Abstract
Purpose: Intestinal epithelial barrier dysfunction is crucial in the pathogenesis of intestinal and systemic diseases. Ferulic acid (FA) possesses promising antioxidant activities. In a previous study, we demonstrated potentially protective effects of FA against heat stress-induced intestinal epithelial barrier dysfunction in IEC-6 cells. However, the underlying mechanisms are unclear. The present study aimed to elucidate whether FA protects IEC-6 cells from heat stress-induced intestinal epithelial barrier dysfunction via antioxidative mechanisms.
Materials and methods: IEC-6 cells were pretreated with FA prior to hyperthermia exposure at 42 °C for 6 h, and the levels of intracellular reactive oxygen species (ROS), malondialdehyde (MDA), nitrogen oxide (NO), and superoxide dismutase (SOD) activity were analyzed. The intestinal epithelial barrier function was determined by transepithelial electrical resistance (TER) values and 4-kDa fluorescein isothiocyanate-dextran (FD4) flux in IEC-6 cell monolayers. Expression of related proteins was detected by Western blotting.
Results: FA suppressed heat stress-induced intestinal oxidative stress damage by reducing ROS, MDA and NO production, while enhancing SOD activity. Furthermore, FA treatment strengthened intestinal barrier function via increasing the phosphorylation levels of Akt, nuclear factor-erythroid 2-related factor 2 (Nrf2) and hemeoxygenase-1 (HO-1) protein expression, which was reversed by zinc protoporphyrin (an HO-1 inhibitor). Additionally, LY294002, a specific PI3K/Akt inhibitor, significantly suppressed FA-induced Nrf2 nuclear translocation and HO-1 protein expression and inhibited FA-induced occludin and ZO-1 protein expression.
Conclusions: FA protected against heat stress-induced intestinal epithelial barrier dysfunction via activating the PI3K/Akt-mediated Nrf2/HO-1 signaling pathway in IEC-6 cells.
Introduction
The intestinal epithelium is lined by a single layer of enterocytes that form a dynamic, permeable barrier enabling the selective absorption of water and nutrients, while restricting access to luminal pathogens and foodborne antigens [Citation1]. When the permeability of intestinal epithelial cells increases, antigens and endotoxins in the lumen enter the circulatory system, contributing to the development of local and systemic diseases, such as inflammatory bowel disease (IBD), sepsis and heatstroke [Citation2–4]. Tight junctions (TJs), the interfaces between the apical and basolateral plasma membrane domains, are the primary determinants of paracellular permeability [Citation5]. TJs are composed of various proteins, among which the transmembrane protein occludin and cytoplasmic protein zonula occludens-1 (ZO-1) maintain TJs structure and intestinal epithelial barrier function [Citation6].
Heat stress refers to conditions that increase body temperature, which elevate human morbidity and mortality compared with normothermic conditions [Citation7]. Heat stress increases the intestinal permeability of pathogens and endotoxins, primarily due to the destruction of protective mucosal lining and widening of epithelial tight junction spaces [Citation8,Citation9]. Thus far, the most prevailing hypothesis to explain heat stress-induced disruption of the intestinal epithelial barrier is that hyperthermia induces splanchnic hypoperfusion to adapt to heat radiation in the periphery, causing intestinal epithelial hypoxia and subsequently oxidative stress [Citation9–11].
Oxidative stress is associated with heat stress-induced intestinal epithelial barrier dysfunction [Citation9,Citation12]. Previous studies have shown that heat stress increases intestinal permeability and intestinal epithelial injury, which can be countered by antioxidant agents [Citation13,Citation14]. Hemeoxygenase-1 (HO-1), also referred to as heat shock protein-32 (Hsp32), is a regulator of oxidative stress and provides an important cellular response that protects cells against oxidative damage [Citation15–17]. Evidence suggests that the upregulation of HO-1 contributes to the protection against an increase in intestinal permeability and TJ deterioration [Citation18–20]. The nuclear factor-erythroid 2-related factor 2 (Nrf2) is a key regulator of HO-1, which binds to antioxidant responsive element (ARE), which acts as a cis-acting enhancer sequence that mediates the transcriptional activation of Nrf2 in the case of oxidative stress [Citation21]. A recent study demonstrated that Nrf2 protects cells against burn-induced intestinal structure damage and intestinal permeability increase [Citation22]. Furthermore, previous studies have revealed that phosphatidylinositol 3-kinase (PI3K)/Akt is an upstream signaling molecule of Nrf2 that plays a dominant role in the cellular defense system against oxidative stress [Citation23,Citation24]. Therefore, the PI3K/Akt pathway is a potential target of drug antioxidative therapy.
Ferulic acid (4-hydroxy-3-methoxycinnamic acid, FA), which belongs to the broad category of phenols, is a natural phytochemical widely found in vegetables and fruits and is also a major active ingredient in many Chinese medicinal herbs, such as Angelica sinensis, Ligusticum chuanxiong and Cimicifuga foetida [Citation25]. The antioxidant effect of FA has been verified against several acute and chronic pathologies, such as diabetes, intestinal ischemia, Alzheimer's disease, cancer and cardiovascular diseases [Citation26,Citation27]. Previous studies found that FA regulated the antioxidant properties against intestinal ischemia-reperfusion injury in vivo [Citation28]. Furthermore, the studies in vitro showed that FA increased ZO-1 and claudin-4 transcription in T84 colon cells [Citation29].
Our previous studies indicated that FA can effectively inhibit heat stress-induced increased paracellular permeability, and protect the epithelial barrier during heat stress by maintaining expression and stabilizing localization of TJs proteins in IEC-6 cells [Citation30]. Moreover, using a heat stress rat model, FA administration significantly reduced the increase of intestinal permeability, improved the disruption of TJs structure and attenuated reduction of TJs protein expression in the intestinal of heat stress-treated rats [Citation30]. However, the molecular mechanisms underlying the protective effect of FA on intestinal epithelial barrier have not been clarified. Therefore, the present study aimed to investigate the antioxidant effects and underlying mechanisms of FA in the protection against heat stress-induced intestinal epithelial barrier dysfunction in IEC-6 cells.
Materials and methods
Reagents
FA (purity >99%), a high purity product derived from plant sources, was obtained from the National Institutes for Food and Drug Control (Beijing, China). Dulbecco’s modified Eagle’s medium (DMEM), fetal bovine serum (FBS) and antibiotic-antimycotic were purchased from Gibco (NY, USA). Fluorescein isothiocyanate-dextran (FITC-dextran, MW 4000 Da, FD4), protease inhibitors and zinc protoporphyrin (ZnPP) were purchased from Sigma-Aldrich (MO, USA). Transwell permeable supports were obtained from Corning (6.5 mm; Costar, MA, USA). LY294002 was purchased from Cell Signaling Technology (Danvers, MA, USA).
Cell culture and treatment
Rat intestinal epithelial (IEC-6) cells were obtained from the Cell Resource Center, Peking Union Medical College (Beijing, China) and maintained in DMEM (Gibco, NY, USA) with 10% fetal bovine serum (FBS, Gibco, NY, USA), 100 U/mL penicillin, 100 mg/mL streptomycin and 0.01 mg/mL insulin in a humidified (37 °C and 5% CO2) incubator. IEC-6 cells were pretreated with FA (5, 10 and 20 μM) for 4 h, followed by heat stress (42 °C) treatment for 6 h. In separate experiments, the cells were pretreated with ZnPP (10 μM) or LY294002 (10 μM) for 1 h prior to FA treatment.
Intracellular ROS determination
Intracellular reactive oxygen species (ROS) levels were detected by a fluorescence probe, 2',7'-dichlorodihydrofluorescein diacetate (DCFH2-DA; Invitrogen, CA, USA). Briefly, IEC-6 cells, cultured in 48-well plates for 24 h before the experiment, were pretreated with FA (5, 10 or 20 μM) for 4 h and then exposed to heat stress (42 °C) for 6 h. The cells were washed three times with PBS and then incubated with 20 μM DCFH2-DA for 15 min. Intracellular fluorescence was visualized by using fluorescence microscopy (Olympus, IX71, Tokyo, Japan), and fluorescence intensity was calculated by a fluorescence microplate reader (Tecan, Männedorf, Switzerland) at an excitation wavelength of 495 nm and an emission wavelength of 525 nm.
NO, SOD and MDA determination
After IEC-6 cells were seeded onto 96-well plates and cultured overnight, the supernatants were collected for nitric oxide (NO) release measurement by an NO assay kit (Beyotime Company, Beijing, China) according to the manufacturer’s protocol. Superoxide dismutase (SOD) activity and malondialdehyde (MDA) content were detected by using commercial kits (Nanjing Jiancheng Bioengineering Institute, Nanjing, China) according to the manufacturer’s instructions.
Intestinal epithelial barrier function determination
The intestinal epithelial barrier function was determined by transepithelial electrical resistance (TER) values and FD4 flux as previously described [Citation30]. IEC-6 cells were placed on polycarbonate membranes in Transwell inserts and monitored daily by measuring TER for 2 weeks. After the cells were fully confluent, different FA concentrations (5, 10 or 20 μM) were added to the apical of the filter for 4 h, followed by heat stress treatment. The TER values were detected by a Millicell-ERS voltohmmeter (Millipore, MA, USA). Changes in TER under various experimental conditions were calculated as a percentage of the initial values. To investigate intestinal epithelial paracellular permeability, FD4 (1 mg/mL) was applied to the apical chamber, and the medium was collected from the basolateral chamber after incubation for 2 h. The fluorescence intensity of FITC was quantified by using a fluorescence plate reader (BioTek, Winooski, VT, USA) with excitation at 492 nm and emission at 520 nm.
Western blot analysis
IEC-6 cells were lysed in RIPA buffer (Beyotime Company, Beijing, China) with protease inhibitors on ice to extract total protein. Nuclear protein was extracted by using a nuclear protein extraction kit (KeyGen Biotech, Ningjing, China). The protein concentrations were measured by using a BCA protein assay kit (Pierce, Illinois, IL, USA). Equal amounts of protein (20 μg) were separated by SDS-PAGE and then transferred onto nitrocellulose membrane (Pierce). After the membranes were blocked with Odyssey blocking buffer (LI-COR Biosciences, Nebraska, NE, USA) for 2 h, they were incubated with the following primary antibodies: rabbit anti-occludin (#71–1500, Invitrogen), rabbit anti-ZO-1 (#61–7300, Invitrogen), rabbit anti-Akt (#4685, Cell Signaling Technology), rabbit anti-phospho-Akt (#4060, Cell Signaling Technology), rabbit anti-PCNA (#13110, Cell Signaling Technology), rabbit anti-β-actin (#4970, Cell Signaling Technology), rabbit anti-Nrf2 (#ab31163, Abcam) and mouse anti-HO-1 (#ab13248, Abcam). The membranes were then washed and incubated with IRDye 800CW goat anti-rabbit IgG (#926–32211, LI-COR Biosciences) or IRDye 800CW goat anti-mouse IgM (#926–32280, LI-COR Biosciences) secondary antibody at a dilution of 1:15 000 for 30 min at room temperature. Then, the membranes were visualized by using the Odyssey infrared imaging system (LI-COR Biosciences) and analyzed with ImageJ (National Institutes of Health, Bethesda, MD, USA).
Statistical analysis
The data are represented as the means ± SEM. Statistical analyses were performed by using GraphPad Prism 5 software (GraphPad, CA, USA). One-way or two-way repeated measures (RM) ANOVA were performed where appropriate, and Tukey or Bonferroni’s post-tests were used to evaluate significant differences. In all cases, the results were considered significant at p < .05.
Results
FA inhibited heat stress-induced oxidative stress in IEC-6 cells
ROS production, SOD activity, MDA content and NO release from IEC-6 cells were determined to evaluate the potential antioxidant effect of FA. The results showed that heat-stress challenge significantly increased the level of ROS in IEC-6 cells, but pretreatment with FA notably prevented heat stress-induced ROS generation. As a well-established ROS scavenger, NAC treatment also showed a significant inhibitory effect on heat stress-induced ROS overproduction (). Furthermore, the levels of MDA and NO were markedly increased, while SOD activity was significantly decreased under heat stress. When cells were pretreated with FA, the levels of SOD activity significantly increased, while the levels of MDA and NO markedly decreased in a dose-dependent manner ().
Figure 1. Effects of FA on heat stress-induced intracellular ROS, SOD, MDA and NO changes in IEC-6 cells. (A and B) IEC-6 cells were pretreated with FA (0, 5, 10 and 20 μM) for 4 h in the presence or absence of 50 μM NAC for 1 h prior to exposure to heat stress (42 °C, 6 h). The cells were incubated with DCFH2-DA for 15 min. ROS generation was detected by a fluorescence microscope, and the fluorescence values of ROS were measured by using a fluorescence microplate reader (Scale bar =200 μm). (C, D and E) The cells were pretreated with FA (0, 5, 10 and 20 μM) for 4 h and then exposed to heat stress. The contents of SOD and MDA were determined by using commercial kits. The NO concentration in the supernatant was determined by the Griess reaction. Data are presented as the means ± SEM of three independent experiments. Statistical analysis was performed by using one-way ANOVA and Tukey’s test. *p < .05, **p < .01 compared to the control group; #p < .05, ##p < .01 compared to the heat stress-treated group.
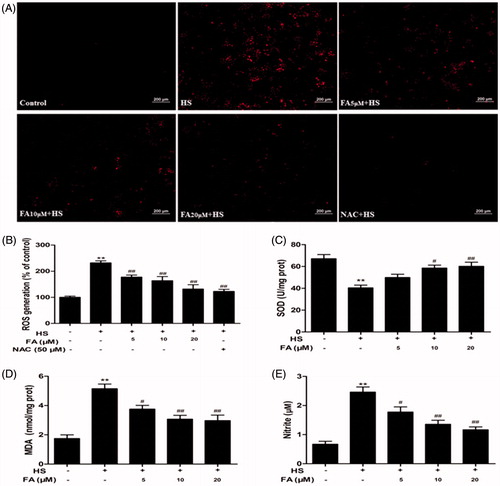
FA protected against heat stress-induced intestinal TJ permeability increase via regulating HO-1 protein expression in IEC-6 cells
Western blot analysis was performed to detect HO-1 protein levels in IEC-6 cells treated with different concentrations of FA (5, 10 and 20 μM) at different time points (0, 1, 4, 8 and 12 h). The data demonstrated that FA increased the levels of HO-1 protein in a time- and dose- dependent manner (). The relative quantity of HO-1 was significantly increased at 4 h after 20 μM FA treatment.
Figure 2. Effects of FA on HO-1 protein expression and intestinal TJ permeability changes in IEC-6 cells. (A) The cells were treated with FA (20 μM) for the indicated time periods (0, 1, 4, 8 and 12 h). (B) The cells were treated with the indicated concentrations (0, 5, 10 and 20 μM) of FA for 4 h. The cell lysates were subjected to Western blot analysis with an anti-HO-1 antibody. β-Actin was used as a loading control. (C) The cells were pretreated with ZnPP (10 μM) for 1 h prior to incubation with FA (20 μM) for 4 h, and then the proteins were extracted for Western blot analysis by using an anti-HO-1 antibody. (D) The cells were pretreated with ZnPP (10 μM) for 1 h and then incubated with or without FA (20 μM) for 4 h, followed by exposure to heat stress. The TER values were monitored across the IEC-6 cell monolayers by using Millicell-ERS. Data are presented as the means ± SEM from three independent experiments. Data are presented as the means ± SEM of three independent experiments. Statistical analysis was performed by using one-way ANOVA and Tukey’s test. *p < .05, **p < .01 compared to the control group; ##p < .01 compared to the heat stress-treated group; &&p < .01 compared to the FA-treated group.
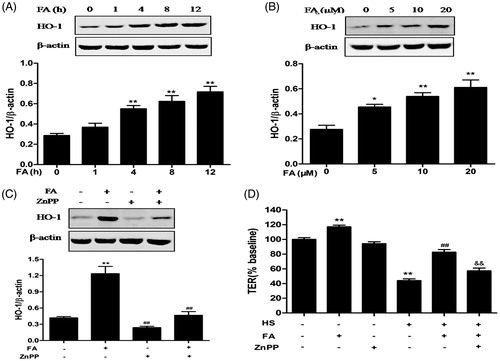
FA-induced HO-1 protein expression was significantly reduced when cells were pretreated with the HO-1 inhibitor, zinc protoporphyrin (ZnPP) (). In addition, FA significantly increased TER in IEC-6 cell monolayers, while ZnPP significantly inhibited the FA-induced TER increase after exposure to heat stress (). These data indicated that HO-1 contributed to the protective effect of FA against the heat stress-induced increase in intestinal TJ permeability.
FA increased the nuclear translocation of Nrf2 and activated the PI3K/Akt pathway in IEC-6 cells
Nrf2, a major transcription factor mediator of HO-1, was examined by Western blot analysis to further characterize the molecular mechanism of FA activity. The results showed that nuclear Nrf2 protein expression was significantly increased at 4 and 8 h and remained elevated for up to 12 h, whereas cytoplasmic Nrf2 protein expression was decreased at 4 and 8 h and remained low for up to 12 h compared with that the expression in the control group (). FA induced the translocation of Nrf2 from the cytosol to the nucleus in a dose-dependent manner ().
Figure 3. Effects of FA on Nrf2 nuclear translocation and PI3K/Akt phosphorylation in IEC-6 cells. (A and C) The cells were treated with FA (20 μM) for the indicated time periods (0, 1, 4, 8 and 12 h). (B and D) The cells were treated with the indicated concentrations (0, 5, 10 and 20 μM) of FA for 4 h. (A and B) The nuclear and cytosolic levels of Nrf2 were determined by Western blot analysis. PCNA was used as a nuclear loading control. Data are presented as the means ± SEM of three independent experiments. **p < .01 calculated versus control by using two-way ANOVA and Bonferroni’s post-test. (C and D) The cell lysates were subjected to Western blot analysis with anti-Akt or p-Akt antibodies. Data are presented as the means ± SEM of three independent experiments. *p < .05, **p < .01 calculated versus control by using one-way ANOVA and Tukey’s test.
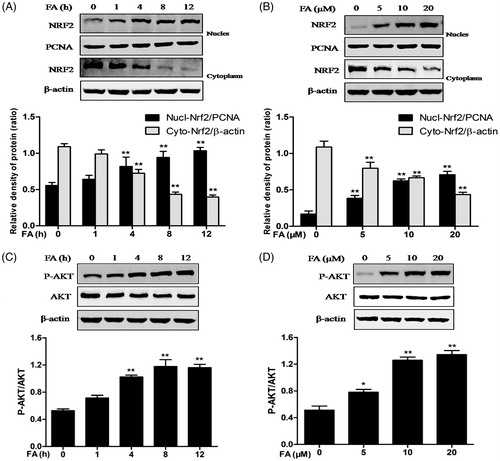
PI3K/Akt is upstream of Nrf2 and induces Nrf2/ARE-driven gene expression. After treatment with FA, the phosphorylation of Akt in IEC-6 cells was analyzed by Western blotting. The data showed that Akt phosphorylation was dose- and time-dependently enhanced after treatment with FA, although the total Akt protein levels showed no significant changes ().
FA increased occludin and ZO-1 protein expression and intestinal epithelial barrier function in IEC-6 cells
Since epithelial TJs increase intestinal epithelial barrier function, the expression of two representative TJ proteins, ZO-1 and occludin, was detected by Western blot analysis. Occludin protein expression was significantly increased at 1, 4, 8 and 12 h after treatment with FA. ZO-1 was also significantly upregulated at 4, 8 and 12 h after treatment with FA (). Moreover, FA increased occludin and ZO-1 protein expression in a dose-dependent manner (). The results indicated that occludin and ZO-1 protein levels were dose- and time-dependently elevated after treatment with FA.
Figure 4. Effects of FA on occludin and ZO-1 protein expression and intestinal TJ permeability in IEC-6 cells. (A and C) The cells were treated with FA (20 μM) for the indicated time periods (0, 1, 4, 8 and 12 h). (B and D) The cells were treated with the indicated concentrations (0, 5, 10 and 20 μM) of FA for 4 h. (A and B) The cell lysates were subjected to Western blot analysis with an anti-occludin antibody. β-Actin was used as a loading control. (C and D) The cell lysates were subjected to Western blot analysis with an anti-ZO-1 antibody. β-actin was used as a loading control. Data are presented as the means ± SEM of three independent experiments. *p < .05, **p < .01 calculated versus control by using one-way ANOVA and Tukey’s test. (E) The cells on Transwell insert membranes were pretreated with FA (0, 5, 10 or 20 μM) at the indicated time points. The TER values were monitored across the cell monolayers. Data are presented as the means ± SEM of three independent experiments. Statistical analysis was performed by using two-way ANOVA and Bonferroni’s post-test. Means not sharing the same letter are significantly different from each other (p < .05).
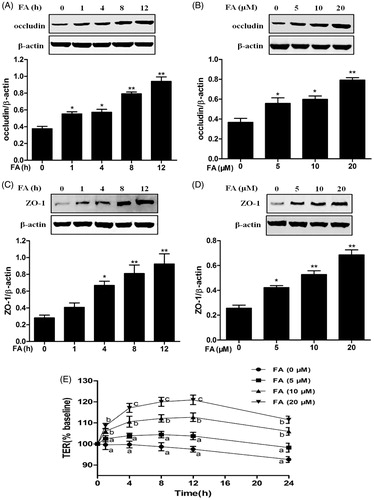
Additionally, the effect of FA on intestinal epithelial TJ permeability was measured by TER. A time- and dose-dependent increase in TER values was observed starting from 1 h after treatment with 10 and 20 μM FA. The TER values peaked at 12 h after treatment with 20 μM FA, with a 20.92% gain compared with the initial value ().
The PI3K/Akt pathway was involved in FA-induced Nrf2 nuclear translocation and HO-1 upregulation in IEC-6 cells
LY294002, a specific PI3K/Akt inhibitor, was used to inhibit Akt phosphorylation in IEC-6 cells to elucidate whether the activation of PI3K/Akt signaling pathway was essential for the nuclear translocation of Nrf2 and HO-1 upregulation induced by FA. The results revealed that LY294002 significantly suppressed FA-induced Akt phosphorylation and nuclear Nrf2 protein expression, whereas the level of Nrf2 protein in the cytoplasm increased (). Furthermore, LY294002 significantly suppressed the FA-induced increase in HO-1 protein expression (). These findings suggested that the PI3K/Akt signaling pathway was essential for FA antioxidant activity by increasing the FA-induced upregulation of HO-1 and nuclear translocation of Nrf2.
Figure 5. Effects of the PI3K/Akt pathway on FA-induced Nrf2 nuclear translocation and HO-1 expression. (A, B and C) IEC-6 cells were pretreated with 10 μM LY294002 for 1 h and then treated with 20 μM FA for 4 h. The levels of Nrf2, P-Akt and HO-1 protein were determined by Western blot analysis. PCNA was used as a nuclear loading control. Data are presented as the means ± SEM of three independent experiments. Statistical analysis was performed by using one-way ANOVA and Tukey’s test. **p < .01 compared to the control group; ##p < .01 compared to the heat stress-treated group.
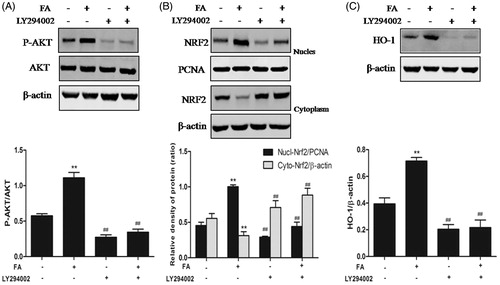
The PI3K/akt pathway was involved in the FA-induced protein upregulation of occludin and ZO-1 in IEC-6 cells
By using the specific inhibitor LY294002, the present study investigated whether the PI3K/Akt signaling pathway was involved in the FA-induced increase in TJ protein expression. The results showed that FA significantly decreased occludin and ZO-1 protein expression in IEC-6 cells after pretreatment with LY294002 (10 μM) ().
Figure 6. Effects of the PI3K/Akt pathway on FA-induced occludin and ZO-1 protein expression in IEC-6 cells. (A and B) IEC-6 cells were pretreated with 10 μM LY294002 for 1 h and then treated with 20 μM FA for 4 h. The levels of occludin and ZO-1 proteins were determined by Western blot analysis. Data are presented as the means ± SEM of three independent experiments. Statistical analysis was performed by using one-way ANOVA and Tukey’s test. **p < .01 compared to the control group; ##p < .01 compared to the heat stress-treated group.
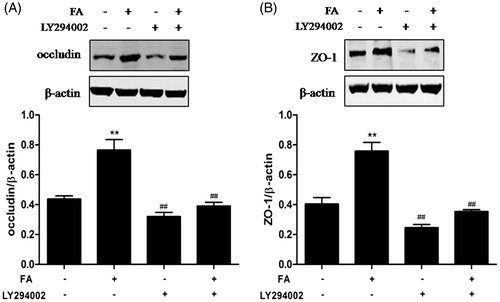
The PI3K/Akt signaling pathway was involved in the FA-mediated prevention of the heat stress-induced increase in intestinal TJ permeability
The present study investigated the effect of LY294002 on the FA-mediated prevention of heat stress-induced intestinal TJ permeability by measuring TER and FD4 flux in IEC-6 cell monolayers. The TER values were increased, while the FD4 flux was significantly decreased after pretreatment with 20 μM FA. However, the FA-mediated prevention of heat stress-induced changes in TER and FD4 flux were largely inhibited by LY294002, indicating that the PI3K/Akt signaling pathway plays a dominant role in the FA-mediated protection of TJ (.
Figure 7. Effects of the PI3K/Akt pathway on the FA-mediated prevention of the heat stress-induced increase in intestinal TJ permeability in IEC-6 cell monolayers. (A and B) The cells on Transwell insert membranes were pretreated with or without 20 μM FA for 4 h in the presence or absence of 10 μM LY294002 for 1 h, followed by exposure to heat stress. (A) The TER values were monitored across the cell monolayers by using Millicell-ERS. (B) The permeability of FD4 across the cell monolayer was measured at 2 h after exposure to heat stress. Data are presented as the means ± SEM of three independent experiments. Statistical analysis was performed by using one-way ANOVA and Tukey’s test. **p < .01 compared to the control group; ##p < .01 compared to the heat stress-treated group; &&p < .01 compared to the FA-treated group.
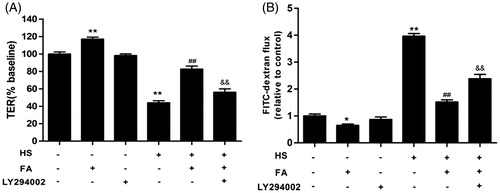
Discussion
FA is a ubiquitous phenolic compound in plant tissues reported to exhibit a large range of biological activities, such as antioxidant, anti-inflammatory and antihypertensive activities [Citation31]. Previous studies have shown that FA pretreatment potentially protects against heat stress-induced intestinal epithelial barrier dysfunction by increasing TJ protein expression and maintaining TJ structure in vitro and in vivo [Citation30]. In the present study, we provided the first evidence that FA could have beneficial effects on intestinal epithelial barrier function via the PI3K/Akt-mediated Nrf2/HO-1 antioxidant signaling pathway in IEC-6 cells.
Oxidative stress, particularly the production of excessive ROS, induces cellular damage by targeting various macromolecules, including proteins, lipids and DNA [Citation32]. The overproduction of ROS causes oxidative stress and contributes to intestinal epithelial barrier dysfunction [Citation33]. MDA, which is produced by the lipid peroxidation of polyunsaturated fatty acids, is a commonly used biomarker for measuring oxidative stress levels in an organism [Citation34]. SOD, an important antioxidant enzyme, participates in antioxidant defenses by scavenging free radicals [Citation35]. Studies have reported that excessive NO may react with superoxide anion radicals, giving rise to strong oxidizing agents, such as peroxynitrite, which consequently destroy functional tissues [Citation36]. In the present study, heat stress remarkably increased the levels of MDA and NO and decreased SOD activity. Pretreatment with FA significantly decreased the levels of ROS, NO and MDA, but increased SOD activity in IEC-6 cells. These findings indicated that FA could increase the antioxidant capacity and prevent the damage of oxidative stress induced by heat stress in IEC-6 cells.
Many studies have indicated that upregulated HO-1 mediates protection against intestinal epithelial barrier deterioration [Citation18–20]. Recently, several studies have reported that FA attenuates the oxidative stress-induced injury of preadipocytes, cardiomyocytes, hepatocytes and neurons via the induction of HO-1 expression [Citation37–39]. Hence, we speculate that HO-1 protein plays a major role in the FA-induced protective effect against the heat stress-induced increase in intestinal TJ permeability. ZnPP, an HO-1 inhibitor, was widely used as an antioxidant enzyme heme oxygenase-1 [Citation40,Citation41]. The present study revealed that FA treatment significantly upregulated the expression of HO-1 protein in IEC-6 cells, which can be blocked in the presence of ZnPP. Since HO-1 contributes to intestinal epithelial barrier function, we studied the effects of HO-1 on the heat stress-induced increase in intestinal epithelial barrier permeability. The results showed that ZnPP partially reversed the FA-induced TER increase after exposure to heat stress. These data indicated that the increase in HO-1 protein expression in IEC-6 cells contributed to the FA-induced protective effect against the heat stress-induced increase in intestinal TJ permeability, indicating that the HO-1 protein plays a dominant role in antioxidant activity induced by FA.
Nrf2 protects cells against environmental and oxidative stress and maintains the cellular redox balance by promoting the gene expression of the antioxidant enzyme HO-1 [Citation21]. Under physiological conditions, Nrf2 is present in the cytoplasm with Keap1 as an inactive complex. When stimulated, Nrf2 dissociates from Keap1, translocates to the cell nucleus and binds to ARE sequences, leading to the transcriptional activation of a series of cytoprotective and antioxidants genes [Citation42]. The present results suggested that FA induced the translocation of Nrf2 from the cytosol to the nucleus in IEC-6 cells and might upregulate HO-1 protein expression through the Nrf2 signaling pathway.
The PI3K/Akt signaling pathway is involved in Nrf2-dependent transcription and overexpression of HO-1 protein in various cell types under oxidative stress [Citation43–45]. The results of the present study suggested that FA remarkably increased the phosphorylation level of Akt protein, which might contribute to FA-induced Nrf2/HO-1 protein upregulation. LY294002, a specific PI3K/Akt inhibitor, significantly suppressed nuclear Nrf2 protein expression and largely blocked the upregulation of HO-1 protein induced by FA. This finding indicated that the PI3K/Akt signaling pathway is associated with FA-induced Nrf2/HO-1 activation.
TJs are the primary determinants of paracellular permeability. Occludin and ZO-1 proteins have been implicated in maintaining TJ structure and intestinal epithelial barrier function [Citation6]. A significant body of evidence indicates that TJs are associated with various intracellular signaling molecules and are controlled by a number of signaling pathways [Citation9,Citation46,Citation47]. Hence, the present study showed that FA significantly increased the proteins levels of occludin and ZO-1 in a time- and dose-dependent manner. Additionally, FA progressively increased TER in IEC-6 cell monolayers. These findings demonstrated that FA could enhance intestinal epithelial barrier function. However, LY294002 significantly blocked the FA-induced increase in occludin and ZO-1 protein expression, implicating the PI3K/Akt signaling pathway in FA-induced intestinal barrier function dysfunction. Furthermore, the FA-mediated prevention of heat stress-induced changes in TER was significantly reduced by LY294002, suggesting that the PI3K/Akt signaling pathway was essential for the FA-mediated protection of intestinal barrier function.
In conclusion, the present study demonstrated that FA protected IEC-6 cells against heat stress-induced oxidative damage and increased intestinal epithelial barrier permeability. Additionally, FA alleviated heat stress-induced intestinal epithelial barrier dysfunction by activating the PI3K/Akt-mediated Nrf2/HO-1 antioxidant signaling pathway. These results provide new insights into the molecular mechanisms underlying the FA-mediated protective effects of intestinal epithelial barrier function and have important clinical applications in intestinal barrier dysfunction-related diseases.
Disclosure statement
The authors confirm that there are no conflicts of interest.
Additional information
Funding
References
- Luissint AC, Parkos CA, Nusrat A. Inflammation and the intestinal barrier: leukocyte-epithelial cell interactions, cell junction remodeling, and mucosal repair. Gastroenterology. 2016;151:616–632.
- Lee SH. Intestinal permeability regulation by tight junction: implication on inflammatory bowel diseases. Intest Res. 2015;13:11–18.
- Xiao G, Yuan F, Geng Y, et al. Eicosapentaenoic acid enhances heatstroke-impaired intestinal epithelial barrier function in rats. Shock. 2015;44:348–356.
- Yoseph BP, Klingensmith NJ, Liang Z, et al. Mechanisms of intestinal barrier dysfunction in sepsis. Shock. 2016;46:52–59.
- Odenwald MA, Turner JR. The intestinal epithelial barrier: a therapeutic target?. Nat Rev Gastroenterol Hepatol. 2017; 14:9–21.
- Costantini TW, Deree J, Loomis W, et al. Phosphodiesterase inhibition attenuates alterations to the tight junction proteins occludin and ZO-1 in immunostimulated Caco-2 intestinal monolayers. Life Sci. 2009; 84:18–22.
- Crandall CG, Wilson TE. Human cardiovascular responses to passive heat stress. Compr Physiol. 2015;5:17–43.
- Xiao G, Tang L, Yuan F, et al. Eicosapentaenoic acid enhances heat stress-impaired intestinal epithelial barrier function in Caco-2 cells. Plos One. 2013;8:e73571.
- Dokladny K, Zuhl MN, Moseley PL. Intestinal epithelial barrier function and tight junction proteins with heat and exercise. J Appl Physiol (1985). 2016;120:692–701.
- Bhattacharyya A, Chattopadhyay R, Mitra S, et al. Oxidative stress: an essential factor in the pathogenesis of gastrointestinal mucosal diseases. Physiol Rev. 2014;94:329–354.
- He S, Hou X, Xu X, et al. Quantitative proteomic analysis reveals heat stress-induced injury in rat small intestine via activation of the MAPK and NF-kappaB signaling pathways. Mol Biosyst. 2015;11:826–834.
- Oliver SR, Phillips NA, Novosad VL, et al. Hyperthermia induces injury to the intestinal mucosa in the mouse: evidence for an oxidative stress mechanism. Am J Physiol Regul Integr Comp Physiol. 2012;302:R845–R853.
- Varasteh S, Fink-Gremmels J, Garssen J, et al. alpha-Lipoic acid prevents the intestinal epithelial monolayer damage under heat stress conditions: model experiments in Caco-2 cells. Eur J Nutr. 2018;57:1577–1589.
- Lambert GP, Gisolfi CV, Berg DJ, et al. Selected contribution: hyperthermia-induced intestinal permeability and the role of oxidative and nitrosative stress. J Appl Physiol (1985). 2002;92:1750–1761. 1749.
- Ryter SW, Choi AM. Targeting heme oxygenase-1 and carbon monoxide for therapeutic modulation of inflammation. Transl Res. 2016;167:7–34.
- Xu X, Guo Y, Zhao J, et al. Punicalagin, a PTP1B inhibitor, induces M2c phenotype polarization via up-regulation of HO-1 in murine macrophages. Free Radic Biol Med. 2017;110:408–420.
- Zhu X, Fan WG, Li DP, et al. Heme oxygenase-1 system and gastrointestinal inflammation: a short review. World J Gastroenterol. 2011;17:4283–4288.
- Wang N, Wang G, Hao J, et al. Curcumin ameliorates hydrogen peroxide-induced epithelial barrier disruption by upregulating heme oxygenase-1 expression in human intestinal epithelial cells. Dig Dis Sci. 2012;57:1792–1801.
- Seo GS, Jiang WY, Park PH, et al. Hirsutenone reduces deterioration of tight junction proteins through EGFR/Akt and ERK1/2 pathway both converging to HO-1 induction. Biochem Pharmacol. 2014;90:115–125.
- Chi X, Yao W, Xia H, et al. Elevation of HO-1 Expression mitigates intestinal ischemia-reperfusion injury and restores tight junction function in a rat liver transplantation model. Oxid Med Cell Longev. 2015;2015:1.
- Park SA, Lee MH, Na HK, et al. 4-Hydroxyestradiol induces mammary epithelial cell transformation through Nrf2-mediated heme oxygenase-1 overexpression. Oncotarget. 2017;8:164–178.
- Chen Z, Zhang Y, Ma L, et al. Nrf2 plays a pivotal role in protection against burn trauma-induced intestinal injury and death. Oncotarget. 2016;7:19272–19283.
- Wang P, Peng X, Wei ZF, et al. Geraniin exerts cytoprotective effect against cellular oxidative stress by upregulation of Nrf2-mediated antioxidant enzyme expression via PI3K/AKT and ERK1/2 pathway. Biochim Biophys Acta. 2015;1850:1751–1761.
- Han D, Chen W, Gu X, et al. Cytoprotective effect of chlorogenic acid against hydrogen peroxide-induced oxidative stress in MC3T3-E1 cells through PI3K/Akt-mediated Nrf2/HO-1 signaling pathway. Oncotarget. 2017;8:14680–14692.
- Mancuso C, Santangelo R. Ferulic acid: pharmacological and toxicological aspects. Food Chem Toxicol. 2014;65:185–195.
- Srinivasan M, Sudheer AR, Menon VP. Ferulic Acid: therapeutic potential through its antioxidant property. J Clin Biochem Nutr. 2007;40:92–100.
- Ghosh S, Basak P, Dutta S, et al. New insights into the ameliorative effects of ferulic acid in pathophysiological conditions. Food Chem Toxicol. 2017;103:41–55.
- Itagaki S, Kurokawa T, Nakata C, et al. In vitro and in vivo antioxidant properties of ferulic acid: a comparative study with other natural oxidation inhibitors. Food Chem. 2009;114:466–471.
- Bergmann H, Rogoll D, Scheppach W, et al. The Ussing type chamber model to study the intestinal transport and modulation of specific tight-junction genes using a colonic cell line. Mol Nutr Food Res. 2009;53:1211–1225.
- He S, Liu F, Xu L, et al. Protective effects of ferulic acid against heat stress-induced intestinal epithelial barrier dysfunction in vitro and in vivo. Plos One. 2016;11:e0145236. e145236.
- Kumar N, Pruthi V. Potential applications of ferulic acid from natural sources. Biotechnol Rep (Amst). 2014;4:86–93.
- Reth M. Hydrogen peroxide as second messenger in lymphocyte activation. Nat Immunol. 2002;3:1129–1134.
- Elamin E, Masclee A, Juuti-Uusitalo K, et al. Fatty acid ethyl esters induce intestinal epithelial barrier dysfunction via a reactive oxygen species-dependent mechanism in a three-dimensional cell culture model. PLoS One. 2013; 8:e58561.
- Janero DR. Malondialdehyde and thiobarbituric acid-reactivity as diagnostic indices of lipid peroxidation and peroxidative tissue injury. Free Radic Biol Med. 1990;9:515–540.
- Li YM, Wang HB, Zheng JG, et al. Dimethyl sulfoxide inhibits zymosan-induced intestinal inflammation and barrier dysfunction. WJG. 2015;21:10853–10865.
- Mikulec CD, Rundhaug JE, Simper MS, et al. The chemopreventive efficacies of nonsteroidal anti-inflammatory drugs: the relationship of short-term biomarkers to long-term skin tumor outcome. Cancer Prev Res (Phila). 2013;6:675–685.
- Koh EJ, Kim KJ, Seo YJ, et al. L1 Cells. Molecules. 2017;22.
- Yu CL, Zhao XM, Niu YC. Ferulic acid protects against lead acetate-induced inhibition of neurite outgrowth by upregulating HO-1 in PC12 cells: involvement of ERK1/2-Nrf2 pathway. Mol Neurobiol. 2016; 53:6489–6500.
- Song Y, Wen L, Sun J, et al. Cytoprotective mechanism of ferulic acid against high glucose-induced oxidative stress in cardiomyocytes and hepatocytes. Food Nutr Res. 2016;60:30323.
- Wang N, Han Q, Wang G, et al. Resveratrol protects oxidative stress-induced intestinal epithelial barrier dysfunction by upregulating heme oxygenase-1 expression. Dig Dis Sci. 2016;61:2522–2534.
- Cheng CC, Guan SS, Yang HJ, et al. Blocking heme oxygenase-1 by zinc protoporphyrin reduces tumor hypoxia-mediated VEGF release and inhibits tumor angiogenesis as a potential therapeutic agent against colorectal cancer. J Biomed Sci. 2016;23:18.
- Ma ZC, Hong Q, Wang YG, et al. Ferulic acid induces heme oxygenase-1 via activation of ERK and Nrf2. Drug Discov Ther. 2011;5:299–305.
- Hwang YP, Jeong HG. Ginsenoside Rb1 protects against 6-hydroxydopamine-induced oxidative stress by increasing heme oxygenase-1 expression through an estrogen receptor-related PI3K/Akt/Nrf2-dependent pathway in human dopaminergic cells. Toxicol Appl Pharmacol. 2010;242:18–28.
- Xu L, He S, Yin P, et al. Punicalagin induces Nrf2 translocation and HO-1 expression via PI3K/Akt, protecting rat intestinal epithelial cells from oxidative stress. Int J Hyperthermia. 2016;32:465–473.
- Xu X, Li H, Hou X, et al. Punicalagin induces Nrf2/HO-1 expression via upregulation of PI3K/AKT pathway and inhibits LPS-induced oxidative stress in RAW264.7 macrophages. Mediators Inflamm. 2015;2015:380218.
- Wang K, Jin X, Chen Y, et al. Polyphenol-rich propolis extracts strengthen intestinal barrier function by activating AMPK and ERK signaling. Nutrients. 2016;8:272.
- Chen S, Zhu J, Chen G, et al. 1,25-dihydroxyvitamin D3 preserves intestinal epithelial barrier function from TNF-alpha induced injury via suppression of NF-kB p65 mediated MLCK-P-MLC signaling pathway. Biochem Biophys Res Commun. 2015; 460:873–878.