Abstract
Objectives: To evaluate the clinical performance of a new microwave ablation (MWA) system with enabled constant spatial energy control (ECSEC) to achieve spherical ablation zones in the treatment of liver malignancies.
Materials and methods: In this retrospective study, 56 hepatic tumours in 48 patients (23 men, 25 women; mean age: 59.6 years) were treated using a new high-frequency MWA-system with ECSEC. Parameters evaluated were technical success, technical efficacy, tumour diameter, tumour and ablation volume, complication rate, 90-day mortality, local tumour progression (LTP) at the 12-month follow-up, ablative margin and ablation zone sphericity. These parameters were compared using the Kruskal–Wallis test with the same parameters collected retrospectively from cohorts of patients treated with conventional high-frequency (HF) MWA (n = 20) or low-frequency (LF) MWA (n = 20).
Results: Technical success was achieved in all interventions. The technical efficacy was 100% (ECSEC) vs. 100% (LF-MWA) vs. 95% (HF-MWA). There were no intra-procedural deaths or major complications. Minor complications occurred in 3.57% (2/56), 0% (0/20) and 0% (0/20) of the patients, respectively. The one-year mortality rate was 16.1% (9/56), 15% (3/20) and 10% (2/20), respectively. The LTP was 3.57% (2/56), 5% (1/20) and 5% (1/20), respectively. The median deviation from ideal sphericity (1.0) was 0.135 (ECSEC) vs. 0.344 (LF-MWA) vs. 0.314 (HF-MWA) (p < 0.001). The absolute minimal ablative margin was 8.1 vs. 2.3 vs. 3.1 mm (p < 0.001).
Conclusions: Microwave ablation of liver malignancies is a safe and efficient treatment independent of the system used. Hepatic MWA with ECSEC achieves significantly more spherical ablation zones and higher minimal ablative margins.
Introduction
Hepatocellular carcinoma and liver metastasis from colorectal cancer are counted among the most common hepatic cancers [Citation1]. Surgical treatment remains the treatment of choice for the curative therapy of liver malignancies; however, a considerable number of patients are inoperable [Citation2,Citation3]; consequently, alternative treatments, such as radiofrequency ablation (RFA) [Citation4], microwave ablation (MWA) [Citation5,Citation6], laser-induced-thermotherapy (LITT) [Citation7], cryoablation [Citation8], transarterial chemoembolization (TACE) and stereotactic radiotherapy, have advanced over the last decade. MWA has been shown to combine the benefits of RFA while having its own benefits, namely, higher ablation volumes, shortened treatment duration, possibility of multiple antenna use, reduced susceptibility to the heat-sink effect and higher rates of local tumour control [Citation9–15].
As many primary and secondary hepatic tumours have satellite lesions or irregular extents that often cannot be seen in pre-ablation imaging, high volume ablation and a sufficient ablative margin of active heating (cytotoxic temperatures) all around the index tumour are necessary to ensure complete tumour destruction [Citation16–18]. Wang et al. [Citation19] identified a uniform margin >5 mm all around the tumour in every direction as being essential for local tumour control. Therefore, if ablation does not produce a sufficient margin, it is the primary cause of local tumour progression in terms of a recurrent tumour focus connected to the edge of an ablation zone, which repeatedly has been identified as an independent risk factor for LTP [Citation20–25].
Microwave ablation is known to produce an oval-shaped ablation zone. However, spherical ablation is more beneficial because it may improve the achievement of large, sufficient margins and further the effectiveness of treating lesions that are more than 3 cm in diameter [Citation26,Citation27]. Additionally, spherical ablation zones may minimise the risk of complications associated with the thermal damage of adjacent normal liver tissue, compared with elongated ablation zones [Citation15,Citation26,Citation28].
A recently introduced MWA ablation system with enabled constant spatial energy control (ECSEC) achieves a more spherical ablation zone by providing field and wavelength control [Citation29]. However, the clinical experience with this system is currently scarce.
Thus, the aim of the current study was to evaluate the clinical performance, especially regarding the spherical ablation zones and ablative margin size, of the new MWA system with ECSEC for treating liver malignancies, compared with two conventional and routinely used devices.
Materials and methods
Study design
The current study was approved by the university hospital’s ethical committee, and informed consent was obtained from every patient before ablation. The new MWA technology was evaluated and compared with two different conventional MWA technologies (low-frequency [LF] and high-frequency [HF]), which are further explained later. Due to the retrospective study design, independently of the used MWA technology, all patients had met the following general MWA inclusion criteria: (1) recent MRI studies available and performed within the last two weeks before ablation, (2) hepatic lesions with a maximum axial diameter ≤5 cm, (3) a maximum number of ≤5 lesions and (4) no invasion into the portal venous system, hepatic venous branches or biliary invasion. The exclusion criteria were as follows: (1) lesions with an axial diameter >5 cm, (2) more than five visible lesions, (3) insufficient coagulation (international normalized ratio [INR] > 1.5 or platelets <50,000/µl), and (4) acute or active inflammatory lesions in any organ on preprocedural imaging.
We retrospectively included all patients who underwent MWA with the new FDA-approved system with ECSEC. On this basis, we performed sample-size and determined comparison groups using 20 sessions each, only to identify distinct effects as being statistically significant between the groups. The comparison groups had been treated with conventional LF-MWA and HF-MWA. LF-MWA sessions proceeded chronologically prior to HF-MWA, and consequently, there were no additional criteria between these technologies. ECSEC and HF-MWA were used alternately during the introduction phase (six months) of ECSEC to prevent bias.
Patient characteristics
Retrospectively included were 48 patients (mean age: 59.6 years ± 12.3; range: 25–82 years) who underwent a total of 56 MWA sessions with ECSEC, including 23 men (mean age: 63.4 years ± 12.6; range: 37–82 years) and 25 women (mean age: 57.5 years ± 12.1; range: 35–78 years). We included the last 20 patients (mean age: 61.9 years ± 11.1; range: 42–85 years) treated with LF-MWA (10 men (mean age: 65.9 years ± 12.1; range: 42–85 years) and 10 women (mean age: 57.9 years ± 8.7; range: 43–71 years). The HF-MWA group consisted of the last 20 patients (mean age: 61.7 years ± 12.1; range: 34–83 years), including 9 men (mean age: 65.1 years ± 10.9; range: 50–83 years) and 11 women (mean age: 58.8 years ± 12.7; range: 34–77 years). There were no significant differences among the groups (p = 0.77). A summary of the patient characteristics is presented in .
Table 1. Patients’ data for the different systems used.
Pre-ablation assessment and ablation procedure
For each patient, recent imaging studies were used to assess the number of lesions, lesion size and anatomical position on the day of the examination. For detailed ablation planning, two radiologists, each with more than 10 years of experience in interventional radiology, evaluated the lesions by contrast-enhanced MRI performed with a 1.5-T system (Magnetom Avanto; Siemens Healthcare, Erlangen, Germany) in 5-mm-thick transverse sections. MRI studies had to have been performed within the last two weeks to provide current data.
All interventional procedures were performed by a single team of two radiologists with more than 15 and 20 years of experience in ablation techniques. The patients were continuously monitored for blood pressure, electrocardiography, and pulse oximetry during the procedure. After positioning the patient in a supine position with the arms elevated above the head, a combination of sedative and analgesic medication with diazepam (Diazepam-ratiopharm®; ratiopharm GmbH, Ulm, Germany; 0.1–0.2 mg/kg body weight) and piritramide (Piritramid-hameln®; Hameln Pharma Plus GmbH, Hameln, Germany; 0.2 mg/kg body weight) were administered intravenously. All ablations were performed under CT planning and guidance (Somatom Sensation 64; Siemens, Erlangen, Germany) with the following parameters: 5-mm collimation, 30 mAs, 120 kV, 5-mm section-thickness and activated real-time tube modulation (CARE Dose 4D; Siemens, Erlangen, Germany).
After disinfection of the treatment area, an antenna was inserted percutaneously. The output energy was increased gradually and adapted for the respective level to achieve complete ablation. Interventionists monitored the ablation procedures using repeated CT single-image acquisitions (every minute for the first 3 min; afterwards, every two minutes) to identify possible complications and verify the real-time ablation zone extent (seen as small areas of vaporisation within the tumour). After the completion of MWA, which was determined as CT imaging evidence of complete tumour coverage, the needle track was cauterised by thermal coagulation. The patients were observed for the following eight hours, and in the case of pathological vital signs, control CT was performed; otherwise, the patients were discharged. Following the standardised SIR grading system, major complications were defined as events that lead to substantial morbidity and disability and increase the level of care or that require a distinctively longer hospital stay; all other occurring complications (such as moderate local pain) were determined to be minor [Citation28,Citation30].
Technical features
The used microwave antenna with ECSEC (Emprint™ with Thermosphere™-Technology, Covidien, Dublin, Ireland) was employed with a generator operating at a frequency of 2.45 GHz ± 50 MHz with an output power range from 5 to 100 W. The two technical aspects that ensure more spherical ablation zones are field and wavelength control. Field control is ensured through a new antenna design that directs the location and intensity of electron movements within the antenna, inducing a spherical field shape. The second parameter influencing the ablation zone extent is wavelength, as it determines how intense the water molecules rotate the treated tissue. Therefore, the device provides complete ablation by circulating the fluid surrounding the antenna, to prevent changes in the active antenna wavelength due to coagulation and desiccation during the procedure.
The MWA systems of comparison groups worked with output frequencies of 915 MHz and maximum output power of 35–45 W (Evident™, Covidien, Dublin, Ireland) (LF-MWA) and 2.45 GHz and up to 140 W (Amica™ HS Hospital Service, Aprilia, Italy) (HF-MWA). Each procedure was performed using a single antenna and following the manufacturer’s recommendations regarding the total duration and output energy.
Imaging and follow-up protocol
The first post-procedural imaging was obtained one day after ablation using contrast-enhanced (Gadovist® 1 mmol/ml; Bayer Healthcare, Leverkusen, Germany) MRI. T1- and T2-weighted MRI scans in transverse and sagittal orientation with a 5- or 6-mm slice thickness using a 1.5-T system (Magnetom Avanto; Siemens, Erlangen, Germany) were performed. Additional image series acquired were T1-weighted GE sequences in two planes, transverse contrast-enhanced dynamic series, and T1-weighted post-contrast series. The applied sequence protocol was: diffusion-transverse, HASTE, TSE, FLASH 2D, in phase and opposed phase, and FLASH 2 D dynamic. Further follow-up was obtained after three, six and 12 months and was performed using contrast-enhanced MRI scans.
Definitions and method of measurement
Technical success was defined as a correct intervention accomplishment according to the protocol and the complete coverage of the index tumour through the ablation zone by CT at the end of the ablation.
Technical efficacy was determined as complete coverage of the index tumour through the ablation zone in the first contrast-enhanced MRI 24 h post-ablation and with a maximal thin symmetric rim of peripheral enhancement [Citation30,Citation31].
Tumour recurrences were differentiated in (a) local tumour progression (LTP) and (b) distant recurrence (DR). LTP was defined as a tumour focus connected to the edge of an ablation zone, which was previously considered technically efficient in 24-h post-ablation MRI. DR was determined to be a new intrahepatic lesion in untreated parenchyma or extrahepatic progress [Citation30,Citation32]. Recurrences were evaluated in contrast-enhanced MRI after three, six and 12 months.
For sphericity measurements in MRI studies of the first post-procedural imaging, one-day post-ablation images were used, as they were available for all patients and enabled the best precision due to their image resolution [Citation30]. The MRI data were post-processed for measurement using dedicated software (VolumeViewer™; GE Healthcare, Little Chalfont, UK). Measurements were performed by two radiologists with 5 and 20 years of experience in liver MRI. Most recurrent lesions emerge from an incomplete ablative margin, including cranial and caudal ends [Citation22,Citation23]; consequently, the ablation zone should be measured as comprehensively as possible [Citation30]. Because the extent of the ablation zone roundness can vary distinctly between image slices, the sphericity evaluation in this study was performed by measuring each slice representing an ablation zone section. Two perpendicular ablation zone diameters were measured per slice to obtain their ratio. As this ratio only describes the cross-sectional roundness of the related ablation zone part, this procedure was performed for each image slice in transverse and sagittal planes to provide a three-dimensional evaluation. The average of all related ratios was calculated per single ablation zone to obtain a final sphericity value for each ablation zone. This final value is stated as the deviation from 1.0 (corresponding to an ideal sphere) and not as a sphericity index ≤1 because the results would have been distorted through the average calculation.
The ablative margin size measurement was performed in pre- and post-ablation MRI according to the method previously described by Wang et al. [Citation19]. The method was supplemented by measuring the ablative margin in a second perpendicular plane; consequently, the results are stated as the minimum ablative margin (transverse, sagittal and absolute minimum) per ablation zone. The aim of this approach was to improve the identification and interpretation of the correlation between sphericity and ablative margin size results.
Statistical analysis
The total number of single interventions was categorised into three groups according to the system used (ECSEC, LF-MWA, or HF-MWA). The parameters collected were age, gender, primary tumour origin, lesion location, treatment duration, output energy, technical success and efficacy, complications, one-year mortality, LTP and DR at the 12-month follow-up, tumour diameter, tumour and ablation volume on MRI and minimal ablative margin size. The final value used to compare the morphology was the deviation from 1.0 for the mean of the diameter ratios in every slice representing the ablation zone.
Minimum and maximum values, the confidence interval (0.95) for the median and the 25th and 75th percentiles were assessed. The Kolmogorov–Smirnov test was used to determine whether the measurements were normally distributed. Because the variables were not normally distributed, the Kruskal–Wallis test was used to determine whether differences between pre- and post-MWA tumour measurements were significant. Statistical software (BiAS for Windows, version 10.12) was used, and a p < 0.05 indicated statistical significance.
Results
Technical success and efficacy
Technical success was achieved in all interventions with ECSEC, LF-MWA and HF-MWA. Technical efficacy was reached in 56/56 (100%) ECSEC interventions, in 20/20 (100%) with LF-MWA, and 19/20 (95%) with HF-MWA.
Safety and complications
We recorded no intra-procedural death. The one-year mortality rate after ablation was 16.1% (9/56) in the ECSEC group, 15% (3/20) in the LF-MWA group and 10% (2/20) in the HF-MWA group without significant differences (p = 0.791).
There were no major complications. The minor complication rate was 3.57% (2/56) for ECSEC treatments, 0% (0/20) for LF-MWA and 0% (0/20) for HF-MWA (p = 0.486). Complications occurring with ECSEC were low-grade subcapsular bleeding that did not require treatment and a minimal subcapsular liver abscess.
Index tumour diameter and volume
The mean transverse initial tumour diameter was 13.95 mm (median: 14 mm, range: 4–37 mm) in the ECSEC group, 11.65 mm (median: 12 mm, range: 4–28 mm) in the LF-MWA group and 12.8 mm (median: 12.5 mm, range: 4–26 mm) in the HF-MWA group. Differences between the index tumours were not significant (p = 0.224).
The mean initial tumour volume was 3.38 cm³ (median: 2.17 cm³, range: 0.5–58.38 cm³) for the ECSEC interventions, 1.97 cm³ (median: 1.73 cm³, range: 0.29–6.44 cm³) for LF-MWA and 3.03 cm³ (median: 2.06 cm³, range: 0.29–11.68 cm³) for HF-MWA. There were no significant differences in pre-ablation tumour volumes among the groups (p = 0.426).
Ablation volume
For interventions with ECSEC, the mean ablation volume was 33 cm³ (median: 29.42 cm³, range: 6.76–94 cm³), 18.69 cm³ (median: 14.09 cm³, range: 6.56–43.99 cm³) for LF-MWA and 51.90 cm³ (median: 51.28 cm³, range: 7.34–116.72 cm³) for HF-MWA. The differences in the post-ablation volumes between ECSEC and LF-MWA (p = 0.005), HF-MWA and LF-MWA (p < 0.0001), and ECSEC and HF-MWA (p = 0.014) were all significant.
Ablation time and output energy
The mean duration of ablation was 8.4 min (median: 8.8 min, range: 2.5–20 min) for ECSEC, 10 min (median: 10 min, range: 5–15 min) for LF-MWA, and 10.1 min (median: 10 min, range: 2.5–20 min) for HF-MWA. The difference in duration time between ECSEC and LF-MWA (p = 0.026) and between ECSEC and HF-MWA was significant (p = 0.04).
The cumulated energy output per treatment was 43,952 J (median: 45,600 J, range: 10,800–1,14,000 J) for ECSEC, 37,435 J (median: 27,000 J, range: 13,500–85,200 J) for LF-MWA, and 44,980 J (median: 46,200 J, range: 8550–98,400 J) for HF-MWA. The differences in output energy were not significant (all p ≥ 0.352).
Sphericity
The sphericity evaluation after 24 h resulted in a median deviation from 1.0 for ECSEC () of 0.135 (25th percentile: 0.085, 75th percentile: 0.178), 0.344 (25th percentile: 0.258, 75th percentile: 0.457) for LF-MWA, and 0.314 (25th percentile: 0.264, 75th percentile: 0.394) for HF-MWA. The differences between ECSEC and HF-MWA and LF-MWA ( and ) were all significant (all p < 0.0001); the difference between LF-MWA and HF-MWA was not significant (p = 0.948). illustrates the almost ideal spherical ablation after MWA with ECSEC in consecutive slices.
Figure 1. T1 weighted MRI of a 69-year-old woman showing metastasis of a primary breast carcinoma in liver segment 8 (arrow) measuring 14.1 mm prior to microwave ablation (a). After microwave ablation with ECSEC for 10 min with a cumulative output energy of 52,800 J, an almost ideal spherical ablation zone (deviation from 1.0 for ideal sphericity was 0.065) was detected 24 h post ablation in consecutive T1 weighted unenhanced transverse (b,c) and sagittal (d,e) MR images. Two consecutive slices per plane are shown for a better demonstration of the spherical ablation zone.
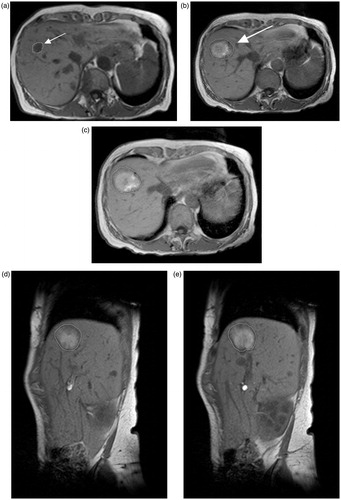
Figure 2. Various ablation zones representing the differences in this study (ECSEC: a–d; HF-MWA: e–h; LF-MWA: i–l). (a) Pre-ablation MR image of the 69-year-old woman from Figure 1 with metastasis of a primary breast carcinoma who underwent microwave ablation ECSEC. T1-weighted axial MR images obtained after 24 h (b) and three months (c) and the CT image after six months (d) show a constant spherical morphology of the ablation zone. (e) The axial T1-weighted MR image shows focal inhomogeneities at a known primary CCC in a 77-year-old woman before thermal MWA therapy. After treatment with HF-MWA, the character of a “tear-drop’’-shaped ablation zone could be detected in the MR follow-up studies after 24 h (f), three months (g) and six months (h). The deviation from the target for the complete ablation zone in this case was 0.374. The third case (i–l) shows a T1-weighted unenhanced MRI of a 43-year-old woman with metastasis of primary renal cell carcinoma in liver segment two measuring 11.3 mm (i). The oval-shaped ablation zone 24 h after microwave treatment with LF-MWA had a diameter measuring 16.3 × 43.3 mm (j). Follow-up studies (k, l) confirmed the oval shape of the ablation zone after three and six months. In each case, technical success and efficacy were achieved, but the minimal ablative margin was larger with ECSEC (trans/sag: b: 10/8.3 mm versus. f: 3.9/5.1 mm versus. j: 4.3/2 mm).
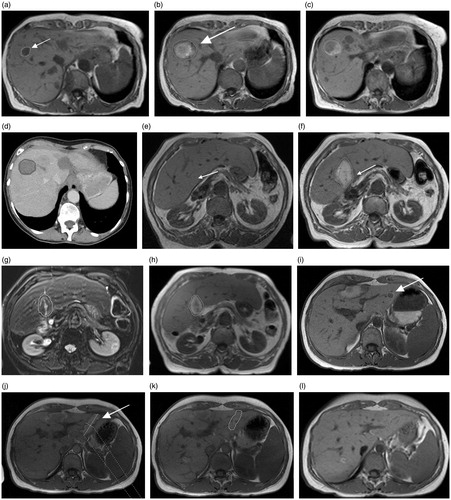
Influence of the primary tumour origin
Primary tumours were classified depending on their origin () for each single intervention. The main three origin locations (breast, colorectal and hepatocellular with 13, 14, and 11 (38/56, others =18) of malignancies treated with ECSEC) were compared regarding sphericity values, to verify whether the primary tumour origin influences the ablation zone sphericity. The differences observed were not significant (all p ≥ 0.086).
Ablative margin and LTP
The minimal ablative margins () showed distinct differences between ECSEC and the other two systems. In the transverse plain, the mean minimal margin in ECSEC was more than double that of the conventional systems (10.8 vs. 4.6 and 4.9 mm). In sagittal measurements, the difference was larger, with a mean margin of 9.1 mm for ECSEC that was 3.4-fold (2.7 mm) that of LF-MWA and 2.3-fold (4 mm) that of the HF-MWA minimal margin. All measurements together resulted in an absolute minimal ablative margin per ablation zone of 8.1 (ECSEC) vs. 2.3 (LF-MWA) and 3.1 mm (HF-MWA). All differences were statistically significant (p < 0.0001) and are detailed in .
Table 2. Minimal ablative margin.
The local tumour progression at the 12-month follow-up was documented in 2/56 (3.57%) of ECSEC, in 1/20 (5%) of LF-MWA and in 1/20 (5%) of HF-MWA interventions. Distant intrahepatic recurrence (DR) within 12 months occurred in 24/56 (42.9%) of ECSEC, 10/20 (50%) of LF-MWA and 8/20 (40%) of HF-MWA interventions. The differences between the groups were not significant (all p > 0.6).
Discussion
The main objective of this study was to evaluate sphericity and the resultant minimal ablative margin. Sphericity results represented by the deviation of the diameters showed significant differences between MW technologies with at least more than 50% less deviation from the target value for ECSEC compared with conventional LF-MWA and HF-MWA. Each procedure was performed with a single antenna. The best previous spherical ablation zone morphologies were achieved using overlapping ablations with multiple antennas. Because the evaluated system achieves nearly spherical ablation, complete ablation can be realised using a single antenna regardless of the angle of approach because the ablation zone extent is similar in all directions, including cranial and caudal ends [Citation22,Citation23,Citation33]. Similar prior evaluations of other MWA concepts were performed using different methods of measurements (but usually not with diameters) and their ratios in every image slice of two perpendicular planes to assess the complete ablation zone. Thus, a comparison of our results with those of prior studies is difficult. Hoffmann et al. [Citation34] reported ex vivo sphericity values of various used systems and features ranging from 0.58 (single antenna) to 0.89 (three simultaneous). Another recently published study [Citation21] showed best circularity values of 0.81 (two antennas) and 0.91 (three antennas) in the ex vivo bovine liver. Our median deviation of 0.135 from 1.0 for comparison can be approximately considered a sphericity/circularity value of 0.865. Thus, although our results cannot be compared optimally, especially due to scarce data from the sphericity evaluation after human MWA, they demonstrate that ECSEC achieved very promising results in human treatments and confirm the ex vivo results.
The measurements of minimal ablative margins showed distinct differences in both planes (). For the overall absolute ablation-zone minimal ablative margin, the study showed a significant larger margin size of 8.1 (ECSEC) vs. 2.3 (LF-MWA) and 3.1 mm (HF-MWA) (p < 0.0001) for comparable patients and index tumour sizes (p = 0.224). The LTP at the 12-month follow-up of 3.57% for ECSEC was in line with previously reported rates after 12 months for comparable MWA systems of 4.2–5.8% [Citation32,Citation33,Citation35]. The results do not often reach the desired size for minimal margin of >1 cm, which previously showed a very low LTP [Citation25,Citation36–38]. However, the median of 7.4 mm for ECSEC reaches the 5–10 mm area, which also showed good results in different studies of thermal ablation [Citation19,Citation37,Citation38]. Kim et al. [Citation38] reported LTP rates of 60% for minimal margins of 1–5 mm and 15% for margins of 5–10 mm. Therefore, for higher validity of the clinical outcomes, relevant LTP studies with a follow-up longer than 12 months and more patients should be performed to identify differences in long-term LTP due to a larger minimal ablative margin between the systems.
As ECSEC is also a type of HF-MWA system, the results indicate that a larger ablation margin is achieved with HF-MWA systems. This fact may result from the general higher ablation volume achieved with HF-MWA and can be supported by lower LTP rates of HF-MWA vs. LF-MWA [Citation35]. Furthermore, this study showed a significant higher ablation volume of conventional HF-MWA vs. ECSEC, but a larger minimal margin of ECSEC. As the index tumours were similar, we refer these results to the more spherical ablation zones achieved with ECSEC. If this is the reason, it would be interesting to evaluate the possibility of sparing the normal liver parenchyma while creating a sufficient margin due to more precise ablation zone formation.
Furthermore, the results of this study demonstrate that all three evaluated MWA systems are safe and efficient. The slightly higher minor complication rate of 3.57% for ECSEC seems to be an effect of group size, as there were no significant differences and the rate was comparable to what is typical for liver MWA [Citation13,Citation15,Citation32].
We also observed a significant reduction in the time required for interventions with ECSEC by 17% (compared with HF-MWA) or 16% (compared with LF-MWA), but notably, in clinical practice, the mean reduction of 2 min is considered trivial with respect to workflow. However, for patients, the reduced time required yields a lower general risk and is more comfortable due to the shorter administration time of sedative medication [Citation15,Citation28].
Certain limitations to this study warrant discussion. First, the assessed patient groups were relatively small. Second, patients with varying hepatic tumour entities were included in this study; a dedicated analysis of this system in a specific tumour subtype would be helpful, particularly because the influence of the primary tumour origin analysis showed a tendency (p ≥ 0.086) to possible influences, although this was not significant. Furthermore, it is noteworthy that only two conventional MWA systems were used for comparison, but there are more than 10 used worldwide. The final aspect is that better results with ECSEC may be related to interventionists’ growing experience in using the systems; however, the interventionists’ overall experience in MWA is far greater than the evaluated groups here and therefore, we assume that there is no significant effect. A further limitation of the study is that we did not evaluate the sphericity index but relied on a more accurate method of assessing the sphericity; hence, a comparison of the current study results with previously published work was not possible.
In conclusion, MWA with new ECSEC is safe and effective for treating hepatic malignancy. Neither the conventional microwave systems nor the ECSEC showed a significant difference regarding the mortality rate of the local tumour progression at the 12-month follow-up. However, the clinical performance of this energy is significantly improved by achieving more spherical ablation zones and larger minimal ablative margins in comparison to the two other routinely used conventional MWA systems.
Disclosure statement
No potential conflict of interest was reported by the authors.
References
- Manfredi S, Lepage C, Hatem C, et al. (2006). Epidemiology and management of liver metastases from colorectal cancer. Ann Surg 244:254–9.
- Hoffmann R, Rempp H, Clasen S. (2012). Microwave tumor ablation. New devices, new applications? Radiologe 52:22–8.
- Tsai S, Pawlik TM. (2009). Outcomes of ablation versus resection for colorectal liver metastases: are we comparing apples with oranges? Ann Surg Oncol 16:2422–8.
- Nishikawa H, Kimura T, Kita R, Osaki Y. (2013). Radiofrequency ablation for hepatocellular carcinoma. Int J Hyperthermia 29:558–68.
- Amabile C, Ahmed M, Solbiati L, et al. (2017). Microwave ablation of primary and secondary liver tumours: ex vivo, in vivo, and clinical characterisation. Int J Hyperthermia 33:34–42.
- Meloni MF, Chiang J, Laeseke PF, et al. (2017). Microwave ablation in primary and secondary liver tumours: technical and clinical approaches. Int J Hyperthermia 33:15–24.
- Wichmann JL, Beeres M, Borchard BM, et al. (2014). Evaluation of MRI T1-based treatment monitoring during laser-induced thermotherapy of liver metastases for necrotic size prediction. Int J Hyperthermia 30:19–26.
- Rong G, Bai W, Dong Z, et al. (2015). Long-term outcomes of percutaneous cryoablation for patients with hepatocellular carcinoma within Milan criteria. PLoS One 10:e0123065.
- Fan W, Li X, Zhang L, et al. (2012). Comparison of microwave ablation and multipolar radiofrequency ablation in vivo using two internally cooled probes. AJR Am J Roentgenol 198:W46–50.
- Ierardi AM, Floridi C, Fontana F, et al. (2013). Microwave ablation of liver metastases to overcome the limitations of radiofrequency ablation. Radiol Med 118:949–61.
- Zhang XG, Zhang ZL, Hu SY, Wang YL. (2014). Ultrasound-guided ablative therapy for hepatic malignancies: a comparison of the therapeutic effects of microwave and radiofrequency ablation. Acta Chir Belg 114:40–5.
- Vogl TJ, Farshid P, Naguib NN, et al. (2014). Thermal ablation of liver metastases from colorectal cancer: radiofrequency, microwave and laser ablation therapies. Radiol Med 119:451–61.
- Liu Y, Li S, Wan X, et al. (2013). Efficacy and safety of thermal ablation in patients with liver metastases. Eur J Gastroenterol Hepatol 25:442–6.
- Correa-Gallego C, Fong Y, Gonen M, et al. (2014). A retrospective comparison of microwave ablation vs. radiofrequency ablation for colorectal cancer hepatic metastases. Ann Surg Oncol 21:4278–83.
- Liang P, Wang Y, Yu X, Dong B. (2009). Malignant liver tumors: treatment with percutaneous microwave ablation–complications among cohort of 1136 patients. Radiology 251:933–40.
- Ierardi AM, Mangano A, Floridi C, et al. (2015). A new system of microwave ablation at 2450 MHz: preliminary experience. Updates Surg 67:39–45.
- Hines-Peralta AU, Pirani N, Clegg P, et al. (2006). Microwave ablation: results with a 2.45-GHz applicator in ex vivo bovine and in vivo porcine liver. Radiology 239:94–102.
- Livraghi T, Goldberg SN, Lazzaroni S, et al. (2000). Hepatocellular carcinoma: radio-frequency ablation of medium and large lesions. Radiology 214:761–8.
- Wang X, Sofocleous CT, Erinjeri JP, et al. (2013). Margin size is an independent predictor of local tumor progression after ablation of colon cancer liver metastases. Cardiovasc Intervent Radiol 36:166–75.
- Park MJ, Kim YS, Rhim H, et al. (2011). A comparison of US-guided percutaneous radiofrequency ablation of medium-sized hepatocellular carcinoma with a cluster electrode or a single electrode with a multiple overlapping ablation technique. J Vasc Interv Radiol 22:771–9.
- Harari CM, Magagna M, Bedoya M, et al. (2016). Microwave ablation: comparison of simultaneous and sequential activation of multiple antennas in liver model systems. Radiology 278:95–103.
- Nakazawa T, Kokubu S, Shibuya A, et al. (2007). Radiofrequency ablation of hepatocellular carcinoma: correlation between local tumor progression after ablation and ablative margin. AJR 188:480–8.
- Liu CH, Arellano RS, Uppot RN, et al. (2010). Radiofrequency ablation of hepatic tumours: effect of post-ablation margin on local tumour progression. Eur Radiol 20:877–85.
- Zytoon AA, Ishii H, Murakami K, et al. (2007). Recurrence-free survival after radiofrequency ablation of hepatocellular carcinoma. A registry report of the impact of risk factors on outcome. Jpn J Clin Oncol 37:658–72.
- Sotirchos VS, Petrovic LM, Gonen M, et al. (2016). Colorectal cancer liver metastases: biopsy of the ablation zone and margins can be used to predict oncologic outcome. Radiology 280:949–59.
- Thamtorawat S, Hicks RM, Yu J, et al. (2016). Preliminary outcome of microwave ablation of hepatocellular carcinoma: breaking the 3-cm barrier? J Vasc Interv Radiol 27:623–30.
- Mulier S, Ni Y, Jamart J, et al. (2005). Local recurrence after hepatic radiofrequency coagulation: multivariate meta-analysis and review of contributing factors. Ann Surg 242:158–71.
- Smolock AR, Lubner MG, Ziemlewicz TJ, et al. (2015). Microwave ablation of hepatic tumors abutting the diaphragm is safe and effective. AJR 204:197–203.
- Alonzo M, Bos A, Bennett S, Ferral H. (2015). The emprint ablation system with thermosphere technology: one of the newer next-generation microwave ablation technologies. Semin Intervent Radiol 32:335–8.
- Ahmed M, Solbiati L, Brace CL, et al. (2014). Image-guided tumor ablation: standardization of terminology and reporting criteria – a 10-year update. Radiology 273:241–60.
- Goldberg SN, Grassi CJ, Cardella JF, et al. (2005). Image-guided tumor ablation: standardization of terminology and reporting criteria. Radiology 235:728–39.
- Xu Y, Shen Q, Liu P, Xu Z, Wu P, Lu Z, et al. (2017). Microwave ablation for the treatment of hepatocellular carcinoma that met up-to-seven criteria: feasibility, local efficacy and long-term outcomes. Eur Radiol. [Epub ahead of print]. doi: 10.1007/s00330-017-4740-0.
- Yu J, Liang P, Yu XL, et al. (2015). Local tumour progression after ultrasound-guided microwave ablation of liver malignancies: risk factors analysis of 2529 tumours. Eur Radiol 25:1119–26.
- Hoffmann R, Rempp H, Erhard L, et al. (2013). Comparison of four microwave ablation devices: an experimental study in ex vivo bovine liver. Radiology 268:89–97.
- Vogl TJ, Hagar A, Nour-Eldin NA, et al. (2016). High-frequency versus low-frequency microwave ablation in malignant liver tumours: evaluation of local tumour control and survival. Int J Hyperthermia 32:868–75.
- Lee HY, Rhim H, Lee MW, et al. (2013). Early diffuse recurrence of hepatocellular carcinoma after percutaneous radiofrequency ablation: analysis of risk factors. Eur Radiol 23:190–7.
- Shady W, Petre EN, Gonen M, et al. (2016). Percutaneous radiofrequency ablation of colorectal cancer liver metastases: factors affecting outcomes – a 10-year experience at a single center. Radiology 278:601–11.
- Kim YS, Lee WJ, Rhim H, et al. (2010). The minimal ablative margin of radiofrequency ablation of hepatocellular carcinoma (>2 and <5 cm) needed to prevent local tumor progression: 3D quantitative assessment using CT image fusion. AJR Am J Roentgenol 195:758–65.