Abstract
Purpose: Hyperthermia (40–44 °C) effectively sensitises tumours to radiotherapy by locally altering tumour biology. One of the effects of heat at the cellular level is inhibition of DNA repair by homologous recombination via degradation of the BRCA2-protein. This suggests that hyperthermia can expand the group of patients that benefit from PARP-inhibitors, a drug exploiting homologous recombination deficiency. Here, we explore whether the molecular mechanisms that cause heat-mediated degradation of BRCA2 are conserved in cell lines from various origins and, most importantly, whether, BRCA2 protein levels can be attenuated by heat in freshly biopted human tumours.
Experimental design: Cells from four established cell lines and from freshly biopsied material of cervical (15), head- and neck (9) or bladder tumours (27) were heated to 42 °C for 60 min ex vivo. In vivo hyperthermia was studied by taking two biopsies of the same breast or cervical tumour: one before and one after treatment. BRCA2 protein levels were measured by immunoblotting.
Results: We found decreased BRCA2-levels after hyperthermia in all established cell lines and in 91% of all tumours treated ex vivo. For tumours treated with hyperthermia in vivo, technical issues and intra-tumour heterogeneity prevented obtaining interpretable results.
Conclusions: This study demonstrates that heat-mediated degradation of BRCA2 occurs in tumour material directly derived from patients. Although BRCA2-degradation may not be a practical biomarker for heat deposition in situ, it does suggest that application of hyperthermia could be an effective method to expand the patient group that could benefit from PARP-inhibitors.
Introduction
Hyperthermia is an anti-cancer therapy that increases efficacy of radiation therapy and of several chemotherapeutic agents [Citation1,Citation2]. During hyperthermia treatment, external applicators are positioned in such a way that the temperature of the tumour is elevated to 40–44 °C. Hyperthermia application devices have been designed to heat various tumour types in a localised fashion. A broad range of tumours can be treated with hyperthermia including, but not limited to, those occurring in the breast [Citation3,Citation4], cervix [Citation5,Citation6], head and neck [Citation7,Citation8], and bladder [Citation9–11], resulting in growing interest for using hyperthermia as a means to increase the effectiveness and specificity of cancer treatment while minimising side-effects [Citation12].
Hyperthermia sensitises tumour cells to radiation and chemotherapy by altering tumour biology, both at the physiological and molecular level [Citation13,Citation14]. The most well-investigated and well-known physiological effects of hyperthermia are the increase in local blood flow and the alterations in vascular permeability, and the change in tumour microenvironment [Citation14,Citation15]. Another physiological effect of hyperthermia that has recently gained more attention is the activation of the immune response by heat [Citation16,Citation17]. On the molecular level, hyperthermia induces membrane stress and it causes protein structures to unfold. All effects of hyperthermia are very dependent on the temperature reached in the tumour and the duration of the treatment. For example, most proteins remain relatively stable with temperatures in a near-physiological range (<42 °C) [Citation18]. However, one known exception is the protein BRCA2, which is degraded at temperatures as low as 41 °C [Citation19].
BRCA2 is an essential player in the DNA repair pathway homologous recombination (HR). This DNA repair pathway is employed by cells to aid in the repair of numerous aberrant DNA structures or DNA lesions [Citation20]. A prime example of a complicated lesion that can be repaired through HR is a DNA double strand break. Once a DNA double strand break has occurred in one chromatid, HR taps into the information on the undamaged sister chromatid to repair the break, thereby ensuring faithful restoration. The necessity for the presence of a sister chromatid, limits HR to the S- and G2-phases of the cell cycle [Citation20]. The main driver proteins of HR are RAD51 and its mediator BRCA2 [Citation21]. Absence of the BRCA2-protein abrogates HR by causing defects in RAD51 loading onto the processed end(s) of the DNA double strand break [Citation22,Citation23].
By degrading BRCA2 in tumour cells, hyperthermia attenuates HR and creates a temporary window for DNA damage to persist, which is one of the explanations for how it sensitises to DNA-damaging anti-cancer treatments, such as radiation therapy and chemotherapy [Citation19]. Likewise, tumours that harbour a heritable BRCA2-mutation resulting in a dysfunctional BRCA2-protein also lack functional HR, causing the tumours to be very sensitive to a specific type of drugs: PARP-inhibitors [Citation24,Citation25]. PARP-inhibitors target the PARP-1 (Poly [ADP-ribose] Polymerase 1) protein, which is involved in repair of single strand DNA breaks. When PARP-1 is not available to repair these DNA breaks, the single strand lesions are converted to double strand breaks during DNA replication. These replication-induced DNA double strand breaks are extremely toxic to cells, and have to be repaired via the HR-pathway in order for cells to survive [Citation26]. This explains why HR-defective tumours are extremely sensitive to PARP-inhibitors, while they are much less toxic to HR-proficient cells [Citation24,Citation25]. This results in PARP-inhibitors being excellent precision medicine to treat patients with HR-deficient cancers, because the patients themselves will experience minimal side-effects, while the tumour is very sensitive to the drug. Recent approvals of two variants of PARP-inhibitors (Olaparib/Lynparza, AstraZeneca and Rucaparib, Clovis) for treatment of BRCA-mutated ovarian tumours by the FDA demonstrate the popularity and effectivity of this type of anti-cancer approach [Citation27].
Although this precision treatment method is very elegant, one inherent drawback is the small group of patients that are eligible for these drugs, because a BRCA-mutation is rare [Citation28]. By inducing temporary HR-deficiency in tumours in a local fashion, hyperthermia could have the potential to enlarge the patient group that might benefit from PARP-inhibitors, irrespective of their BRCA gene status. However, it is unknown whether the molecular mechanisms present in established tumour cell lines, and necessary for hyperthermia-mediated degradation of BRCA2, are also functioning in primary tumour tissue. Therefore, we explore hyperthermia’s ability to degrade BRCA2 in primary tumour material of tumours that can clinically be heated, as a proof-of-concept study and as a first step towards application of PARP-inhibitors and hyperthermia in a clinical setting. Moreover, because the currently available heating techniques in vivo harbour large variability in heating patterns and their quality assurance is challenging, we also explore the possibility to use BRCA2 degradation in tumours as a biomarker for the effectivity of hyperthermia.
Materials and methods
Cell culture
HeLa, FaDu, T24 and MDA-MB-231 were cultured as previously described [Citation19].
Tumour collection (ex vivo study)
The effects of hyperthermia on BRCA2 protein levels were studied in tumour material by subjecting collected biopsies to heat ex vivo. All described specimens were obtained between August 2012 and December 2016 within the Erasmus MC Cancer Institute, Rotterdam (MEC 2017.234). All tumours included in this study were leftover tissues after pathological reviews, and could therefore be used for research according to the “Code Proper Secondary Use of Human Tissue”, founded by the Dutch Federation of Medical Societies (www.fmwv.nl). All patients were informed by letter that they might refuse the use of leftover tissue for research purposes, in an opt-out manner. Tumour tissue specimens were anonymised by coding, so that they could not be traced back to individual patients.
Fresh cervical tumour tissue was taken with a biopsy forceps from patients with locally advanced cervical carcinoma (FIGO stage IIB-IVA) or recurrent disease, undergoing pelvic examination under anaesthesia. All head and neck specimens were primary tumours originating from the tongue and obtained during COMMANDO-surgery. Bladder specimens of both primary and recurrent tumours were collected during transurethral resection procedures.
Tumour collection and hyperthermia treatment (in vivo studies)
The study was continued by investigating BRCA2 protein levels in biopsies of breast and cervical tumours taken before and after clinical application of hyperthermia (in vivo). In the first study (MEC 2012.559) we aimed to include 12 patients who had local recurrent breast cancer without distant metastases for whom treatment with superficial hyperthermia was feasible. Patients with a BRCA1 or BRCA2 germline mutation were excluded from this study. The biopsies were taken with a 4-mm cutter from a previously irradiated area, right before and directly after an extra hyperthermia session before the start of the combined treatment. The study-related hyperthermia treatment was limited to one 433 MHz Lucite cone applicator [Citation29] for superficial hyperthermia and given for 30 min. Due to technical problems, the study was closed after inclusion of five biopsy sets originating from four patients.
For the second study (MEC 2014.469) we aimed to include 16 patients with locally advanced cervical carcinoma that underwent hyperthermia as part of their primary treatment. The included patients were treated with hyperthermia according to the standard treatment protocol with the BSD-2000 3D system (Pyrexar Medical Systems, Salt Lake City, UT). If possible, the first biopsy was taken during the examination under anaesthesia, approximately four weeks before the start of the treatment, to reduce patient burden. To prevent radiotherapy-induced alterations of tumours included in this study, the sequence of the first radiotherapy and hyperthermia combination was altered: before the patient received the first radiotherapy fraction, the second biopsy was taken after one hour hyperthermia treatment (excluding pre-heating time). The remaining four hyperthermia treatments of the treatment cycle were delivered directly after radiotherapy. The patients in these two studies were recruited between July 2013 and May 2016, and a written informed consent was obtained from all patients.
Tumour dissociation and ex vivo hyperthermia treatment
Tumour biopsies were first transferred to the Department of Pathology, for inspection by a pathologist. After analysis, remaining material was transported to the laboratory in RPMI medium (Sigma-Aldrich, St. Louis, MO), supplemented with penicillin/streptomycin. The material was processed immediately upon arrival, and macroscopically visible blood vessels and fat were removed. The tumour was manually cut into small pieces (1 mm3). The samples were then homogenised using a human Tumour Dissociation kit and corresponding protocol (Miltenyi Biotec, Bergisch Gladbag, Germany). Cells in the homogenised mixture from tumours collected within the context of the in vivo study were lysed immediately, while cells from tumours in the ex vivo study were treated as described in .
Cell lysis
Cells were lysed as previously described [Citation19]. Prior to lysis, the cell suspension from tumour material was harvested by centrifuging (5 min, 300 rcf, 4 °C). The pellet then washed twice by resuspension in cold PBS supplemented with 5 mM EDTA and subsequent centrifugation. If necessary, blood was removed from the pellet by resuspension and subsequent incubation for 2 min at room temperature in ACK buffer (150 mM NH4Cl, 10 mM KHCO3, 0.1 mM Na2EDTA, pH 7.3), after which the sample was centrifuged and washed once more with cold PBS. Following the last centrifugation step, the pellet was resuspended in an appropriate amount of PBS, supplemented with 5 mM EDTA, 2.5x cOmplete™ Protease Inhibitor cocktail (Roche, Basel, Switzerland) and 1 mg/ml Pefabloc® SC (Sigma-Aldrich, St Louis, MO).
Immunoblotting procedure
After determining protein concentration in the samples using a Lowry assay [Citation30], 50–75 μg of the tumour lysate was loaded and run on a 3–8% gradient Tris-Act gel (Novex, Thermo Fisher Scientific, Waltham, MA). The separated proteins were blotted on a PVDF membrane in a tank containing transfer buffer (0.4 M Glycine, 5 mM Tris, 20% methanol) at a constant rate of 300 mA for two hours at 4 °C. The membrane was horizontally cut into three separate pieces, which were blocked for at least one hour in 3% milk in PBS-T (0.05% Tween). The primary antibodies, BRCA2 (1:1000, OP95, Calbiochem, San Diego, CA), PARP-1 (1:5000, Alexis/Enzo, Farmingdale, NY) and Origin replication complex 2 (ORC2) (1:1000, BD Pharmingen, San Diego, CA), were diluted in PBS-T with 3% milk and incubated overnight at 4 °C. Blots were washed in PBS-T and incubated with a secondary antibody (Sheep-anti-mouse HRP conjugated 1:2000; Jackson Immunoresearch, Suffolk, UK) for two hours at room temperature. The signal was detected in an Alliance (Uvitec Cambridge, Cleaver Scientific, Warwickshire, UK) at several exposure times. The signal was detected by enhancing the signal with ECL (Amersham; GE Life Sciences, Chicago, IL), but if the BRCA2 signal proved too weak to detect, an enhanced version of ECL (Supernova, Cyanagen, Bologna, Italy or Pierce, Thermo Fisher Scientific, Waltham, MA) was used. In the presentation of the blots, an asterisk indicates that the detected signals were enhanced by changing the shadows-value from 0 to 175 in the “levels adjustment” tool in Photoshop (CS6, Adobe). This adjustment was necessary for a clear impression of the relative signal intensities.
Blot quantification
Antibody signals on the immunoblot were quantified using the FIJI [Citation31] “analyze gels” tool. To minimalize saturation of the quantified signals, different exposure times were analysed. The BRCA2 signals were normalised to ORC2 to correct for unequal loading, with notable exception of one bladder tumour where we did not find ORC2 expression and used PARP-1 instead. In the figures, the 42 °C signal is always expressed as a percentage of the matched 37 °C control.
Results
Mild hyperthermia induces BRCA2 degradation in various established cell lines
We started our proof-of-concept study by testing whether hyperthermia induces BRCA2 degradation in established cell lines of various origins. The selected cell lines, HeLa (cervix), FaDu (head and neck), T24 (bladder), and MDA-MB-231 (breast), correspond to tumour types that are routinely treated with hyperthermia in the Erasmus MC Cancer Institute. After 60 min of effective hyperthermia, the cells were lysed, immunoblotted and the intensity of the BRCA2-signal was analysed. We observed that in all cell lines employed the BRCA2-signal was markedly decreased by treatment with 42 °C (), demonstrating that hyperthermia is able to degrade BRCA2 in multiple tumour cell lines.
Figure 1. BRCA2 degradation in established cell lines of various tumour origins. Immunoblots of cells without treatment (37 °C) or treated with hyperthermia (42 °C) for 60 min effectively, excluding 15 min of pre-heating time. Upper row of the panels show the BRCA2-signal, the lower panel shows the PARP-1-signal. Each panel represents a set samples from a different cell line, from left to right: HeLa (cervical), FaDu (head- and neck), T24 (bladder) and MDA-MB-231 (breast). Percentages at the bottom of each panel indicate the relative intensity of the BRCA2 signal (corrected to PARP-1) in the heat-treated sample compared to the non-treated sample.
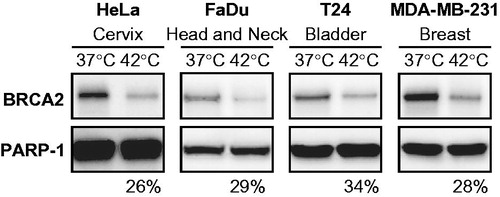
Hyperthermia-induced BRCA2 degradation in primary material
We extended our study by analysing BRCA2 degradation upon hyperthermia in fresh tumour material. We collected tumours from different sites that are treated with mild hyperthermia: cervix (15), head and neck (9) and bladder (27). The tumours were directly transported to the laboratory, dissociated upon arrival and the resulting suspension was divided in two aliquots: one aliquot was incubated ex vivo at 37 °C and the other at 42 °C for 75 min total. We then lysed the cells, analysed the resulting samples by immunoblot and subsequently quantified the BRCA2-signals, when possible (). Because we were not always able to prevent loading differences between the two samples of the same tumour, we normalised the BRCA2-signals to the ORC2-signal in the same sample. We then expressed the normalised BRCA2-signal in the hyperthermia-treated sample as a percentage of that in the untreated sample.
Figure 2. Schematic representation of tumour collection. Fresh biopsies obtained are dissociated enzymatically and mechanically. Dissociated suspensions of tumours collected were prepared for treatment by centrifugation (5 min, 300 rcf) and subsequent resuspension in RMPI supplemented with 10% FCS. The resulting cell suspension was divided over two separate culture dishes: one was incubated at 37 °C for 75 min in a controlled environment (37 °C, 5% CO2, 20% O2), while the other was incubated at 42 °C in a similarly controlled environment for the same amount of time, allowing 15 min to pre-heat the medium and thus resulting in 60 min of effective heating. After the treatment, cells in both samples are lysed and the proteins are analysed on an immunoblot.
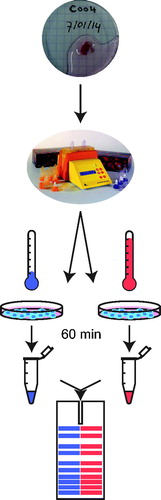
The loading difference was mainly caused by the relatively mild nature of the employed dissociation method and the differences in tumour structure, sometimes resulting in incomplete homogenisation of the tumour samples. Even though we used the Lowry protein assay to determine the total protein content in the lysates and corrected for the amount measured, loading differences within the same tumour set could not always be prevented. When necessary, we re-ran samples, by adjusting the amount loaded based on the previous obtained result. However, this was not always possible, because relative to the size of the samples obtained, a significant amount of material is needed to detect BRCA2 on a Western blot. To solve this, we normalised the signal to ORC2, its signal intensity being comparable to that of BRCA2. For one bladder tumour we used the PARP-1 signal to normalise, because no ORC2-signal could be detected (marked with ** in ).
Figure 3. BRCA2 degradation in tumours heated ex vivo. Immunoblots of (A) 11 cervical tumours, (B) five head and neck tumours, and (C) 16 bladder tumours. Each pair of samples represents the non-hyperthermia-treated and treated samples of a tumour sample. ORC2 is shown as a loading control. Percentages at the bottom of each panel indicate the relative intensity of the BRCA2 signal (corrected to ORC2) in the heat-treated sample compared to the non-treated sample. *For clarity of presentation, the shadow values were reset from 0 to 175 in Adobe Photoshop. **PARP-1 is shown as a loading control and as an alternative to ORC2, because the latter could not be detected in this sample.
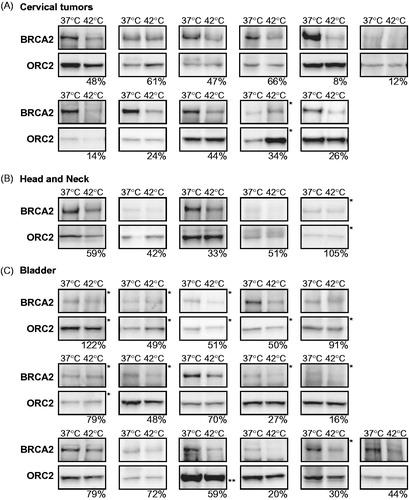
Due to technical issues during dissociation or immunoblotting, not all tumours included could be analysed, but we were able to obtain results of 11 out of 15 cervical tumours, five out of nine head- and neck tumours and 16 out of 27 collected bladder tumours. In the cervical tumours we detected degradation of BRCA2 in all ex vivo treated samples, the residual BRCA2-levels varying between 8% and 66% (). In the head and neck tumours, we found BRCA2 degradation in four samples (33–59% remaining levels after 42 °C), but hyperthermia did not result in degradation of the BRCA2 protein in the fifth (). In material obtained from bladder tumours, hyperthermia degraded BRCA2 in 14 samples (20–79% residual protein levels), but in two samples we found no clear alteration of BRCA2-protein levels upon heat treatment ().
BRCA2 degradation as a biomarker for heat deposition
Next, we checked if BRCA2 degradation in biopsy material can be used as a biomarker for effectivity of hyperthermia [Citation4,Citation32,Citation33]. To this end, two pilot studies were set up in which we investigated BRCA2 protein levels in biopsies of recurrent breast and primary cervical tumours that were taken before and directly after clinical treatment with mild hyperthermia.
In the first pilot study, we included a total of four patients with local recurrent breast cancer without distant metastases, of which one patient was included twice. The biopsies we tested were taken from previously irradiated areas of the tumour, as only patients with these lesions have a standard indication for treatment with hyperthermia. Moreover, superficial hyperthermia elicits higher temperatures, has a more homogeneous heating pattern and is less demanding for patients, compared to deep hyperthermia [Citation3,Citation6]. Unfortunately, we found that the dissociation of the biopsies obtained from these areas was insufficient, in contrast to our previous experiences with primary biopsies. These difficulties might be caused by macroscopic changes in the extracellular matrix and tumour structure in the re-irradiated areas. Owing to the insufficient dissociation, we were only able to detect a BRCA2-signal on the immunoblot in the biopsy material from two patients, and never in both biopsies from the same patient (). Because the technical problems in this patient group prevented us from investigating the effect of clinically applied hyperthermia on the BRCA2-protein levels in the tumours, we decided to terminate this study.
Figure 4. BRCA2 protein levels in biopsies taken before and after hyperthermia treatment in vivo. Immunoblots of biopsies taken before (pre) and after (post) hyperthermia in (A) breast tumours and in (B) cervical tumours. Samples marked with “42 °C” received ex vivo hyperthermia as an internal control. Shown are BRCA2 and ORC2. The percentages at the bottom of each panel indicate the relative intensity of the BRCA2 signal (corrected to ORC2) in the heat-treated sample compared to the non-treated sample. *For clarity of presentation the shadow values were reset from 0 to 175 in Adobe Photoshop.
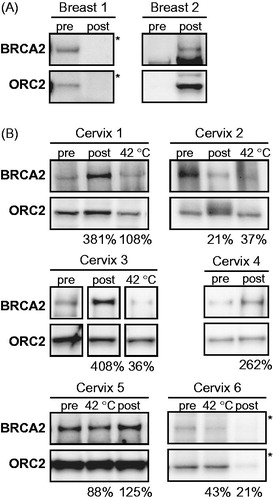
In the second pilot study, we included six patients with locally advanced cervical tumours, who are treated with a combination treatment of hyperthermia and radiotherapy as a standard of care [Citation6]. In all biopsies tested, we were able to detect BRCA2 (). We compared the BRCA2 signal of the two biopsies taken before and directly after the end of the hyperthermia to examine the effects of in vivo hyperthermia and found: (1) lower BRCA2-levels post-hyperthermia than pre-hyperthermia in two cases (cervix 2 and 6, ); (2) similar levels of BRCA2 in both biopsies in one case (cervix 5, ); and (3) considerably more (>2 fold) BRCA2 in the post-hyperthermia biopsy in three cases (cervix 1, 3 and 4, ).
In five of these tumours, the amount of material obtained was sufficient to include an ex vivo hyperthermia treatment on the same biopsy material taken before the clinical hyperthermia treatment, as a control for the effectivity of hyperthermia in vivo (, indicated with 42 °C). In contrast to the cervix tumour samples presented in , where ex vivo hyperthermia triggered BRCA2 degradation in all cases, the same treatment did not induce degradation of BRCA2 in two tumours within this set (cervix 1 and 5, ). However, importantly, we did observe that the increase in BRCA2 found in the post-hyperthermia biopsies of cervix 1 and 3 did not occur in the controlled ex vivo hyperthermia, and were therefore most probably not inherent to molecular mechanism in the tumour itself.
The variability in relative BRCA2 levels in the tumour biopsy taken after hyperthermia treatment in vivo might be explained by tumour heterogeneity, which would cause inherent differences between the two biopsied parts of the tumour. To test this possibility, we took biopsies (designated biopsy A and B) from two macroscopically similar parts of the same cervical tumour during an examination under anaesthesia, and ex vivo incubated half of each biopsy at 37 °C and the other half at 42 °C. In both sample-sets, we observed BRCA2 degradation to an equal extent upon heat. However, the initial BRCA2-levels at 37 °C were very different (). Theoretically, if biopsy B would have been the biopsy taken before hyperthermia in vivo, and if biopsy A was taken after hyperthermia, we would have found no differences in BRCA2-levels, while BRCA2 was in fact degraded by the heat treatment ().
Figure 5. Tumour heterogeneity contributes to pre-hyperthermia protein levels of BRCA2 in cervical tumours. Immunoblots of two biopsies (A and B) taken from the same cervical tumour and treated ex vivo in the same hyperthermia-session at 42 °C. Upper panel shows the BRCA2-signal, the lower ORC2. The percentages at the bottom of the panel indicate the relative intensity of the BRCA2 signal (corrected to ORC2) in the heat-treated sample compared to the non-treated sample for each biopsy.
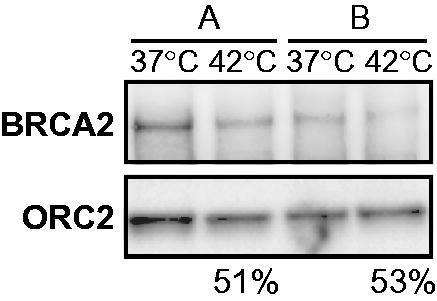
Discussion
Treatment with hyperthermia (41–42 °C, 60 min) induces degradation of the BRCA2 protein in established cell lines, resulting in a temporary inhibition of the DNA repair pathway homologous recombination (HR) [Citation19]. This provides a possible explanation for hyperthermia’s sensitising effects towards irradiation and chemotherapeutic compounds that induce double strand breaks, such as cisplatin [Citation34,Citation35] and Trabectedin [Citation36]. Moreover, attenuation of HR by hyperthermia sensitises cultured cells and mouse tumours to PARP-inhibitors [Citation19,Citation37–39], a class of therapeutics that specifically target cells deficient in HR. PARP-inhibitors are currently available as personalised treatment to patients with ovarian cancer that harbour a BRCA-mutation and have relapsed after initial platinum-containing treatment [Citation27]. Via inhibition of HR, mild hyperthermia has the potential to enlarge the group of patients eligible for this treatment, especially because it can be applied in a loco-regional fashion and is available to treat a broad range of tumours [Citation40].
In this study, we aimed to demonstrate that hyperthermia induces degradation of BRCA2 not only in established cell lines, but also in fresh, human tumours. We focussed on tumour types that can be treated with hyperthermia in daily practice: cervix, head and neck, bladder and breast. We first show that BRCA2 is degraded upon heat (60 min at 42 °C) in established cell lines of these tumour types. We then collected cervix, head and neck and bladder tumours, and tested BRCA2 protein degradation by shifting the temperature ex vivo from 37 °C to 42 °C for 60 min. The BRCA2 protein levels after hyperthermia were lower than without treatment in 11/11 cervical tumours in 4/5 of the head and neck tumours and in 14/16 of the bladder tumours origin (summarized in Figure S1), demonstrating that the molecular pathways that mediate BRCA2-degradation upon heat are functional in fresh tumour material, independent of its.
Our final aim was to determine whether BRCA2-degradation could be used as a biomarker for efficiency of clinical hyperthermia treatment. We investigated this by obtaining two biopsies of the same breast tumour or cervical tumour: one taken before and one taken after in vivo hyperthermia treatment. Due to unforeseen technical problems with the preparation of the material obtained in the breast cancer study, we were unable to obtain interpretable results. In the six cervical cancer patients included, we found unexpected variation in the BRCA2-levels in the biopsy taken after clinically applied hyperthermia relative to the levels in the biopsies taken before hyperthermia.
The possibility that thermal doses reached in the clinical setting are not sufficient to induce BRCA2 degradation does not explain the observed increase in BRCA2 protein levels after hyperthermia. However, there are several other factors that could contribute to the variability in relative BRCA2-levels in tumour biopsies taken after hyperthermia treatment in vivo. The first explanation is the inherent variability of BRCA2-protein levels in each biopsy, caused by intra-tumour heterogeneity (), a feature which commonly occurs in cervical tumours [Citation41–43]. Differences in the cell cycle distribution within a tumour part would influence the BRCA2 protein levels, because its expression depends heavily on the cycling state of the cells [Citation44]. These inherent tumour differences might be amplified by the fact that, in four of the six cases, we opted to obtain the pre-hyperthermia biopsy up to a month before the start of the treatment, in order to reduce the burden on the patient (, cervix 1, 2, 5 and 6). Other biological events that may have contributed to the increase in BRCA2 protein levels after hyperthermia are the physiological effects of hyperthermia in vivo: the increased blood flow and the immunological response upon heat could introduce new cells to the tumour, in which the BRCA2 proteins have not had a chance to be affected by heat [Citation45]. Since the logistics in this study limited us to taking the second biopsy approximately 45 min after the end of hyperthermia, non-heated, non-tumour cells potentially could have infiltrated the tumour after the end of treatment, clouding the effects of hyperthermia on BRCA2.
The results obtained in the studies that examine the effects of clinically applied hyperthermia on BRCA2, demonstrate that no valid conclusions can be drawn when comparing immunoblot signals resulting from two separate biopsies. In a clinical setting, the BRCA2-signal in a first biopsy can therefore not be used as a control for the BRCA2-signal in the biopsy taken after hyperthermia. However, the results do imply that an experimental approach where a tumour biopsy is taken before hyperthermia and another one after could be a valuable method to identify differences in the cell populations in the biopsies upon hyperthermia, elucidating physiological or immunological mechanisms of hyperthermia.
In this study we aimed to explore hyperthermia’s ability to degrade BRCA2 in freshly biopsied tumour material. We have indeed demonstrated that heat-induced BRCA2 degradation is not limited to established cell lines, but is also applicable to tumours heated ex vivo. We also explored whether BRCA2-degradation could be a feasible biomarker for determining effectivity of heat deposition by clinically applied hyperthermia, but found that this is not the case: due to technical difficulties in dissociation of some tumours and intra-tumour heterogeneity, it was not possible to accurately determine BRCA2-levels after hyperthermia in vivo. However, the results of this study indicate that hyperthermia is an effective method to create a local environment of HR-deficiency in tumours, and could indeed provide a rational basis to increase the group of patients that could potentially benefit from PARP-inhibitors.
Acknowledgements
The authors would like to thank Titia Meijer, Nicole Verkaik and Cecile Beerens for their help with collecting breast and head and neck tumours, Coby van der Zee for her help with the first breast patient, Claire Wyman for proofreading the manuscript and the members of the Clinical Chemistry laboratory of the Erasmus MC Cancer Institute for their hospitality.
Disclosure statement
No potential conflict of interest was reported by the authors.
Additional information
Funding
References
- Horsman MR, Overgaard J. (2007). Hyperthermia: a potent enhancer of radiotherapy. Clin Oncol (R Coll Radiol) 19:418–26.
- Issels RD. (2008). Hyperthermia adds to chemotherapy. Eur J Cancer 44:2546–54.
- Van Der Zee J, De Bruijne M, Mens JWM, et al. (2010). Reirradiation combined with hyperthermia in breast cancer recurrences: overview of experience in Erasmus MC. Int J Hyperth 26:638–48.
- Linthorst M, Baaijens M, Wiggenraad R, et al. (2015). Local control rate after the combination of re-irradiation and hyperthermia for irresectable recurrent breast cancer: results in 248 patients. Radiother Oncol 117:217–22.
- van der Zee J, van Rhoon GC. (2006). Cervical cancer: radiotherapy and hyperthermia. Int J Hyperthermia 22:229–34.
- Franckena M. (2012). Review of radiotherapy and hyperthermia in primary cervical cancer. Int J Hyperthermia 28:543–8.
- Paulides MM, Verduijn GM, Van Holthe N. (2016). Status quo and directions in deep head and neck hyperthermia. Radiat Oncol 11:21.
- Datta NR, Rogers S, Ordonez SG, et al. (2016). Hyperthermia and radiotherapy in the management of head and neck cancers: a systematic review and meta-analysis. Int J Hyperthermia 32:31–40.
- Rampersaud EN, Vujaskovic Z, Inman BA. (2010). Hyperthermia as a treatment for bladder cancer. Oncology (Williston Park, N.Y.) 24:1149–55.
- Longo TA, Gopalakrishna A, Tsivian M, et al. (2016). A systematic review of regional hyperthermia therapy in bladder cancer. Int J Hyperthermia 32:381–9.
- Crezee H, Inman BA. (2016). The use of hyperthermia in the treatment of bladder cancer. Int J Hyperthermia 32:349–50.
- Wust P, Hildebrandt B, Sreenivasa G, et al. (2002). Hyperthermia in combined treatment of cancer. Lancet Oncol 3:487–97.
- Dewhirst MW, Lee C-T, Ashcraft KA. (2016). The future of biology in driving the field of hyperthermia. Int J Hyperthermia 32:4–13.
- van den Tempel N, Horsman MR, Kanaar R. (2016). Improving efficacy of hyperthermia in oncology by exploiting biological mechanisms. Int J Hyperthermia 32:446–54.
- Vujaskovic Z, Song CW. (2004). Physiological mechanisms underlying heat-induced radiosensitization. Int J Hyperthermia 20:163–74.
- Evans SS, Repasky EA, Fisher DT. (2015). Fever and the thermal regulation of immunity: the immune system feels the heat. Nat Rev Immunol 15:335–49.
- Datta NR, Ordóñez SG, Gaipl US, et al. (2015). Local hyperthermia combined with radiotherapy and-/or chemotherapy: recent advances and promises for the future. Cancer Treat Rev 41:742–53.
- Roti Roti JL. (2008). Cellular responses to hyperthermia (40–46 degrees C): cell killing and molecular events. Int J Hyperthermia 24:3–15.
- Krawczyk PM, Eppink B, Essers J, et al. (2011). Mild hyperthermia inhibits homologous recombination, induces BRCA2 degradation, and sensitizes cancer cells to poly (ADP-ribose) polymerase-1 inhibition. Proc Natl Acad Sci USA 108:9851–6.
- Jasin M, Rothstein R. (2013). Repair of strand breaks by homologous recombination. Cold Spring Harb Perspect Biol 5:a012740.
- Moynahan ME, Pierce AJ, Jasin M. (2001). BRCA2 is required for homology-directed repair of chromosomal breaks. Mol Cell 7:263–72.
- Tarsounas M, Davies D, West SC. (2003). BRCA2-dependent and independent formation of RAD51 nuclear foci. Oncogene 22:1115–23.
- Thorslund T, West SC. (2007). BRCA2: a universal recombinase regulator. Oncogene 26:7720–30.
- Bryant HE, Schultz N, Thomas HD, et al. (2005). Specific killing of BRCA2-deficient tumours with inhibitors of poly(ADP-ribose) polymerase. Nature 434:913–7.
- Farmer H, McCabe N, Lord CJ, et al. (2005). Targeting the DNA repair defect in BRCA mutant cells as a therapeutic strategy. Nature 434:917–21.
- Saleh-Gohari N, Bryant HE, Schultz N, et al. (2005). Spontaneous homologous recombination is induced by collapsed replication forks that are caused by endogenous DNA single-strand breaks. Mol Cell Biol 25:7158–69.
- Brown JS, Kaye SB, Yap TA. (2016). PARP inhibitors: the race is on. Br J Cancer 114:713–5.
- Alexandrov LB, Nik-Zainal S, Wedge DC, et al. (2013). Signatures of mutational processes in human cancer. Nature 500:415–21.
- van Rhoon GC, Rietveld PJ, van der Zee J. (1998). A 433 MHz Lucite cone waveguide applicator for superficial hyperthermia. Int J Hyperthermia 14:13–27.
- Lowry OH, Rosebrough NJ, Farr AL, Randall RJ. (1951). Protein measurement with the Folin phenol reagent. J Biol Chem 193:265–75.
- Schindelin J, Arganda-Carreras I, Frise E, et al. (2012). Fiji: an open source platform for biological image analysis. Nat Methods 9:676–82.
- Franckena M, Fatehi D, De Bruijne M, et al. (2009). Hyperthermia dose-effect relationship in 420 patients with cervical cancer treated with combined radiotherapy and hyperthermia. Eur J Cancer 45:1969–78.
- Oldenborg S, Griesdoorn V, van Os R, et al. (2015). Reirradiation and hyperthermia for irresectable locoregional recurrent breast cancer in previously irradiated area: Size matters. Radiother Oncol 117:223–8.
- Franckena M, De Wit R, Ansink AC, et al. (2007). Weekly systemic cisplatin plus locoregional hyperthermia: an effective treatment for patients with recurrent cervical carcinoma in a previously irradiated area. Int J Hyperthermia 23:443–50.
- Heijkoop ST, van Doorn HC, Stalpers LJA, et al. (2014). Results of concurrent chemotherapy and hyperthermia in patients with recurrent cervical cancer after previous chemoradiation. Int J Hyperthermia 30:6–10.
- Harnicek D, Kampmann E, Lauber K, et al. (2016). Hyperthermia adds to trabectedin effectiveness and thermal enhancement is associated with BRCA2 degradation and impairment of DNA homologous recombination repair. Int J Cancer 139:467–79.
- Oei AL, Vriend LEM, van Leeuwen CM, et al. (2017). Sensitizing thermochemotherapy with a PARP1-inhibitor. Oncotarget 8:16303–12.
- Oei AL, van Leeuwen CM, Ahire VR, et al. (2017). Enhancing synthetic lethality of PARP-inhibitor and cisplatin in BRCA-proficient tumour cells with hyperthermia. Oncotarget 8:28116–24.
- Oei AL, Ahire VR, van Leeuwen CM, et al. (2017). Enhancing radiosensitisation of BRCA2-proficient and BRCA2-deficient cell lines with hyperthermia and PARP1-i. Int J Hyperthermia. [Epub ahead of print]. doi: 10.1080/02656736.2017.1324642
- Eppink B, Krawczyk PM, Stap J, Kanaar R. (2012). Hyperthermia-induced DNA repair deficiency suggests novel therapeutic anti-cancer strategies. Int J Hyperthermia 28:509–17.
- Guo Z, Pontén F, Wilander E, Pontén J. (2000). Clonality of precursors of cervical cancer and their genetical links to invasive cancer. Mod Pathol 13:606–13.
- Kidd EA, Grigsby PW. (2008). Intratumoral metabolic heterogeneity of cervical cancer. Clin Cancer Res 14:5236–41.
- Vaupel PW, Kelleher DK. (2012). Blood flow and associated pathophysiology of uterine cervix cancers: characterisation and relevance for localised hyperthermia. Int J Hyperthermia 28:518–27.
- Vaughn JP, Cirisano FD, Huper G, et al. (1996). Cell cycle control of BRCA2. Cancer Res 56:4590–4.
- Evans SS, Wang WC, Bain MD, et al. (2001). Fever-range hyperthermia dynamically regulates lymphocyte delivery to high endothelial venules. Blood 97:2727–33.