Abstract
Objective: The aim of this study was to investigate the post-sauna residual consequences on brain neural network arousal, information processing and cognitive performance.
Methods: Sixteen male subjects (24 ± 1 yr.) participated in the study. Whole-body hyperthermia was induced with Finnish sauna bathing. Before and 90 min after the sauna, resting electroencephalography (EEG) for spectral analysis and EEG event-related potentials (ERPs) during oddball tasks by two modalities (auditory and visual) were recorded.
Results: Sauna bathing increased rectal temperature (Tre, 37.11 ± 0.33 °C to 38.84 ± 0.32 °C) and heart rate (HR, 65.63 ± 9.39 bpm to 151.0 ± 21.8 bpm). At 90 min after the sauna, Tre (37.00 ± 0.29 °C) and HR (72.1 ± 2.80 bpm) recovered to baseline levels. An increase was found in alpha power following sauna recovery. In the visual task modality, post-sauna recovery led to enhancement in the N2 amplitude with centroparietal distribution and decreases in P3 amplitude with distribution along the frontoparietal axis for executive motor–cognitive processing. In the auditory task modality, post-sauna recovery led to a decrease in P3 amplitude with a frontoparietal distribution and this change was accompanied by auditory N2 amplitude enhancement along the centroparietal distribution for non-target cognitive processing. No significant differences in task performance were found.
Conclusion: Post-sauna recovery to normothermia led to enhanced resting neural network relaxation followed by increases in cognitive processing economy for the given oddball tasks. The auditory processing was not affected more by post-sauna recovery than was visual processing. Post-sauna recovery modifications in ERP components (stimulus processing) were insufficient to affect cognitive performance in both visual and auditory task modalities.
Introduction
Finnish sauna bathing, an activity that promotes relaxation and well-being, has become increasingly popular worldwide. Regular sauna bathing is associated with reduced risks of fatal cardiovascular diseases and all-cause mortality [Citation1], and acts as a protective lifestyle factor against common memory diseases (e.g., dementia and Alzheimer’s disease) [Citation2]. Sauna bathing has also been used as a thermal therapy to treat pain, aches and other symptoms of rheumatic disease [Citation3,Citation4]. There is also experimental evidence showing that sauna bathing therapy reduces the levels of systemic inflammatory markers [Citation5] and improves pulmonary function [Citation6,Citation7]. It also results in decreases in salivary cortisol (a stress-related marker) [Citation8], anxiety and benefits for mood [Citation9], which is of great importance for human general well-being and mental and physical health [Citation10].
The usual sauna visit consists of short stays (5–20 min) in dry air (relative humidity (rh) of 10–20%) at a relatively high temperature (air temperature, Ta 80–100 °C), which induces short-term mild to severe whole-body hyperthermia [Citation11]. Evidence indicates that under such thermal conditions, the heat-stressed brain operates at lower levels of blood and energy supply, resulting in impairments in brain neural networks [Citation12,Citation13] and metabolism [Citation14] which lead to suppression of cognitive function [Citation15] and motor inhibition [Citation12,Citation16]. Even a mild increase in core temperature (∼1.2 °C) acutely decreases cerebral perfusion [Citation17,Citation18] and produces decreases in cognition [Citation19]. External skin cooling (e.g., with fans or ice packs) when body core temperature remains high restores cerebral perfusion and improves thermal perception [Citation19], but does not affect impaired motor functions [Citation20] or cognitive processing [Citation19]. By contrast, whole-body cooling (e.g., with a tube-lined water-perfused suit) to normothermia is sufficient to restore motor performance [Citation20] and to modulate cognitive processing [Citation19]. However, external cold or cool stimuli to the body surface when the body temperature is high can affect low-temperature-sensitive receptors (e.g., TRPA1 and/or TRPM8 channels) in cutaneous nerve endings by sensitizing the preoptic area and insula to increase arousal and alertness for thermoregulatory and sensation responses, respectively [Citation21–23]. Mainly because of cool/cold-induced central arousal, forced external cooling in hyperthermic subjects can blunt the natural residual consequences of the post-heat-stressed brain on perception, behavior and cognitive processing. However, little is known about the functional residual consequences of recovering body temperatures from moderate whole-body hyperthermia in a thermoneutral environment on the effectiveness of cognitive processing. This knowledge gap is surprising given that in the heat-stressed brain, the water, hormonal and neurotransmitter balances are disturbed and brain morphology is altered [Citation24,Citation25], and cognitive modulation (dysfunction or arousal) may persist into the recovery period after removal from the sauna heat or heat per se.
Event-related brain potentials (ERPs) using oddball paradigms, which reflect sensory and cognitive processing, provide a unique and powerful method to investigate alterations in information processing following mental and/or physiological stress [Citation19,Citation26,Citation27]. The early N1 ERP component reflects primary sensory processing [Citation28], whereas later ERP components, including N2 and P3, are associated with cognitive processes, such as stimulus discrimination, evaluation and categorization [Citation29,Citation30]. As these ERP components are sensitive to the stress-related alterations in brain homeostasis [Citation19,Citation31], there is a question as to whether moderate whole-body hyperthermia-induced alterations in brain homeostasis and cognitive processing will persist into residual recovery to normothermia (i.e., 90 min post-sauna). Therefore, the aim of this study was to induce whole-body moderate hyperthermia (rectal temperature, Tre ∼38.5 °C; Lucas et al. [Citation32]) and simultaneously stress the brain (∼0.3 °C warmer than arterial blood entering the brain; Nybo et al. [Citation14]) in subjects using a traditional sauna and to investigate the post-sauna residual consequences on brain neural network arousal, information processing, and cognitive performance. A higher electroencephalography (EEG) alpha power, appearing mostly in a state of relaxation, is associated with reduced cortical activity [Citation33] and is inversely related to cognitive processing [Citation34]. We expected that post-sauna recovery would produce enhanced relaxation in the brain neural network, which would be characterized by an increase in resting EEG alpha activity, and lead to impairments in cognitive processing (stimulus discrimination, evaluation and categorization) and cognitive performance (reaction time and accuracy). We hypothesized that in view of the greater complexity (anatomical and physiological) of the auditory system compared with the visual system [Citation35], greater post-sauna heat-stress modulation of cognitive processing would be more pronounced in tasks with auditory than with visual stimuli.
Methods
Subjects
Sixteen healthy male subjects (24 ± 1 years old, 184.19 ± 2.07 cm in height, 84.27 ± 3.82 kg mass, 24.73 ± 0.81 kg · m−2 body mass index, and 15.74 ± 1.01% body fat; all mean ± standard deviation, SD) were recruited to the study. They were considered healthy, with no mental diseases (e.g., epilepsy or schizophrenia), were physically active with no excessive sport activities (<3 times per week), had normal or corrected-to-normal visual acuity, and did not consume any psychotropic substances or other drugs. Participants were not acclimated to heat. They were asked to avoid strenuous exercise and any alcohol consumption within 48 h before testing, any mental and physical work during testing day, any food and drink (except water) within 4 h before testing, and to avoid any eating or drinking during both study trials. Participants were asked to sleep 7–8 h on the night before testing. All subjects gave written informed consent. The experimental procedures were approved by the Lithuanian University of Health Sciences Kaunas Region Biomedical Research Ethics Committee.
Experimental protocol
The experiment was designed to investigate post-sauna residual effects on brain neural network arousal levels, information processing, and cognitive performance. The subjects were familiarized with the procedure 1 week before the trial and practiced oddball tasks three times on three different days. In an experimental (EXP) trial, on arrival at the laboratory each subject’s nude body was weighed with a TBF-300 scale (Tanita UK Ltd. Philpots Close, UK). After that, each participant was asked to rest in a semirecumbent posture for 20 min in a thermally neutral ambient temperature (Ta 23 °C) dressed in a T-shirt, swim shorts and socks. Rectal temperatures (Tre; measured with a rectal probe, Ellab, Hvidovre, Denmark; accuracy ±0.01 °C, Cernych et al. [Citation36]) and stabilized heart rates (HR; measured with an S-625X Polar Electro monitor, Kempele, Finland) were assessed and recorded. Within ∼3 min after these resting measurements, the participants were seated at a table in an EEG-dedicated room and EEGs were recorded with their eyes closed (to investigate the brain neural network arousal) and during two oddball tasks (to investigate the information processing and cognitive performance). Upon completion of the EEG recording, the participants were asked to enter a sauna. Sauna bathing (Ta 80–90 °C, rh 20%) consisted of four sets (first one 15 min, next three sets 10 min, with 15 min rest between each set with the participants in a semirecumbent posture at Ta 23 °C; .
Figure 1. Schematic representation of the experimental protocol. Temperature in the sauna was 80–90 °C; the ambient temperature (Ta) during rest was 23 °C.
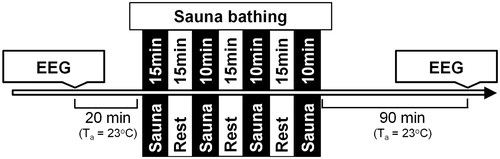
By the end of the fourth sauna set the participants’ Tre and HR were recorded. After sauna completion, each participant was asked to rest for 60 min at Ta 23 °C, then take a 1-min brief warm shower (T 40 °C), dress, and enter the EEG room. The distance from sauna room to EEG-dedicated room was ∼10 meters. Before starting EEG recording, the Tre and HR values were evaluated. EEG was recorded with the participants’ eyes closed and during two oddball tasks. After the EEG recordings, the subjects’ nude bodies were weighed again to determine the amount of sweat lost. In the control (CON) trial, we assessed the same variables in the same time points under normothermia without causing any changes in body temperature. Each participant completed the study trials twice (CON and EXP trials), both times at the same time of day (starting at 4 pm), and at least 7 days apart. The order of trials was randomized.
Oddball tasks
Two oddball tasks in randomized order with 175 stimuli in each task were used in this study. In one task, visual stimuli and in the other task, auditory stimuli were presented. Two types of stimuli in each task were used: requiring a response (press the left mouse button) to the target stimuli (20% probability, ‘X’ for the visual task, 2000 Hz tone for the auditory task) or no response to the standard stimuli (80% probability, ‘O’ for the visual task, 1000 Hz for the auditory task). Stimulus duration for the visual task was 0.5 s, and for the auditory task it was 0.05 s; interstimuli intervals were 1.5 s for both tasks. The auditory stimuli were presented via headphones at 60 dB sound pressure level. Stimuli were presented in random order. Reaction times (RTs, in ms) and accuracy of response to the target stimulus (%) were measured.
EEG recording and analysis
EEGs were recorded using a 32-channel Standard Brain Cap (EasyCap GmbH, Herrsching, Germany) with electrode placement according to the International 10–20 System, from positions Fz, Cz and Pz. The sampling rate was 5000 Hz. The ground electrode was placed at AFz. All electrodes were referenced to linked earlobes. The impedance was kept below 5 kΩ. Participants were asked to sit with their eyes closed for 5 min, after which they performed an oddball task.
Off-line processing was performed using Brain Vision Analyzer 2.0 software (Brain Products, Gilching, Germany). Brain activity data at baseline resting periods were filtered using a low cutoff of 0.53 Hz, a high cutoff at 70 Hz, a notch at 50 Hz, and were digitized continuously at 256 Hz with 12-bit resolution [Citation37]. After artifact rejection, data were divided into equal sized segments (5.12 s) and fast Fourier transformations were conducted. Each participant’s data were averaged across the epochs for Fz, Cz and Pz electrodes, and mean absolute power was computed for theta (4–8 Hz), alpha (8–12 Hz) and beta (12–30 Hz) frequency bands.
Data recorded during the oddball task were filtered using 0.01 Hz low cutoff and 70 Hz high cutoff filters. Epochs containing muscle contraction artifacts were rejected manually. Eye movements and blinks were corrected using independent component analysis. The epoch for each ERP stimulus started 150 ms before and ended 800 ms after stimulus. Only correct responses were averaged. The peak amplitudes and latencies of the N1 factor were defined from the first negative peak in the time window of 50–120 ms; N2 was defined as the second negative peak in the time window of 180–270 ms; and P3 was defined as third positive peak in the time window of 240–400 ms. Amplitudes were measured from baseline to peak.
Statistical analysis
The data were tested for normal distribution using the Kolmogorov–Smirnov test, and all were found to be normally distributed. The data are presented as mean and standard deviation (SD). A one-way analyses of variance (ANOVA) using Tukey’s adjustment was conducted to study the effects of the sauna visit of three time levels (before vs end of sauna vs 90-min post-sauna) as within-subject factor on HR and Tre.
A three-way analysis ANOVA (General Linear Model) was used to determine the effects of residual effects of sauna bathing (before vs 90-min post-sauna) as within-subject factor of two levels and trial (CON vs EXP) as within-subject factor of two levels and electrode location (Fz vs Cz, vs Pz) as within-subject factor of three levels on brain neural network arousal (EEG theta, alpha or beta power), cognitive processing (characteristics of ERPs components) and cognitive performance (reaction time or accuracy).
A four-way analysis ANOVA was used to determine the effects of residual effects of sauna bathing (before vs 90-min post-sauna recovery) as within-subject factor of two levels and trial (CON vs EXP) as within-subject factor of two levels and electrode location (Fz vs Cz, vs Pz) as within-subject factor of three levels and task modalities (auditory vs visual) on cognitive processing (characteristics of ERPs components) and cognitive performance (reaction time or accuracy).
If significant effects were found, Tukey’s post-hoc adjustment was used for multiple comparisons within each repeated-measure ANOVA. In addition to significant effect, the statistical observed power (OP) was performed and the partial eta squared (η2) value was estimated as a measure of the residual sauna effect size. A dependent-sample t tests were used to localize the time point difference at each separate electrode location as within-subjects factor. Statistical significance was defined as p < .05. Statistical analyses were performed using IBM SPSS Statistics software (v. 22; IBM Corp., Armonk, NY, USA).
Results
Effect of sauna bathing on physiological parameters
Sauna bathing induced a significant Tre increase (from 37.11 ± 0.33 °C to 38.84 ± 0.32 °C). The participants’ HR rose significantly from 65.63 ± 9.39 beats per minutes ( bpm) to 151.00 ± 21.82 bpm during exposure. Their absolute sweat loss was 1.30 ± 0.33 kg (1.57 ± 0.10% from total body mass). At 90 min after the sauna, Tre (37.00 ± 0.29 °C) and HR (72.14 ± 2.80 bpm) recovered to baseline levels with no significant changes. In the CON trial the Tre and HR values did not change significantly.
Residual effects of sauna bathing on brain neural network activity
No changes in theta and beta activity were found between trials (CON vs EXP) before and 90 min after the sauna (. Alpha power increased significantly 90 min after the sauna in Fz, Cz and Pz electrodes compared with before exposure and did not change in any measured electrode in the CON trial.
Figure 2. Mean absolute powers presented using a base 10 logarithmic scale for the Fz, Cz, and Pz electrodes (top). The mean absolute powers for the theta (4–8 Hz), alpha (8–12 Hz) and beta (12–30 Hz) frequency bands at three EEG sites (Fz, Cz, and Pz) before and 90 min after the sauna for the control (CON) vs experimental (EXP) trials are shown on the lower panel. Values are shown as the mean ± SEM; *p <.05 compared with before.
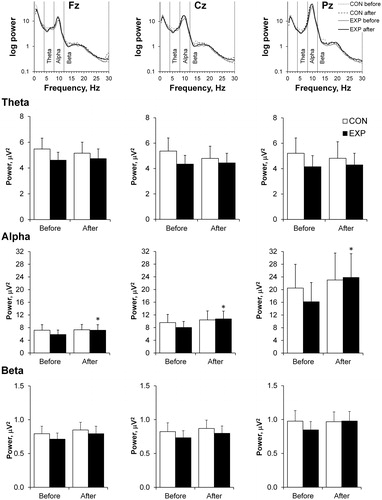
Residual effects of sauna bathing on information processing
There were no main interaction effects (p > .05) on the differences between the auditory and visual task modalities for evoked ERPs component characteristics.
Visual task
In both trials (CON and EXP), latencies of the visual N1, N2 and P3 components did not change significantly after sauna resting compared with before (). No effects were found on the amplitude of the N1 component (CON vs EXP; before vs 90 min after the sauna), and on the amplitudes of the N2 and P3 components (before vs 90 min after the sauna) in the CON trial. The amplitude of the visual target N2 component was significantly higher 90 min after sauna for the Cz and Pz electrodes compared with before (p < .05, η2 > 0.25, OP > 0.5), but no significant differences were found comparing visual standard N2 amplitudes before and 90 min after the sauna. The amplitude of visual target P3 component decreased significantly 90 min after the sauna for all measured electrodes compared with before (p < .05, η2 > 0.3, OP > 0.6).
Figure 3. Grand-averaged event-related potential (ERP) waveforms at three EEG sites (Fz, Cz, and Pz) before and 90 min after the sauna for the control (CON) vs experimental (EXP) trials for visual tasks are shown on the left. The mean N1, N2, and P3 amplitudes at three sites (Fz, Cz, Pz) before and after rest for the CON vs EXP trials for visual tasks are shown on the right. Values are shown as the mean ± SD; *p <.05 compared with before. Note. N.D: Not Detected; the response of ERPs P3 component in the 80% non-target (standard) condition was too small to compute a reliable mean response for amplitude and latency.
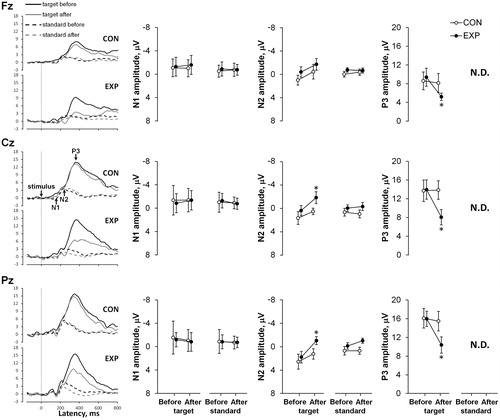
Auditory task
No effects of post-sauna recovery on auditory N1, N2 and P3 latencies were found between trials (CON vs EXP) before and after (. Amplitudes of the N1 component in CON vs EXP trials and amplitudes of the N2 and P3 components in the CON trial did not change significantly (before vs 90 min after the sauna). No changes were found for auditory target N2 amplitudes comparing before with 90 min after the sauna for all measured electrodes. Auditory standard N2 wave amplitudes were significantly smaller 90 min after the sauna for the Cz and Pz electrodes (p < .01, η2 > 0.35, OP > 0.80) compared with before. The amplitudes of auditory target P3 decreased significantly 90 min after sauna exposure for all measured electrodes (p < .01, η2 > 0.35, OP > 0.70).
Figure 4. Grand-averaged event-related potential (ERP) waveforms at three EEG sites (Fz, Cz, and Pz) before and 90 min after the sauna for the control (CON) vs experimental (EXP) trials for auditory tasks are shown on the left. The mean N1, N2, and P3 amplitudes at three sites (Fz, Cz, and Pz) before and after rest for the CON vs EXP trials for auditory tasks are shown on the right. Values are shown as the mean ± SD; *p <.05 compared with before. Note. N.D: Not Detected; the response of ERPs P3 component in the 80% non-target (standard) condition was too small to compute a reliable mean response for amplitude and latency.
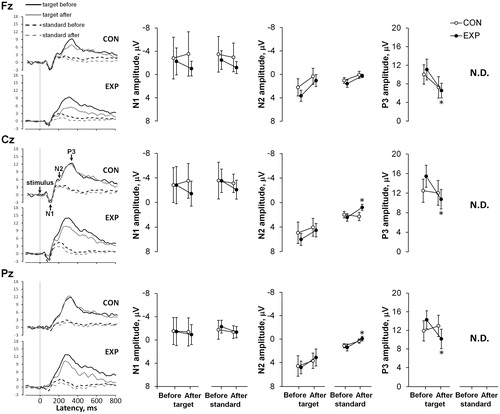
Residual effects of sauna on cognitive performance
No significant differences in task performances were found comparing values before versus after sauna bathing or in experimental versus control conditions (). In addition, there were no main interaction effects (p >.05) on the differences between the auditory and visual task modalities for cognitive performance variables.
Table 1. Mean response time (ms) and mean percentage (%) of correct responses in the target condition.
Discussion
Traditional Finnish sauna exposure in our study induced moderate to severe whole-body hyperthermia (Tre 38.5–39.6 °C). Given that the brain (jugular-to-arterial) temperature is at least ∼0.3 °C higher than that of the body core with or without hyperthermia [Citation14], this indicates that sauna bathing in this study led to even greater brain temperatures (heat stress) in respect to changes in body core temperature. However, such hyperthermia reduces cerebral blood flow by ∼30% and increases cerebral metabolic demands by ∼10% [Citation38]. Moreover, the physiological range of hyperthermia (38.5–39.5 °C) elicits breakdown of the blood–brain barrier leading to permeability (and possibly associated edema) which appears relatively quickly (>20 min) [Citation24], suggesting minor hyperthermia-induced morphological changes to water and ion contents in the brain [Citation39]. This has been shown to modulate neural functions strongly [Citation24]. Along with increased brain heat stress, lower blood energy supply, impairments in the brain neural network [Citation12,Citation13] and metabolism [Citation14,Citation19] have been shown to influence cognitive processing and performance [Citation15,Citation40–42]. Indeed, when the total cognitive resources are insufficient for both the task and dealing with thermal stress [Citation41], complex cognitive task performances that require processing for calculation, memorizing and logical thinking deteriorate [Citation43]. On the other hand, a moderate elevation in brain temperature has been suggested to benefit cognitive function because all chemical processes, including synaptic functions and the propagation of action potentials, are temperature dependent and elevated brain temperature may result in slightly faster transmission of signals in the central nervous system leading to marginally faster performances in simple choice reaction tests [Citation24,Citation44]. Thus, in view of this paradigm on how heat stress on the brain might affect structural, metabolic, and functional homeostasis, the aim of our study was to examine whether cognitive processing and performance would persist into residual post-sauna (whole-body hyperthermia) recovery.
As expected, we found an increase in resting (pre-test) EEG alpha power (but no change in theta or beta activity) with distribution along the frontoparietal axis following sauna recovery (), suggesting enhanced relaxation in the brain neural network (i.e., reduced cortical activity). These data are in agreement with those observed after high-intensity exercise, where a post-exercise increase in alpha power activity has been shown in the frontal brain region—the region related to emotional processes—and has been accompanied by subjective experiences of high sense of well-being and/or positive emotions [Citation45]. Higher alpha power has been shown to be associated with reduced cortical activity and has been described as ‘cortical idling’, with a greater availability of resources for engagement in cognitive tasks [Citation33]. However, as a task is engaged, alpha power activity decreases in the occipital, parietal and frontal areas of the brain [Citation40,Citation46] and conversely, an increase in theta power relative to rest has been reported for working memory [Citation47] and concentration tasks [Citation48]. However, here we did not measure theta, alpha and beta power activity during the cognitive task, as our analysis points to an absence of modification in cognitive processing (ERP components) following residual sauna recovery.
Table 2. Generalized overview of 90-min post-sauna effect on neural network arousal, information processing and cognitive behavior.
We found no effects of post-sauna recovery on the visual and auditory N1 components (latency and amplitude), indicating that sensory processing [Citation28] was intact during these task modalities (). However, in the visual task modality we found that post-sauna recovery led to enhanced amplitude of the N2 component with centroparietal distribution and decreased amplitude of the P3 component with distribution along the frontoparietal axis only for executive (‘Target’ trial) motor–cognitive processing. For the auditory task modality, post-sauna recovery led to a decrease in the P3 amplitude and was found for executive cognitive processing with a frontoparietal distribution, and this change was accompanied by auditory N2 amplitude enhancement along a centroparietal distribution only for standard cognitive processing. Taken together, these results are not in agreement with a previous observation [Citation49] that the more pronounced effects seen on auditory than on visual tasks suggest that the brain systems supporting auditory processing might be more vulnerable to stress and post-stress recovery conditions than those supporting visual processing [Citation35].
Intriguingly, those post-sauna recovery modifications in ERP components (stimulus processing) were insufficient to affect cognitive performance (reaction time and accuracy) in both visual and auditory task modalities. This result might in part be explained by post-sauna recovery leading to increases in the attentional resources allocated to stimulus discrimination (ERP-N2) which presumably acts as a modulator for compensation for the reduced attentional recourses allocated to stimulus categorization (ERP-P3). Considering that the original P3 component involves much greater available processing (attentional) resources (i.e., area under the curve) than the N2 component for the same task ( and ), we suggest that fewer cognitive recourses were allocated for the same cognitive performance efficiency (i.e., reaction time and accuracy) following sauna recovery. From a functional point of view, as the body cools down from acute short-term whole-body hyperthermia, the potentiated modulation involving fewer cognitive processing resources in respect to the same cognitive performance (behavioral) efficiency might be regarded as a way to increase the ability to maintain mental working capacity for a longer duration.
Conclusions
The data obtained in this study suggest that recovery to normothermia after a sauna leads to a greater resting neural network relaxation followed by increases in cognitive processing economy for a given oddball task. By contrast with our expectation, the auditory processing was not affected more by post-sauna recovery than was visual processing. Post-sauna recovery modifications in ERP components (stimulus processing) were insufficient to affect cognitive performance (reaction time and accuracy) in both visual and auditory task modalities.
Acknowledgements
The authors acknowledge the subjects who took part in the study.
Disclosure statement
No potential conflict of interest was reported by the authors.
Additional information
Funding
References
- Laukkanen T, Khan H, Zaccardi F, et al. Association between sauna bathing and fatal cardiovascular and all-cause mortality events. JAMA Intern Med. 2015;175:542–548.
- Laukkanen T, Kunutsor S, Kauhanen J, et al. Sauna bathing is inversely associated with dementia and Alzheimer’s disease in middle-aged Finnish men. Age Ageing. 2017;46:245–249.
- Isomäki H. The sauna and rheumatic diseases. Ann Clin Res. 1988;20:271–275.
- Nurmikko T, Hietaharju A. Effect of exposure to sauna heat on neuropathic and rheumatoid pain. Pain. 1992;49:43–51.
- Laukkanen JA, Laukkanen T. Sauna bathing and systemic inflammation. Eur J Epidemiol. 2018;33:351–353.
- Hasan J, Karvonen MJ, Piironen P. Special review. I. Physiological effects of extreme heat as studied in the Finnish ‘sauna’ bath. Am. J. Phys. Med. 1966;45:296–314.
- Laitinen LA, Lindqvist A, Heino M. Lungs and ventilation in sauna. Ann Clin Res. 1988;20:244–248.
- Toda M, Morimoto K, Nagasawa S, et al. Change in salivary physiological stress markers by spa bathing. Biomed Res. 2006;27:11–14.
- Hayasaka S, Nakamura Y, Kajii E, et al. Effects of charcoal kiln saunas (Jjimjilbang) on psychological states. Complement Ther Clin Pract. 2008;14:143–148.
- Hannuksela ML, Ellahham S. Benefits and risks of sauna bathing. Am J Med. 2001;110:118–126.
- Leppäluoto J, Tuominen M, Väänänen A, et al. Some cardiovascular and metabolic effects of repeated sauna bathing. Acta Physiol Scand. 1986;128:77–81.
- Nybo L, Nielsen B. Perceived exertion is associated with an altered brain activity during exercise with progressive hyperthermia. J Appl Physiol. 2001;91:2017–2023.
- Rasmussen P, Stie H, Nybo L, et al. Heat induced fatigue and changes of the EEG is not related to reduced perfusion of the brain during prolonged exercise in humans. J Therm Biol. 2004;29:731–737.
- Nybo L, Møller K, Volianitis S, et al. Effects of hyperthermia on cerebral blood flow and metabolism during prolonged exercise in humans. J Appl Physiol. 2002;93:58–64.
- Racinais S, Gaoua N, Grantham J. Hyperthermia impairs short-term memory and peripheral motor drive transmission. J Physiol (Lond). 2008;586:4751–4762.
- Brazaitis M, Eimantas N, Daniuseviciute L, et al. Two strategies for the acute response to cold exposure but one strategy for the response to heat stress. Int J Hyperthermia. 2015;31:325–335.
- Brothers RM, Wingo JE, Hubing KA, et al. The effects of reduced end-tidal carbon dioxide tension on cerebral blood flow during heat stress. J Physiol (Lond). 2009;587:3921–3927.
- Wilson TE, Cui J, Zhang R, et al. Heat stress reduces cerebral blood velocity and markedly impairs orthostatic tolerance in humans. Am J Physiol Regul Integr Comp Physiol. 2006;291:R1443–R1448.
- Shibasaki M, Namba M, Oshiro M, et al. Suppression of cognitive function in hyperthermia; From the viewpoint of executive and inhibitive cognitive processing. Sci. Rep. 2017;7:43528.
- Thomas MM, Cheung SS, Elder GC, et al. Voluntary muscle activation is impaired by core temperature rather than local muscle temperature. J Appl Physiol. 2006;100:1361–1369.
- Craig AD. Interoception: the sense of the physiological condition of the body. Curr Opin Neurobiol. 2003;13:500–505.
- Nakamura K, Morrison SF. Preoptic mechanism for cold-defensive responses to skin cooling. J Physiol (Lond). 2008;586:2611–2620.
- Vay L, Gu C, McNaughton Peter A. The thermo-TRP ion channel family: properties and therapeutic implications. Br J Pharmacol. 2012;165:787–801.
- Kiyatkin EA. Brain temperature homeostasis: physiological fluctuations and pathological shifts. Front Biosci (Landmark Ed). 2010;15:73–92.
- Kiyatkin EA. Brain hyperthermia as physiological and pathological phenomena. Brain. Brain Res Brain Res Rev. 2005;50:27–56.
- Boksem MAS, Meijman TF, Lorist MM. Effects of mental fatigue on attention: an ERP study. Brain Res Cogn Brain Res. 2005;25:107–116.
- Kertzman S, Lowengrub K, Aizer A, et al. Go-no-go performance in pathological gamblers. Psychiatry Res. 2008;161:1–10.
- Näätänen R. The role of attention in auditory information processing as revealed by event-related potentials and other brain measures of cognitive function. Behav Brain Sci. 1990;13:201–233.
- Donchin E, Coles MGH. Is the P300 component a manifestation of context updating? Behav Brain Sci. 1988;11:357–374.
- Picton TW. The P300 Wave of the human event-related potential. J Clin Neurophysiol. 1992;9:456–479.
- Shibasaki M, Namba M, Oshiro M, et al. The effect of elevations in internal temperature on event-related potentials during a simple cognitive task in humans. Am J Physiol – Regul Integr Comp Physiol. 2016;311:R33–R38.
- Lucas RAI, Sarma S, Schlader ZJ, et al. Age-related changes to cardiac systolic and diastolic function during whole-body passive hyperthermia. Exp Physiol. 2015;100:422–434.
- Klimesch W. EEG alpha and theta oscillations reflect cognitive and memory performance: a review and analysis. Brain Res Brain Res Rev. 1999;29:169–195.
- Lang W, Lang M, Kornhuber A, et al. Event-related EEG-spectra in a concept formation task. Hum Neurobiol. 1988;6:295–301.
- Duncan CC, Kosmidis MH, Mirsky AF. Closed head injury-related information processing deficits: an event-related potential analysis. Int J Psychophysiol. 2005;58:133–157.
- Cernych M, Baranauskiene N, Eimantas N, et al. Physiological and psychological responses during exercise and recovery in a cold environment is gender-related rather than fabric-related. Front Psychol. 2017;8:1344.
- Griskova I, Ruksenas O, Dapsys K, et al. The effects of 10 Hz repetitive transcranial magnetic stimulation on resting EEG power spectrum in healthy subjects. Neurosci Lett. 2007;419:162–167.
- Bain AR, Nybo L, Ainslie PN. Cerebral vascular control and metabolism in heat stress. Compr. Physiol. [Internet]. John Wiley & Sons, Inc. 2015;5(3):1345–1380. [cited 2017 Sep 15]. Available from: http://onlinelibrary.wiley.com/doi/10.1002/cphy.c140066/abstract.
- Zlokovic BV. The blood–brain barrier in health and chronic neurodegenerative disorders. Neuron. 2008;57:178–201.
- Gaoua N, Herrera CP, Périard JD, et al. Effect of passive hyperthermia on working memory resources during simple and complex cognitive tasks. Front Psychol. 2018;8:2290.
- Hocking C, Silberstein RB, Lau WM, et al. Evaluation of cognitive performance in the heat by functional brain imaging and psychometric testing. Comp Biochem Physiol Part A Mol Integr Physiol. 2001;128:719–734.
- McMorris T, Swain J, Smith M, et al. Heat stress, plasma concentrations of adrenaline, noradrenaline, 5-hydroxytryptamine and cortisol, mood state and cognitive performance. Int J Psychophysiol. 2006;61:204–215.
- Simmons SE, Mündel T, Jones DA. The effects of passive heating and head-cooling on perception of exercise in the heat. Eur J Appl Physiol. 2008;104:281–288.
- Serwah N, Marino FE. The combined effects of hydration and exercise heat stress on choice reaction time. J Sci Med Sport. 2006;9:157–164.
- Brummer V, Schneider S, Abel T, et al. Brain cortical activity is influenced by exercise mode and intensity. Med Sci Sports Exerc. 2011;43:1863–1872.
- Gundel A, Wilson GF. Topographical changes in the ongoing EEG related to the difficulty of mental tasks. Brain Topogr. 1992;5:17–25.
- Mizuhara H, Wang L-Q, Kobayashi K, et al. A long-range cortical network emerging with theta oscillation in a mental task. Neuroreport. 2004;15:1233–1238.
- Jensen O, Tesche CD. Frontal theta activity in humans increases with memory load in a working memory task. Eur J Neurosci. 2002;15:1395–1399.
- Duncan CC, Kosmidis MH, Mirsky AF. Event-related potential assessment of information processing after closed head injury. Psychophysiology. 2003;40:45–59.