with early stage primary or secondary lung cancers who are not surgical candidates or for patients in whom palliation of tumour-related symptoms is the intent. The goals of thermal ablation of thoracic malignancies are threefold: 1) clinicians should aim to ablate the entire tumour and a margin of normal parenchyma surrounding it, 2) they should avoid injury to critical structures, and 3) they should create a large ablation area quickly. Data regarding outcomes of thermal ablation are diverse because study groups are heterogeneous and follow-up periods and reporting standards vary. Additionally, aside from case series there are no randomised controlled trials comparing thermal ablation with various other treatment modalities or to surgical resection [Citation28].
Although we have already touched on several benefits of microwave ablation therapy versus radiofrequency ablation in treating lung tumours, the major advantage of RFA over MWA is experience. RF technology has been used for ablation of pulmonary malignancies for over a decade and there are numerous studies in existence, which have validated the safety and efficacy of this therapy. One of the largest prospective trials to date included 566 patients treated with RFA for primary and secondary pulmonary malignancies and demonstrated an overall survival of 62 months. Kaplan–Meier survival estimates at 1, 2, 3, 4, and 5 years were 92.4%, 79.4%, 67.7%, 58.9%, and 51.5%, respectively, thus indicating a survival benefit with such treatment [Citation29]. The only prospective multicentre study enrolled 54 stage IA NSCLC patients and showed 86.3% and 69.8% overall survival at 1 and 2 years respectively. This survival benefit improved to 83% at 2 years in patients with tumour size <2 cm [Citation30]. Given the amount of evidence, the American College of Chest Physicians included RF ablation in their most recent recommendations for tumours <3 cm [Citation31].
However, a major theoretical limitation of RF ablation in the treatment of lung tumours is the insulating effects of aerated lung tissues. This natural insulation concentrates thermal energy at the site of the lesion with local air exchange and vascular flow carrying heat energy away from the target ablation zone [Citation32]. Although this ‘heat sink’ phenomenon can have a protective effect on adjacent vulnerable non-target structures it also limits the effectiveness of RF heating [Citation33]. In addition, RF ablation is limited due to tissue char effects, which impede thermal conductance and can limit thermal necrosis near the periphery of the lesion [Citation34,Citation35]. In contrast, microwave propagation is not insulated by air or the limited water content of lung parenchyma, thus allowing for broader energy deposition capable of more rapidly producing higher intratumoural temperatures resulting in a larger zone of active heating [Citation34,Citation36].
Microwave ablation systems also allow for the use of multiple applicators and can provide more effective heating of cystic masses, less char effect, and elimination of grounding pad injuries associated with RFA () [Citation37]. These theoretical advantages could conceivably improve ablation margins, thus reducing the chance of local recurrence. Microwave ablation is also associated with less procedural pain, perhaps from lack of electrical nerve stimulation associated with RFA [Citation37]. However, as previously mentioned, the higher temperatures generated during MWA, especially with higher frequency probes (i.e. 2450 MHz) may theoretically lead to an increased bronchopleural fistula rate relative to RFA [Citation20]. Therefore, leaving an unablated tract at least 2 cm from visceral pleura is preferred [Citation20].
Figure 3. An 84-year-old woman with history of severe COPD from extensive cigarette smoking on oxygen therapy presented with severe left chest wall pain. (A) Axial CT scan shows a large necrotic mass with extension into chest wall (arrow) which was confirmed to be squamous cell carcinoma on subsequent biopsy. (B) Coronal image from staging FDG PET/CT shows extensive uptake with central necrosis without evidence of regional or distant spread (Stage 2B). Based on size and necrosis, a combination treatment with MWA and radiotherapy was planned. (C, D) Axial CT-fluoroscopic images show two antennas (arrows) placed superiorly and inferiorly within the mass close to the chest wall. Note the placement of the cold saline bag placed on the skin to reduce heating during the MWA. The patient’s pain was markedly relieved within 24 h of the MWA. (E) Image from subsequent radiation treatment plan targeting the mass. (F) Axial CT follow-up images at 6 months and (G) 2 years after treatment shows central necrosis (white arrow) and eventual shrinkage and cavitation (black arrow). The patient died of her respiratory disease, but not from her lung cancer.
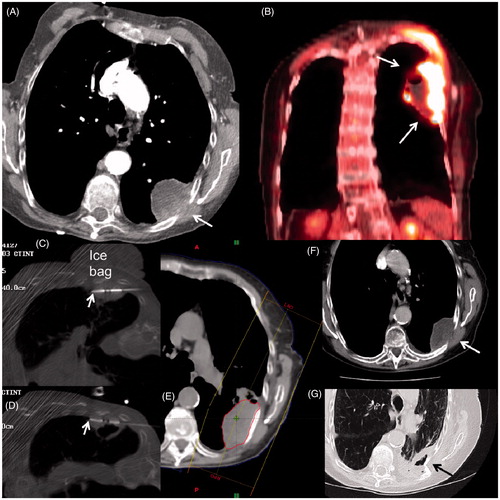
Several large single-institution studies have been published demonstrating MWA’s effectiveness in the treatment of thoracic malignancies. For instance, a study by Belforie et al. demonstrated cancer-specific mortalities of 69%, 54% and 49% at 1, 2 and 3 years in 56 lung tumour patients treated with MWA [Citation38]. A more recent study evaluating MWA in 87 patients from two centres demonstrated an overall survival of 58.1% at 5 years and disease-free survival at 5 years of 27.9% [Citation39]. In another retrospective analysis of 82 lung parenchymal lesions, MWA resulted in a 1-year local control rate of 67% with mean time to recurrence of 16.2 months. The survival rates were 83%, 73%, 61% at 1, 2, and 3 years, respectively [Citation23]. Another prospective study reported a 73.1% complete ablation rate with MWA in 130 pulmonary metastatic lesions with minimal procedural complications. The 1- and 2-year survival rates were 91.3% and 75%, respectively [Citation40].
Cryoablation is also associated with many of the same advantages as MWA over RFA in that it can produce larger tumour ablation volumes, allow for the use of multiple applicators, create less procedural pain due to local anaesthetic effects of freezing, and does not require grounding pads thus eliminating the possibility for associated grounding pad injuries. In addition, compared with heat-based thermal ablation therapies, cryoablation allows for the preservation of cellular architecture in frozen tissues [Citation41,Citation42]. Major theoretical disadvantages to this technique include longer procedural times required in the freeze–thaw–freeze cycle with an increased complication rate especially seen with bleeding requiring possible intervention. Also additional probes are often needed to attain similar coverage as compared to MWA thus posing another potential source of complications [Citation41,Citation43].
Wang et al. reported their experience and safety profile following cryoablation treatment of 187 patients with peripheral lung masses. They were able to achieve 99% coverage in peripheral masses <4 cm with tumour size and location being the most significant predictors of adequate ablation zone coverage [Citation41]. More recently, Yashiro et al. reported treatment effectiveness rates of 92%, 83.4%, and 83.4% at 1, 2, and 3 years respectively in 210 smaller tumours (<3 cm) in 71 patients treated with cryoablation. Local progression-free rates in this study were 80.4%, 69% and 67.7% at 1, 2, and 3 years respectively. They cited risk factors for local progression as tumours larger than [Citation44] 2 cm in diameter and the presence of a vessel at least 3 mm in diameter within 3 mm of the target lesion [Citation44]. Another study treated 47 non-small cell lung cancer (NSCLC) tumours in 45 patients with a 5-year survival rate of 67.8% ± 15.3%, with results similar to survival benefits seen in RFA [Citation45]. Currently, the only prospective multicentre trial which included sites in both the USA and Europe demonstrated a 1-year local control rate of 94.6% in 60 treated metastatic lung lesions in 40 patients with a mean tumour size of 1.5 cm [Citation46].
In comparison to more traditional therapies, the reported 5-year survival for stage I NSCLC following surgical lobectomy is 60–80% and with external beam radiation is up to 40% [Citation47]. A recent meta-analysis demonstrated average 3- and 5-year survival rates of 56.6% and 41.2% in patients with stage I NSCLC treated with SBRT [Citation3]. The overall survival benefit in older patients with early stage NSCLC treated with thermal ablation as compared to sublobar resection showed no significant difference at a significantly lower cost up to 12 months post treatment [Citation48,Citation49]. This advantage was most evident in outpatient procedures, with the median difference in treatment-related cost of US$16,105 [49]. However, there were also significant cost savings reported when performed during inpatient stays as well due to shorter length of hospital stays [Citation45]. Thermal ablation also has a relatively insignificant effect on pulmonary function, can be performed regardless of prior local treatment, and if necessary utilised repeatedly in the treatment of locally recurrent disease [Citation50–52].
There is also evidence suggesting that thermal ablation has additive benefits when performed in conjunction with chemotherapy and/or external beam radiation due to the effects of cytoreduction [Citation5,Citation6,Citation53,Citation54]. However, at present further investigation into the potential synergistic effects between thermal ablation and external radiation is still ongoing.
Conclusion
Microwave ablation is an exciting and relatively new technique for the treatment of thoracic malignancies in patients who are poor surgical candidates or in those seeking palliation of tumour-related symptoms. There are also numerous theoretical advantages to the use of microwave ablation versus radiofrequency and cryoablation in the lung. The main benefits of MWA in comparison to RF lung tumour ablation include larger ablation zones, quicker heating times, higher intralesional temperatures, less procedural pain, and less susceptibility to both insulating effects intrinsic to lung tissue and those associated with tissue charring. However, given the higher temperatures associated with microwave ablation, planning for optimal antenna trajectory is critical to avoid potential risks of persistent air leak and bronchopleural fistula. Additional benefits seen both with MWA and cryoablation over RF ablation include the ability to use multiple probes within the same lesion and a theoretical safety advantage due to the lack of need for a grounding pad. However, the major limitations associated with cryoablation include longer required treatment times due to the freeze–thaw–freeze mechanism and the frequent need for a higher number of probes to ensure appropriate treatment margins. These factors increase the theoretical risk of pulmonary complications requiring intervention including bleeding.
Although surgical resection remains the standard of care for patients healthy enough to undergo an operation, there are a substantial number of medically inoperable patients for whom conventional therapies have shown relatively modest survival benefits. As the technology continues to advance, ablation therapies have shown their benefits not only in safety and effectiveness but also in cost savings. There is also a growing body of evidence that supports the possible adjunctive role for thermal ablation with other conventional treatment modalities including a possible synergistic effect with radiation therapy. Going forward it will be important for radiologists and referring clinicians alike to understand the relative advantages and disadvantages of each ablation modality in various organ systems, including the unique benefits of MWA in treating lung tumours. At present, further research is needed to elucidate relative safety and survivability benefits not only between ablation modalities but also conventional non-operative therapies.
Disclosure statement
D.D. received a research grant from NeuWave Medical Inc., is a BSD Medical Corporation stockholder, is on the Board of Directors of BSD Medical Corporation, and is a speaker for BSD Medical Corporation at educational symposia. The authors alone are responsible for the content and writing of the paper.
References
- American Cancer Society. Key statistics for lung cancer. 2016. Available from http://www.cancer.org/cancer/lungcancer-non-smallcell/detailedguide/non-small-cell-lung-cancer-key-statistics (accessed 23 February 2016).
- Mazzone P. Preoperative evaluation of the lung resection candidate. Cleveland Clin J Med 2012;79:eS17–22.
- Zheng X, Schipper M, Kidwell K, Lin J, Reddy R, Ren Y, et al. Survival outcome after stereotactic body radiation therapy and surgery for stage I non-small cell lung cancer: A meta-analysis. Int J Radiat Oncol 2014;90:603–11.
- Jain SK, Dupuy DE, Cardarelli GA, Zheng Z, DiPetrillo TA. Percutaneous radiofrequency ablation of pulmonary malignancies: combined treatment with brachytherapy. Am J Roentgenol 2003;181:711–15.
- Grieco CA, Simon CJ, Mayo-Smith WW, DiPetrillo TA, Ready NE, Dupuy DE. Percutaneous image-guided thermal ablation and radiation therapy: outcomes of combined treatment for 41 patients with inoperable stage I/II non-small-cell lung cancer. J Vasc Interv Radiol 2006;17:1117–24.
- Dupuy DE, DiPetrillo T, Gandhi S, Ready N, Ng T, Donat W, Mayo-Smith WW. Radiofrequency ablation followed by conventional radiotherapy for medically inoperable stage I non-small cell lung cancer. Chest 2006;129:738–45.
- McTaggart RA, Dupuy DE, Dipetrillo T. Image guided ablation in the thorax. In: Geschwind JF H, Soulen MC, eds. Interventional oncology: Principles and practice. New York: Cambridge University Press, 2008, pp. 440–74.
- Ward RC, Healey TT, Dupuy DE. Microwave ablation devices for interventional oncology. Expert Rev Med Devices 2013;10:225–38.
- Liang P, Wang Y, Yu X, Dong B. Malignant liver tumors: Treatment with percutaneous microwave ablation – complications among cohort of 1136 patients. Radiology 2009;251:933–40.
- Suh R, Reckamp K, Zeidler M, Cameron R. Radiofrequency ablation in lung cancer: promising results in safety and efficacy. Oncology (Williston Park, NY). 2005;19(11Suppl4):12–21.
- Leung VA, DiPetrillo TA, Dupuy DE. Image-guided tumor ablation for the treatment of recurrent non-small cell lung cancer within the radiation field. Eur J Radiol 2011;80:e491–9.
- Dupuy DE, Mayo-Smith WW, Abbott GF, DiPetrillo T. Clinical applications of radio-frequency tumor ablation in the thorax 1. Radiographics 2002;22:S259–S69.
- Dupuy DE. Image-guided thermal ablation of lung malignancies. Radiology 2011;260:633–55.
- Alexander ES, Healey TT, Martin DW, Dupuy DE. Use of endobronchial valves for the treatment of bronchopleural fistulas after thermal ablation of lung neoplasms. J Vasc Interv Radiol 2012;23:1236–40.
- Skonieczki BD, Wells C, Wasser EJ, Dupuy DE. Radiofrequency and microwave tumor ablation in patients with implanted cardiac devices: is it safe? Eur J Radiol 2011;79:343–6.
- vanSonnenberg E, Shankar S, Morrison PR, Nair RT, Silverman SG, Jaklitsch MT, et al. Radiofrequency ablation of thoracic lesions: part 2, initial clinical experience – technical and multidisciplinary considerations in 30 patients. Am J Roentgenol 2005;4:381–90.
- Solomon SB, Thornton RH, Dupuy DE, Downey RJ. Protection of the mediastinum and chest wall with an artificial pneumothorax during lung ablations. J Vasc Interv Radiol 2008;19:610–15.
- Yamamoto A, Nakamura K, Matsuoka T, Toyoshima M, Okuma T, Oyama Y, et al. Radiofrequency ablation in a porcine lung model: correlation between CT and histopathologic findings. Am J Roentgenol 2005;185:1299–306.
- Baeère TD, Palussière J, Aupérin A, Hakime A, Abdel-Rehim M, Kind M, et al. Midterm local efficacy and survival after radiofrequency ablation of lung tumors with minimum follow-up of 1 year: prospective evaluation 1. Radiology 2006;240:587–96.
- Hinshaw JL, Lubner MG, Ziemlewicz TJ, Lee Jr FT, Brace CL. Percutaneous tumor ablation tools: microwave, radiofrequency, or cryoablation – what should you use and why? Radiographics 2014;34:1344–62.
- Beland MD, Wasser EJ, Mayo-Smith WW, Dupuy DE. Primary non-small cell lung cancer: review of frequency, location, and time of recurrence after radiofrequency ablation. Radiology 2010;254:301–7.
- Suh RD, Wallace AB, Sheehan RE, Heinze SB, Goldin JG. Unresectable pulmonary malignancies: CT-guided percutaneous radiofrequency ablation – preliminary results. Radiology 2003;229:821–9.
- Wolf FJ, Grand DJ, Machan JT, Dipetrillo TA, Mayo-Smith WW, Dupuy DE. Microwave ablation of lung malignancies: Effectiveness, CT findings, and safety in 50 patients. Radiology 2008;247:871–9.
- Abtin FG, Eradat J, Gutierrez AJ, Lee C, Fishbein MC, Suh RD. Radiofrequency ablation of lung tumors: Imaging features of the postablation zone. Radiographics 2012;32:947–69.
- Chheang S, Abtin F, Guteirrez A, Genshaft S, Suh R. Imaging features following thermal ablation of lung malignancies. Semin Intervent Radiol 2013;30:157–68.
- Okuma T, Okamura T, Matsuoka T, Yamamoto A, Oyama Y, Toyoshima M, et al. Fluorine-18-fluorodeoxyglucose positron emission tomography for assessment of patients with unresectable recurrent or metastatic lung cancers after CT-guided radiofrequency ablation: preliminary results. Ann Nucl Med 2006;20:115–21.
- Akeboshi M, Yamakado K, Nakatsuka A, Hataji O, Taguchi O, Takao M, Takeda K. Percutaneous radiofrequency ablation of lung neoplasms: initial therapeutic response. J Vasc Interv Radiol 2004;15:463–70.
- Zemlyak A, Moore WH, Bilfinger TV. Comparison of survival after sublobar resections and ablative therapies for stage I non-small cell lung cancer. J Am Coll Surg 2010;211:68–72.
- de Baere T, Aupérin A, Deschamps F, Chevallier P, Gaubert Y, Boige V, et al. Radiofrequency ablation is a valid treatment option for lung metastases: experience in 566 patients with 1037 metastases. Ann Oncol 2015;26:987–91.
- Dupuy DE, Fernando HC, Hillman S, Ng T, Tan AD, Sharma A, et al. Radiofrequency ablation of stage IA non-small cell lung cancer in medically inoperable patients: Results from the American College of Surgeons Oncology Group Z4033 (Alliance) trial. Cancer 2015;121:3491–8.
- Howington JA, Blum MG, Chang AC, Balekian AA, Murthy SC. Treatment of stage I and II non-small cell lung cancer: diagnosis and management of lung cancer: American College of Chest Physicians evidence-based clinical practice guidelines. CHEST J 2013;143:eS278–313.
- Nahum Goldberg S, Dupuy DE. Image-guided radiofrequency tumor ablation: challenges and opportunities – part I. J Vasc Interv Radiol 2001;12:1021–32.
- Nemcek AA. Complications of radiofrequency ablation of neoplasms. Semin Interv Radiol 2006;23:177–87.
- Brace CL, Hinshaw JL, Laeseke PF, Sampson LA, Lee FT Jr. Pulmonary thermal ablation: Comparison of radiofrequency and microwave devices by using gross pathologic and CT findings in a swine model. Radiology 2009;251:705–11.
- Goldberg SN, Gazelle GS, Solbiati L, Rittman WJ, Mueller PR. Radiofrequency tissue ablation: Increased lesion diameter with a perfusion electrode. Acad Radiol 1996;3:636–44.
- Dupuy DE. Microwave ablation compared with radiofrequency ablation in lung tissue – is microwave not just for popcorn anymore? Radiology 2009;251:617–18.
- Simon CJ, Dupuy DE, Mayo-Smith WW. Microwave ablation: principles and applications 1. Radiographics 2005;25:S69–S83.
- Belfiore G, Ronza F, Belfiore MP, Serao N, di Ronza G, Grassi R, Rotondo A. Patients’ survival in lung malignancies treated by microwave ablation: Our experience on 56 patients. Eur J Radiol 2013;82:177–81.
- Palussiere J, Lagarde P, Aupérin A, Deschamps F, Chomy F, de Baere T. Percutaneous lung thermal ablation of non-surgical clinical N0 non-small cell lung cancer: Results of eight years’ experience in 87 patients from two centers. Cardiovasc Intervent Radiol 2015;38:160–6.
- Vogl TJ, Naguib NN, Gruber-Rouh T, Koitka K, Lehnert T, Nour-Eldin NE. Microwave ablation therapy: Clinical utility in treatment of pulmonary metastases. Radiology 2011;261:643–51.
- Wang H, Littrup PJ, Duan Y, Zhang Y, Feng H, Nie Z. Thoracic masses treated with percutaneous cryotherapy: Initial experience with more than 200 procedures. Radiology 2005;235:289–98.
- Maiwand MO. The role of cryosurgery in palliation of tracheo-bronchial carcinoma. Eur J Cardiothorac Surg 1999;15:764–8.
- Dupuy DE, Shulman M. Current status of thermal ablation treatments for lung malignancies. Semin Intervent Radiol. 2010;27:268–75.
- Yashiro H, Nakatsuka S, Inoue M, Kawamura M, Tsukada N, Asakura K, et al. Factors affecting local progression after percutaneous cryoablation of lung tumors. J Vasc Intervent Radiol 2013;24:813–21.
- Moore W, Talati R, Bhattacharji P, Bilfinger T. Five-year survival after cryoablation of stage I non-small cell lung cancer in medically inoperable patients. J Vasc Interv Radiol 2015;26:312–19.
- De Baere T, Tselikas L, Woodrum D, Abtin F, Littrup P, Deschamps F, et al. Evaluating cryoablation of metastatic lung tumors in patients – safety and efficacy the ECLIPSE trial – interim analysis at 1 year. J Thorac Oncol 2015;10:1468–74.
- Timmerman R, Paulus R, Galvin J, Michalski J, Straube W, Bradley J, et al. Stereotactic body radiation therapy for inoperable early stage lung cancer. JAMA 2010;303:1070–6.
- Kwan SW, Mortell KE, Talenfeld AD, Brunner MC. Thermal ablation matches sublobar resection outcomes in older patients with early-stage non-small cell lung cancer. J Vasc Intervent Radiol 2014;25:1–9.
- Kwan SW, Mortell KE, Hippe DS, Brunner MC. An economic analysis of sublobar resection versus thermal ablation for early-stage non–small-cell lung cancer. J Vasc Intervent Radiol 2014;25:1558–64.
- Lencioni R, Crocetti L, Cioni R, Suh R, Glenn D, Regge D, et al. Response to radiofrequency ablation of pulmonary tumours: A prospective, intention-to-treat, multicentre clinical trial (the RAPTURE study). Lancet Oncol 2008;9:621–8.
- Lanuti M, Sharma A, Digumarthy SR, Wright CD, Donahue DM, Wain JC, et al. Radiofrequency ablation for treatment of medically inoperable stage I non-small cell lung cancer. J Thorac Cardiovasc Surg 2009;137:160–6.
- Hiraki T, Gobara H, Mimura H, Matsui Y, Toyooka S, Kanazawa S. Percutaneous radiofrequency ablation of clinical stage I non-small cell lung cancer. J Thorac Cardiovasc Surg 2011;142:24–30.
- Horkan C, Dalal K, Coderre JA, Kiger JL, Dupuy DE, Signoretti S, et al. Reduced tumor growth with combined radiofrequency ablation and radiation therapy in a rat breast tumor model. Radiology 2005;235:81–8.
- Chan MD, Dupuy DE, Mayo-Smith WW, Ng T, DiPetrillo TA. Combined radiofrequency ablation and high-dose rate brachytherapy for early-stage non-small cell lung cancer. Brachytherapy 2011;10:253–9.