Abstract
Purpose: This study evaluated the characteristics of a thyroid-dedicated bipolar RF electrode (BRFE) and compared its ablation performance with that of monopolar RF electrodes (MRFEs) in normal bovine liver blocks.
Methods: BRFE was tested on 60 bovine liver blocks with six different time–power combinations, applying 20, 30 and 40 W for 60 and 120 s. Subsequently, BRFE and MRFEs with 0.5-, 0.7- and 1-cm active tips were applied on 160 bovine liver blocks, creating 16 time–electrode combinations (10, 30, 60 and 120 s). The ablation characteristics, RF efficacy and true RF efficacy of each electrode group were then evaluated and compared. True RF efficacy was defined as the total ablation volume created within the total time during which valid RF current was generated.
Results: The true RF efficacy of BRFE with 30 W at 60 s was significantly higher than that of BRFE with 20 W and 40 W during the preliminary experiment (p = 0.011). BRFE showed larger vertical and transverse diameters (DT1, DT2) than MRFE with a 0.5-cm active tip (all p values < 0.001, except 10 s). By contrast, MRFE with a 0.7-cm active tip created a larger DT1, DT2, volume, efficacy and true efficacy than BRFE (maximum p value = 0.011). The shape ratio of BRFE was significantly higher than that of MRFEs with 0.5-cm and 0.7-cm tips (all p < 0.001).
Conclusions: Application of BRFE with 30 W could achieve a median ablation volumes and efficacy between that of MRFEs with 0.5-cm and 0.7-cm active tips and created a more ellipsoid-shaped ablation zone.
Introduction
Radiofrequency (RF) ablation has been successfully used as a minimally invasive technique to treat benign thyroid nodules and recurrent thyroid cancers [Citation1–6]. Monopolar RF electrode (MRFE) has been mainly used in thyroid RF ablation, despite their disadvantages due to the use of grounding pads as well as the treating electrode. Specifically, MRFE may induce skin burns at the patch site of the grounding pad or interfere with implanted electrical devices, including pacemakers and implantable cardioverter defibrillators (ICDs) [Citation7–9]. To avoid these complications, bipolar RF electrode (BRFE) has been introduced as a new treatment tool [Citation10]; in this method, an electric current is generated in a limited zone between the two poles of the electrode by integrating the anode and cathode within a single electrode [Citation11–14]. Therefore, BRFE can simplify the ablation procedure as grounding pads are not required, and also relieving pain, and reducing heat injury to surrounding tissues. Moreover, localisation of the electric current in BRFE may allow safer RF ablation in patients with implanted electrical devices, such as pacemakers and ICDs, and in pregnant and lactating women needing treatment for thyroid nodules [Citation13,Citation15,Citation16]. Also, limiting the electric current to the area surrounding the BRFE will result in ablation zones of more predictable size and shape. However, because BRFE produces high current density between the two electrodes on the same needle, a rapid increase in temperature and tissue boiling may result in tissue charring. To overcome the issue of charring, internal cooling of the probe as in MRFE is adapted in some BRFE [Citation17].
According to previous studies, RF ablation using BRFE has been reported effective in the treatment of liver cancers [Citation18]. Meanwhile, recent studies have reported that BRFE can be an effective and safe treatment method for benign thyroid nodules [Citation12–14]. However, previous studies focussed on clinical aspects, such as comparing follow-up results of treated and control groups, rather than comparing the ablation performance of BRFE and MRFE. In addition, the electrodes used in earlier studies were of relatively large diameter (15–15.5 gauge) or had no internal cooling system [Citation17]. For these reasons, we developed a prototype of thyroid-dedicated BRFE, with a combined 17 and 18 gauge, a modified internal cooling system and a 5.5-mm active tip. This is the first study that is carried out after the development of this new thyroid-dedicated BRFE. This study assessed the ablation characteristics of the thyroid-dedicated BRFE by comparing it with the characteristics of MRFEs, thereby establishing a basis for selecting electrodes according to the size of thyroid nodules. Therefore, this study evaluated the characteristics of a thyroid-dedicated BRFE and compared its ablation performance with that of MRFEs in normal bovine liver blocks.
Materials and methods
This ex vivo study was approved by the Institutional Animal Care and Use Committee of Asan Medical Center. This study was supported by The STARmed Co. (Goyang, Korea) which provided and developed the required equipment including the RF generator, monitoring devices and electrodes. Before performing this study, we developed a prototype of thyroid-dedicated BRFE, consisting of a 17-gauge anode, an 18-gauge cathode, and connecting portion via insulation, a modified internal cooling system with a 5.5-mm active tip. The tip has a ratio of 5.5:2:8, to the active electrode (cathode), the connecting portion with insulation, and the return electrode (anode), respectively (). This difference in ratio of the cathode and anode to the electrode tip was determined after preliminary tests that sought to make this BRFE comparable to an MRFE with a 0.8-cm active tip as a target. The combined 17- and 18-gauge structure was aimed to achieve sufficient internal cooling flow through overlap.
Figure 1. Photographs of thyroid-dedicated bipolar electrode in this study. (A) Structure of bipolar electrode that contains modified internal cooling system and the tip containing a pair of electrodes on the same needle. (B) A magnified photograph of the electrode tip that has a ratio of 5.5:2:8 for cathode, connecting portion with insulation, and anode, respectively.
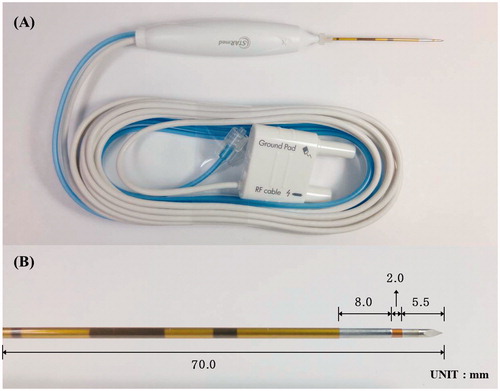
Experimental setup
RF ablation was performed ex vivo in freshly excised bovine liver blocks using BRFE and MRFEs. The preliminary experiment evaluated the ablation characteristics of BRFE, including volume and efficacy for a range of treatment durations and power settings. Subsequently, the ablation characteristics of BRFE and BRFE were compared, including volume and efficacy, according to serial ablation time. The liver was exposed to room temperature for more than 2 h. The tissue temperature before ablation ranged from 22 °C to 26 °C. The livers were cut into 6 × 6×6-cm3 blocks [Citation19]. The tip of the electrode was positioned to a depth of 2 cm in each liver block, and the electrode was fixed during the ablation. RF ablation was feasible in all ex vivo studies.
Preliminary experiment of BRFE ablation power
Preliminary experiments were performed to evaluate the ablation characteristics and volume along with power increments, thereby determining the optimal ablation power for BRFE, resulting in superior ablation efficacy. Superior ablation efficacy was determined by comparing the size of the ablation zone and the duration of true RF time, with optimum RF power creating a larger ablation zone with longer true RF time. The combined 17- and 18-gauge modified internally cooled BRFE (VIVA, STARmed, Goyang, Korea) with a 7-cm shaft length and a 5.5-mm active tip was used. The RF generator (VIVA RF generator, STARmed, Goyang, Korea) delivered three output powers [20, 30 and 40 W] for 60 and 120 s, constituting six time–power combinations. Ten liver blocks were subjected to each time–power combination, for a total of 60 measurements. All the technical parameters, including temperature, impedance and current, in the tissue were measured and documented using VIVA Monitor Software V 1.0 (STARmed, Goyang, Korea). All specimens were examined to determine whether the BRFE produces reproducible ablation lesions, defined as continually connected lesion margins through the entire ablation zone without partial confluent or separated ablation area. Charring was also recorded after finishing each ablation. Moreover, true RF time, defined as the total valid time when RF current was generated per ablation unit, was measured. This parameter was programmed to simulate the condition of moving-shot technique, as drops in RF current and sudden elevations of impedance can be detected at a certain time using the fixed electrode method [Citation19,Citation20].
RF ablation using BRFE and MRFEs
The BRFE described above was compared with MRFEs having an 18 gauge, modified internally cooled system, a shaft 7 cm in length with 0.5-, 0.7- and 1-cm active tips (VIVA, STARmed, Goyang, Korea) [Citation4,Citation6,Citation21]. These tip lengths were selected because this study sought to determine whether BRFE can achieve results comparable to commercially available MRFEs, widely used for recent thyroid RF ablation. Ablation using each MRFE was performed for four time units (10, 30, 60 and 120 s) and 15, 30 and 50 W of RF power. By comparison, BRFE ablation was performed using the power determined from the preliminary experiment with a pulsing mode. Thus, there were 16 time–electrode combinations. Each time–electrode combination was tested on 10 liver blocks, resulting in a total of 160 measurements. An RF generator (VIVA RF generator, STARmed, Goyang, Korea) and a peristaltic pump (VIVA pump, STARmed, Goyang, Korea) were used. The pump continuously infused the cold saline solution (0 °C) into the lumen of the electrodes to maintain the electrode shaft temperature < 20 °C. During ablation with MRFEs, a dispersive metallic pad (20 × 15 cm2) was attached to the lateral wall of the bath in the MRFE group. Tissue impedance was measured after insertion of the electrode, and temperature, impedance, and current in the tissue were measured throughout the entire ablation time and true RF time in all cases. Charring was also recorded after each ablation. As ablation volume plateaus at ablation times >120 s [Citation19], the ablation duration in this study was limited to 120 s.
Measurement of ablation size, volumes, efficacy and shape
After finishing ablation, the liver blocks were cut along the longitudinal plane (L-plane), defined as that passing through the long axis of the electrode. Each block was sliced transversely and perpendicular to the L-plane at the centre of the ablation zone (transverse plane, T-plane). The vertical diameter (DV) along the electrode, in the L-plane, the transverse diameter (DT1) perpendicular to the DV in the L-plane and the other transverse diameter (DT2) in the T-plane were measured by consensus of two researchers, with 5 and 10 years of experience in RFA experiments [Citation22]. The slices were photographed using a digital camera (Nikon Coolpix S6900; Nikon Inc., Tokyo, Japan) alongside a ruler on a copy stand to prevent any measurement bias. The ablated white area was defined as the ablation zone, which reportedly corresponds to the zone of coagulation necrosis [Citation23–26]. The volume of the ablation zone was approximated using the formula [Citation27]: volume = π × (DV × DT1 × DT2)/6 [28]. Ablation efficacy was calculated using the formula: ablation efficacy = ablation volume (mL)/total RF time (s); and true ablation efficacy was calculated using the formula: true ablation efficacy = ablation volume (mL)/true RF time (s), which refers that the total ablation volume created within the total time when valid RF current was generated. The shape of the ablation zone was determined by calculating the ratio between the DV and the mean of the DT1 and DT2 (DV/[(DT1 + DT2)/2]) [Citation17].
Statistical analysis
All statistical analyses were performed using SPSS-PC, version 12.0 (SPSS Inc., Chicago, IL). Quantitative results are presented as the mean ± standard deviation. Measured values of variables were compared using the Kruskal–Wallis test, i.e. impedance before and during the ablation, DV, DT1, DT2, ablation volume and efficacy, in the BRFE and MRFE groups, and the post hoc test with Bonferroni’s correction if warranted. Otherwise, a p value < 0.05 was considered statistically significant.
Results
Preliminary experiment of the BRFE
RF power of 30 W created the largest ablation zone, as determined by DV and DT1 at 60 and 120 s and volume at 60 s, although none of the differences was statistically significant. The true RF efficacy of 30 W at 60 s was significantly higher than other two RF powers (p = 0.011). The RF power of 40 W created smaller DV, DT1, DT2, ablation volume and true efficacy than the other two RF powers. Based on these results, an RF power of 30 W was selected as appropriate for BRFE, as its ablation characteristics, including ablation efficacy for all time–power combinations were significantly superior to those of the other RF powers. Charring did not occur in any of the 60 blocks. All specimens showed reproducible ablation lesions without technical failure, partial confluence or separated ablation area. Ablation parameters, including the comparison of results of RF powers of 20, 30 and 40 W at 60 and 120 sec, are summarised in , and a cross-sectional view of each time–power combination is illustrated in (see Supplementary Appendices 1 and 2 for details of other quantitative parameters).
Figure 2. The cross-sectional views of each time–power combination in the experiment for the bipolar electrode. Ablation zone of corresponding powers (row) for corresponding times (column).
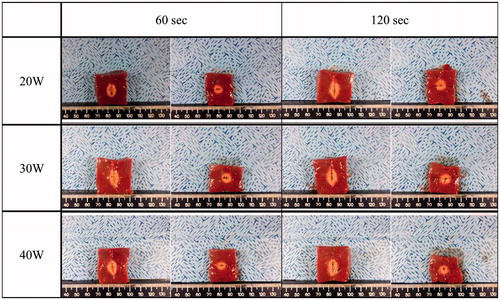
Table 1. Preliminary experiment data of the bipolar electrode.
Comparison of BRFE and MRFE
summarises the comparison result of ablation volumes, true efficacy and shape ratio of BRFE and MRFEs. A cross-sectional view of each time–electrode combination is illustrated in (see Supplementary Appendices 3 and 4 for details of other quantitative parameters). Considering the result of preliminary experiment, we adopted the RF power of 30 W for BRFE for the comparison. Compared with MRFE with a 0.5-cm active tip, BRFE showed significantly larger DV, DT1 and DT2 at all time points (all p values < 0.001), except for DT1 and DT2 at 10 s. The volume, efficacy and true efficacy were also higher in BRFE than in MRFE with a 0.5-cm active tip. Compared with MRFE with a 0.7-cm active tip, BRFE achieved a significantly larger DV at all four time points. Conversely, MRFE with a 1-cm active tip achieved significantly larger DV, DT1, DT2, volume, efficacy and true efficacy than BRFE at all time points (maximum p values = 0.003), except for DT1 and DT2 at 10 s (). The shape ratio of BRFE was significantly higher than that of MRFEs with 0.5-cm and 0.7-cm active tips at all time points, indicating that use of the BRFE resulted in a more elliptical and, spindle shaped ablation zone than use of the MRFEs (p < 0.001). BRFE also showed a significantly higher shape ratio at 60 and 120 s, but not at 30 s, than MRFE with a 1-cm active tip (p < 0.001). All specimens showed reproducible ablation lesions without technical failure, partial confluence or separated ablation except for MRFE with a 1-cm active tip at 10 s. As the ablation lesion at this time–electrode combination was partially confluent, we used the mean of the minimum and maximum transverse diameters for DT1 and DT2, although it may have affected the statistical results. Charred areas were observed in only four liver blocks ablated of MRFE with a 0.5-cm active tip.
Figure 3. The cross-sectional views of each time–electrode combinations in comparison experiments between bipolar and monopolar electrodes. Ablation zone of corresponding BRFE and each MRFE tips (0.5, 0.7 and 1 cm) (row) for corresponding time (column). BRFE: bipolar RF electrode; MRFE: monopolar RF electrode.
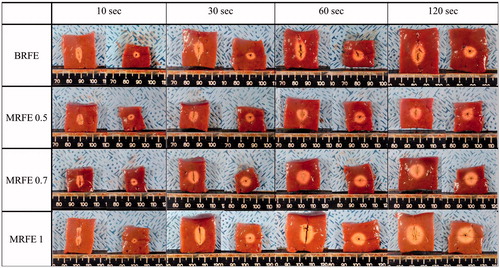
Table 2. Comparison data of the bipolar and monopolar electrodes.
Discussion
The present ex vivo study demonstrated that an RF power of 30 W can be applied for the thyroid-dedicated BRFE and that the ablation volume and efficacy of BRFE were median value between those of MRFEs with 0.5-cm and 0.7-cm active tips. BRFE generated a more ellipsoid, spindle-shaped lesion than MRFEs. All specimens ablated by BRFE showed reproducible ablation lesions without charring. Collectively, these results indicate that the thyroid-dedicated BRFE can be used as complementary to MRFEs with 0.5-cm or 0.7-cm active tips and may form different shaped ablation zones.
Our preliminary experiment showed that an RF power of 30 W showed the highest true RF efficacy at 60 s among the three RF powers tested for BRFE (p = 0.011). By contrast, an RF power of 40 W created smaller ablation diameters and true efficacy than the other two RF powers. These results may suggest that application of higher RF power results in more frequent occurrence of a roll-off phenomenon, which interferes with effective conduction of the ablation power [Citation19]. This may have been responsible for the superior true efficacy using an RF power of 30 W. Moreover, application of higher RF power during RF ablation using the moving-shot technique may be more burdensome. Consequently, an RF power of 30 W may be the most appropriate power setting for thyroid-dedicated BRFE.
In terms of ablation size, thyroid-dedicated BRFE created significantly larger ablation lesions than MRFE with a 0.5-cm active tip. This result can be attributed to the basic difference between BRFE and MRFE in their generation of electrical current flows. BRFE is able to drive a high density current between a pair of electrodes located on the same needle, resulting in faster and more focal heating between the electrodes. By contrast, the current in MRFE spreads from each electrode centrifugally to the periphery. However, the ablation zone volume was smaller in BRFE than that of MRFE with a 0.7-cm active tip. This may have been caused by the larger diameters in DT1 and DT2 of MRFEs, which created more spherical-shaped lesions. However, because our experiment was performed at room temperature, an in vivo study performed at body temperature may generate different results.
The current Korean guidelines for thyroid RF suggest using the moving-shot technique for RF ablation of benign thyroid nodules [Citation5,Citation19,Citation29]. Unlike the liver, the thyroid gland is a small organ, whereas thyroid nodules are relatively large and often have exophytic and ellipsoidal shape. There is also concern about the critical structures surrounding thyroid nodules including the oesophagus, trachea and recurrent laryngeal nerve, making ablation of thyroid nodules difficult using a fixed technique. Therefore, it is advantageous to treat a thyroid nodule divided into multiple small ablation units, which can be treated using the moving shot technique, which involves rapid repositioning of the electrode during the procedure [Citation19]. The results of our study suggest that multiple repositioning of the RF electrode with elaborate shaping may be necessary to generate a fully covered coagulation volume using the thyroid dedicated BRFE compared with MRFE with a 0.7-cm active tip when applying moving shot technique. However, because the ablation zone of BRFE had an elongated spindle-shaped configuration with a smaller volume than MRFE with a 0.7-cm active tip, BRFE may allow more precise handling, particularly when ablating the residual portion of a previously treated nodule, reducing heat injury to surrounding critical structures and allowing the procedure to be performed easily, even by inexperienced physicians [Citation30].
The mechanism of BRFE has several advantages over MRFE. First, the BRFE avoids the risk of skin burn on ground pad. In addition, as the electric current is only localised around the electrode tip, BRFE may be used safely in patients with pacemakers or in pregnant women [Citation10,Citation13]. Further, the higher energy density generated by a localised electric current accumulates around the electrode tip, which may shorten procedure time compared with MRFE [Citation10]. Moreover, localising the electrical current to a limited zone surrounding the BRFE may result in a more predictable size and shape of the ablation zone, which was also confirmed in our results. However, the BRFE ablation zones tend to be ellipsoid in shape, as well as being smaller in area than ablation zones generated by the MRFE with a 0.7-cm active tip [Citation17].
Recently, BRFE has been used to treat thyroid nodules [Citation13–15,Citation30,Citation31]. As in the development of MRFE, researchers have attempted to overcome tissue desiccation and charring at the electrode tip because of the increased current density between the two electrodes in BRFE. Although a BRFE equipped with an internal cooling system may reduce possible shortcomings and increase both electrical conductance and thermal conductivity, these BRFEs have a relatively large diameter (15–15.5 gauge) or are customised for hepatic RFA [Citation14,Citation17,Citation32]. Therefore, the BRFE described in this study has a relatively thin calibre while retaining the internal cooling system, making it suitable for thyroid nodules. This modification may have contributed to the superior results obtained with the BRFE than with the MRFE using a 0.5-cm active tip in our study and with a previous report using a BRFE with a 0.9-cm active tip without an electrode cooling system [Citation17].
This study had several limitations. First, this study was performed using bovine liver tissue. Differences between liver and thyroid tissues may have yielded different results. However, it is difficult to obtain satisfactory results when using porcine thyroid glands due to their small size and commercial unavailability. Also, several previous ex vivo studies validating electrodes for thyroid RFA or microwave ablation were performed using bovine liver blocks [Citation19,Citation28,Citation33]. Finally, we decided to use the bovine liver block for the experimental material in this study. The second possible limitation was our use of a fixed electrode position rather than applying a moving-shot technique. To overcome this limitation, we assessed true RF time and true RF efficacy, which simulate experimental conditions of the moving-shot technique. However, because our experiments were performed at room temperature, which ranged from 22 °C to 26 °C, further prospective in vivo studies using the moving-shot technique are necessary to ensure that the ablation performance of BRFE is both accurate and satisfactory. A third limitation was our macroscopic measurement of ablation zones on cross-sectional views without histological inspection. Additionally, we only used a thyroid-dedicated BRFE with a 7-cm length and a tip ratio of 5.5:2:8. Using BRFEs of different lengths may have yielded different results. Finally, a small bump at the insulation part of BRFE may limit electrode manipulation during RF ablation.
Conclusions
In summary, we developed a thyroid dedicated BRFE, determined its optimal power setting and compared this BRFE with MRFEs. Thyroid dedicated BRFE yielded optimal results at an RF power of 30 W. Compared with MRFEs with 0.5-cm and 0.7-cm active tips, BRFE achieved median ablation volumes and efficacy without charring and created a more ellipsoid-shaped ablation zone in an ex vivo bovine liver model.
Acknowledgements
The authors wish to thank Dong Un Kim, BA and Jung Hyuk Zu, BA for his contribution for technical assistance for radiofrequency ablation experiments.
Disclosure statement
Jung Hwan Baek is a consultant of STARmed and RF medical company since 2017. Other authors report no conflicts of interest. The authors alone are responsible for the content and writing of the paper.
References
- Ha SM, Sung JY, Baek JH, et al. (2017). Radiofrequency ablation of small follicular neoplasms: initial clinical outcomes. Int J Hyperthermia 33:931–7.
- Kim JH, Baek JH, Sung JY, et al. (2017). Radiofrequency ablation of low-risk small papillary thyroidcarcinoma: preliminary results for patients ineligible for surgery. Int J Hyperthermia 33:212–9.
- Lim HK, Baek JH, Lee JH, et al. (2015). Efficacy and safety of radiofrequency ablation for treating locoregional recurrence from papillary thyroid cancer. Eur Radiol 25:163–70.
- Baek JH, Kim YS, Lee D, et al. (2010). Benign predominantly solid thyroid nodules: prospective study of efficacy of sonographically guided radiofrequency ablation versus control condition. AJR Am J Roentgenol 194:1137–42.
- Baek JH, Lee JH, Valcavi R, et al. (2011). Thermal ablation for benign thyroid nodules: radiofrequency and laser. Korean J Radiol 12:525–40.
- Jeong WK, Baek JH, Rhim H, et al. (2008). Radiofrequency ablation of benign thyroid nodules: safety and imaging follow-up in 236 patients. Eur Radiol 18:1244–50.
- Chung SR, Suh CH, Baek JH, et al. (2017). Safety of radiofrequency ablation of benign thyroid nodules and recurrent thyroid cancers: a systematic review and meta-analysis. Int J Hyperthermia 33:920–30.
- Tong NY, Ru HJ, Ling HY, et al. (2004). Extracardiac radiofrequency ablation interferes with pacemaker function but does not damage the device. Anesthesiology 100:1041.
- Bernardi S, Stacul F, Zecchin M, et al. (2016). Radiofrequency ablation for benign thyroid nodules. J Endocrinol Invest 39:1003–13.
- Park HS, Baek JH, Park AW, et al. (2017). Thyroid radiofrequency ablation: updates on innovative devices and techniques. Korean J Radiol 18:615–23.
- Ahmed M, Brace CL, Lee FT, Jr., et al. (2011). Principles of and advances in percutaneous ablation. Radiology 258:351–69.
- Kohlhase KD, Korkusuz Y, Groner D, et al. (2016). Bipolar radiofrequency ablation of benign thyroid nodules using a multiple overlapping shot technique in a 3-month follow-up. Int J Hyperthermia 32:511–6.
- Korkusuz Y, Erbelding C, Kohlhase K, et al. (2016). Bipolar radiofrequency ablation of benign symptomatic thyroid nodules: initial experience. Rofo 188:671–5.
- Li XL, Xu HX, Lu F, et al. (2016). Treatment efficacy and safety of ultrasound-guided percutaneous bipolar radiofrequency ablation for benign thyroid nodules. Br J Radiol 89:20150858.
- Osaki Y, Ikeda K, Izumi N, et al. (2013). Clinical effectiveness of bipolar radiofrequency ablation for small liver cancers. J Gastroenterol 48:874–83.
- Sadoul N, Blankoff I, de Chillou C, et al. (1997). Effects of radiofrequency catheter ablation on patients with permanent pacemakers. J Interv Card Electrophysiol 1:227–33.
- Bruners P, Lipka J, Gunther RW, et al. (2008). Bipolar radiofrequency ablation: is the shape of the coagulation volume different in comparison to monopolar RF-ablation using variable active tip lengths? Minim Invasive Ther Allied Technol 17:267–74.
- Baldwin K, Katz SC, Rubin A, et al. (2012). Bipolar radiofrequency ablation of liver tumors: technical experience and interval follow-up in 22 patients with 33 ablations. J Surg Oncol 106:905–10.
- Ha EJ, Baek JH, Lee JH. (2014). Moving-shot versus fixed electrode techniques for radiofrequency ablation: comparison in an ex-vivo bovine liver tissue model. Korean J Radiol 15:836–43.
- Ahn HS, Kim SJ, Park SH, et al. (2016). Radiofrequency ablation of benign thyroid nodules: evaluation of the treatment efficacy using ultrasonography. Ultrasonography 35:244–52.
- Baek JH, Moon WJ, Kim YS, et al. (2009). Radiofrequency ablation for the treatment of autonomously functioning thyroid nodules. World J Surg 33:1971–7.
- Ahmed M, Solbiati L, Brace CL, et al. (2014). Image-guided tumor ablation: standardization of terminology and reporting criteria-a 10-year update. Radiology 273:241–60.
- Clasen S, Schmidt D, Dietz K, et al. (2007). Bipolar radiofrequency ablation using internally cooled electrodes in ex vivo bovine liver: prediction of coagulation volume from applied energy. Invest Radiol 42:29–36.
- Lee JD, Lee JM, Kim SW, et al. (2001). MR imaging-histopathologic correlation of radiofrequency thermal ablation lesion in a rabbit liver model: observation during acute and chronic stages. Korean J Radiol 2:151–8.
- Lee JM, Han JK, Kim HC, et al. (2007). Switching monopolar radiofrequency ablation technique using multiple, internally cooled electrodes and a multichannel generator: ex vivo and in vivo pilot study. Invest Radiol 42:163–71.
- Lee JM, Han JK, Kim HC, et al. (2007). Multiple-electrode radiofrequency ablation of in vivo porcine liver: comparative studies of consecutive monopolar, switching monopolar versus multipolar modes. Invest Radiol 42:676–83.
- Lee JM, Han JK, Kim SH, et al. (2004). Comparison of wet radiofrequency ablation with dry radiofrequency ablation and radiofrequency ablation using hypertonic saline preinjection: ex vivo bovine liver. Korean J Radiol 5:258–65.
- Na DG, Lee JH, Kim SM, et al. (2011). Unidirectional ablation electrode to minimize thermal injury during radiofrequency ablation: an experimental study in an ex vivo bovine liver model. J Vasc Interv Radiol 22:935–40.
- Na DG, Lee JH, Jung SL, et al. (2012). Radiofrequency ablation of benign thyroid nodules and recurrent thyroid cancers: consensus statement and recommendations. Korean J Radiol 13:117–25.
- Yi B, Somasundar P, Espat NJ. (2009). Novel laparoscopic bipolar radiofrequency energy technology for expedited hepatic tumour ablation. HPB (Oxford) 11:135–9.
- Holmer C, Lehmann KS, Knappe V, et al. (2011). Bipolar radiofrequency ablation for nodular thyroid disease – ex vivo and in vivo evaluation of a dose–response relationship. J Surg Res 169:234–40.
- Lee JM, Han JK, Kim SH, et al. (2004). Hepatic bipolar radiofrequency ablation using perfused-cooled electrodes: a comparative study in the ex vivo bovine liver. Br J Radiol 77:944–9.
- Feng B, Liang P, Cheng Z, et al. (2012). Ultrasound-guided percutaneous microwave ablation of benign thyroid nodules: experimental and clinical studies. Eur J Endocrinol 166:1031–7.