Abstract
Introduction: Percutaneous image-guided cryo- (CA) and radiofrequency- (RFA) ablations have been widely used in the treatment of painful bone metastases (BM). However, paucity of data is available for the performance of these treatments when used with a curative intent. The aim of this study is to investigate the local progression free-survival (LPFS) after radical percutaneous image-guided ablation of BM in oligometastatic patients, and to identify predictive factors associated with local tumor progression.
Materials and Methods: This is a retrospective review of all patients who underwent percutaneous image-guided CA or RFA of BM with a radical intent between 2007 and 2018.
Results: Forty-six patients with a total of forty-nine BM underwent percutaneous image-guided CA (N = 37; 75,5%) or RFA (N = 12; 24,5%). Primary malignancies included thyroid (N = 11, 22.5%), breast (N = 21; 42.9%), lung (N = 8; 16.3%) and other (N = 9; 18,3%) cancers. Additional consolidation was performed after ablation in 20.4% cases (N = 10). Mean follow-up was 34.1 ± 22 months. Local progression at the treated site was observed in 28.5% cases (N = 14); 1- and 2-year LPFS was 76.8% and 71.7%, respectively. Size of BM (>2 cm) predicted local tumor progression (p = .002).
Conclusions: Percutaneous image-guided locoregional therapies used in the radical treatment of BM in oligometastatic patients demonstrate significant rates of LPFS providing the size of BM ≤2 cm.
Introduction
Bone is the third most common site of metastasis, closely behind the lung and liver which are the most common sites [Citation1]; and primaries with a greater propensity for bone metastases, such as lung, breast, and prostate cancers account for 45% of commonly diagnosed cancers [Citation2].
Symptomatic treatment of bone metastases (BM) is often necessary due to skeletal related events [Citation3]. Nevertheless, in selected patients such as those presenting with oligometastatic disease [Citation4–7], radical treatments may be possible with the intent of increasing disease-free (DFS) and overall survival (OS).
Recently, minimally-invasive percutaneous image-guided locoregional treatments [Citation5,Citation8,Citation9] have been introduced for the treatment of BM. In particular, in the past two decades, radiofrequency- (RFA) and cryo-ablation (CA) have been successfully applied for the symptomatic treatment of painful BM [Citation10–13]; and to a lesser extent, both these techniques have also been used with a curative intent [Citation14,Citation15] as an alternative to the surgery or radiation therapy (RT).
The aim of this retrospective single-institution study is to investigate the local progression free-survival (LPFS) after radical percutaneous image-guided locoregional treatments of BM; and to identify factors associated with local tumor progression.
Materials and methods
This retrospective study did not receive any funding and was approved by the local Institutional Review Board. Written informed consent was waived for retrospective study participation.
Population
Between January 2008 and November 2017, 46 patients (16 male; 30 female; mean age 59 ± 11.6 year old; median 61; IC 95% 55.5 – 62.4) underwent percutaneous image-guided locoregional treatments of 49 BM with a radical intent. Patients were selected by a multidisciplinary tumor board according to the following criteria: Eastern Cooperative Oncology Group (ECOG) performance status (PS) ≤ 2; oligometastatic status (≤3 bone or visceral metastases), oligo-progression in one bone site and/or slow-evolving disease; contraindication to surgery and/or RT due to patients refusal, comorbidities, unacceptable delay to systemic therapies or other technical causes (e.g. maximal radiation dose delivered to the same anatomic site). Patient demographics and characteristics of BM are summarized in .
Table 1. Patient demographics and BM characteristics.
Percutaneous image-guided locoregional treatments
All interventions were performed under conscious sedation or general anesthesia in a strict sterile environment. Computed Tomography (CT)-guidance alone or both CT and fluoroscopic guidance were systematically used.
RFA or CA were applied at the discretion of the interventional radiologist performing the procedure. One or more cryoprobes (IceSphere®, IceRod® or IceForce® Galil medical, Yokneam, Israel) or electrodes (Cool-tip or Osteo-Cool, Medtronic, USA) resulting in different shapes and sizes of the ablation zone were used, often simultaneously to achieve a synergistic effect.
Radical treatment was performed by ensuring a large ablation area that would cover the entire BM with an additional safety margin of at least 5–10 mm.
When non-target critical structures such as nerves were in close proximity (≤1 cm) to the target BM, protective measures were applied [Citation16].
According to the type and location of BM, with particular consideration to biomechanics [Citation17], percutaneous bone consolidation (i.e. percutaneous osteoplasty, osteosynthesis, or combination of both) was performed immediately after ablation within the same interventional session to prevent bone fracture at the treated site (.
Figure 1. Forty year old female patient with a history of breast cancer; (A, B) the patient was referred for a single, lytic (17 mm) FDG-avid metastasis of L1 (arrows). (C, D) The patient was treated with a single cryoablation probe (white arrows) combined with thermal monitoring and hydro-dissection of the epidural space (black arrow). (E) The procedure was followed by bone consolidation (i.e. vertebroplasty). (F, G) PET-CT follow-up 3 years later showed no FDG uptake in the treated area nor in other visceral or bone sites.
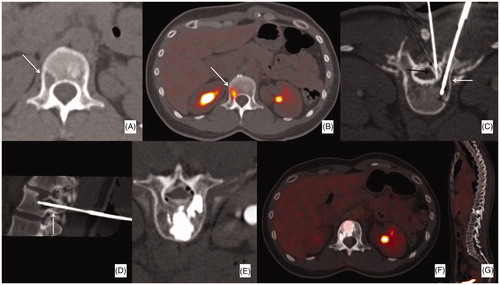
Collected data
The following data were collected from each patient’s electronic records:
Sex and age at the time of locoregional treatment; histology of the primary cancer; metastatic status;
Target BM location, size, radiographic features, status of the overlying cortical bone, presence of synchronous primary tumor, concomitant treatments other than ablation, proximity to nearby non-target critical structures;
Type of ablation and anesthesia, the need for protective measures and consolidation, complications encountered.
The metastatic status was defined by the burden of systemic disease including bone and visceral organs.
BM location was categorized into four different groups including the spine, pelvic ring, long bone, and others.
The size of the target BM was selected according to the maximum diameter observed on the most recent multiplanar cross-sectional imaging available before ablation.
Radiographic feature of the target BM was categorized into lytic, blastic or mixed.
Non-target critical structures were considered as those (e.g. nerves, joints, visceral or parenchymal organs) in close proximity to the target BM (≤1 cm) thus, being at risk for unintentional thermal-induced injury. In these cases, protective measures were applied during ablation.
Procedure-related complications were evaluated according to the National Cancer Institute
Common Terminology Criteria for Adverse Events version 5.0 (NCI CTC-AE V5).
Systematic cross-sectional imaging (contrast-enhanced CT or MRI, PET-CT) performed regularly every 3 to 6 months to monitor the systemic disease and including the treated bone within the field of view, was retrospectively reviewed to evaluate local progression at the treated site; which was defined as any BM increase in size and/or contrast-enhancement or radiotracer uptake on the most recent imaging follow-up available as compared to baseline imaging. Local progression-free survival (LPFS) refers to the time interval between the locoregional treatment and the development of local tumor progression at the treated site. Bone disease-free survival (BDFS - defined as the time interval between the locoregional treatment and disease progression in the treated BM or in another bone) and disease-free survival (DFS – defined as the time interval between the locoregional treatment and the time of any visceral or bone tumor progression) were also evaluated. Finally, the most recent clinical notes available on the medical records were assessed to evaluate the overall survival (OS).
Data analysis
Basic descriptive statistics were used for continuous variables. An univariate analysis was conducted to identify factors predicting local progression at the treated site; factors reporting a p values < .1 entered a multivariate model.
The Kaplan-Meier method was used to outline LPFS, BDFS, DFS and OS; and the log-rank test to stratify these results according to tumor histology. All significance levels were set at a 0.05 value.
Statistical analysis was performed by using SAS 9.4.
Results
Forty-nine BM (mean size 27.7 ± 14.6 mm; median 24 mm; IC95% 23.5–31.9) were treated with RFA (N = 12; 24.5%) or CA (N = 37; 75.5%). Target BM originated from thyroid (N = 11, 22.5%), breast (N = 21; 42.9%), lung (N = 8; 16.3%) or other (N = 9; 18,3%) cancers.
The pelvic ring (N = 27; 55.1%) and the spine (N = 10; 20.4%) were the most common treated bone regions; lytic lesion was the most common radiographic feature of the target BM (N = 32, 65.3%).
Nerves were the most common critical non-target structure being close to the treated BM (N = 34, 69.4%) thus, necessitating protective measures during the ablation in more than half of the cases (N = 29; 59.1%).
Additional consolidation followed the ablation in 20.4% cases (N = 10).
Complications were observed in 20.4% cases (N = 10; ): post-operative pain (grade 2 complication) was noted in eight patients; and two grade 3 complications requiring interventional or surgical treatments were noted in two cases.
Table 2. Complications.
Mean follow-up was 34.1 ± 22 months (median 30.5; IC95% 27.6–40.7). Local tumor progression at the treated site was noted in 28.5% cases (N = 14); 1- and 2-year LPFS was 76.8% and 71.7%, respectively (. Only BM size >2 cm was associated with local tumor progression () thus, impeding multivariate analysis. BDFS was 71.7% and 53.1% at 1- and 2-year follow-up, respectively. At the same time interval, DFS was 86.3% and 61.5%, respectively. Lastly, 1- and 2-year OS was 95.4%. LPFS, BDFS, DFS and OS did not show any significant difference when stratified according to tumor histology (.
Table 3. Univariate analysis of factors predicting local recurrence at the treated site.
Discussion
Radical treatment of BM is challenging and often requires an integrated multidisciplinary approach [Citation18–21]. Surgery and RT have traditionally been the treatment of choice in such a clinical scenario. However, due to patients’ pre-existing comorbidities and the fact that surgery often delays systemic treatment, the opportunities of radical surgery have often been narrow [Citation20]. Nowadays, radical surgery is mainly reserved to patients with good life expectancy and PS, very limited burden of systemic disease, and favorable tumor histotype (e.g. thyroid or kidney cancer), especially when the BM is associated with a pathologic or an impending fracture. To achieve effective local tumor control, radical surgery is often combined with RT [Citation22], of which the field of action has progressively increased in the past few years as a result of the introduction of stereotactic body radiation therapy (SBRT); which offers high rate of local tumor control (around 90% at 1-year [Citation23]) and better rates of pain management as compared to standard external beam radiation therapy (EBRT) [Citation24]. Wang et al. reported on 166 mechanically stable, non-cord-compressing spinal BM originating from various primaries in 149 patients undergoing SBRT [Citation25]. Their inclusion criteria was similar to those applied in the present study (e.g. oligometastatic disease, failure of previous conventional RT or surgery, residual tumor after surgery, medical inoperability, and refusal to undergo surgery); and similarly, their oncologic results were analogous to those obtained with percutaneous image-guided ablation in the present series: the rate of local tumor progression reported by Wang et al. is 28% (vs. 28.5% in the present series), resulting in 1- and 2-year LPFS 80.5% and 72.4%, respectively (vs. 76.8% and 71.7%, respectively in the present series).
Compared to RT [Citation18], percutaneous image-guided locoregional therapies can achieve effective tumor destruction irrespective of the tumor histology, as confirmed by the similar LPFS rates obtained in the present series among all the different tumor histologies. Nevertheless, it should be noted that effective local tumor control provided by ablation is strictly dependent on the size of the target BM as local progression is much more likely to occur when BM >2 cm are treated.
This finding is concordant with literature which has reported that tumor size represents the most critical factor affecting local tumor progression when percutaneous ablation is performed, irrespective of the target organ treated and the primary or metastatic nature of the treated tumor [Citation26–31]. In the present series, most (67%) of the treated BM were ≤2 cm in size, which may explain the slightly better rate of local tumor control achieved at 1-year follow-up as compared to other similar series (Deschamps et al. reported 67% local control at 1-year in a population with several different BM histology; however, 2/3 treated BM were ≥20 cm [Citation14]; similarly, Ma et al reported 68% local tumor control (median BM diameter 3.6 cm) at median 10-month follow-up in a population of 45 patients presenting with non-small cell lung cancer BM [Citation15]). Concordant with Deschamps et al. [Citation14], the present series confirmed the critical threshold of 2 cm to limit tumor progression after the radical treatment of BM. Moreover, Deschamps et al. [Citation14] demonstrated that lower local progression rates at the site of treated BM are expected after percutaneous locoregional treatments when small (<2 cm), metachronous BM without cortical disruption and no critical neurological structure within their vicinity are treated. Most of these factors are in favor of a less-aggressive disease; which was probably the case in the current study in view of the high OS and the relative high BDFS and DFS rates; nevertheless, due to the several different tumor histologies included, OS, BDFS and DFS rates should be interpreted with caution.
Recently, a role for potential radical treatment of BM has also been reported with Magnetic Resonance-Guided Focused Ultrasound (with 33.3% complete or partial response observed) [Citation32]; however, it is still generally accepted that the primary role of this technique is mainly palliative in the setting of BM and that, although local tumor control may be an added value, further investigations are needed to validate the technique [Citation33].
Additionally, compared to the other non-percutaneous techniques such as RT and MRgFUS, percutaneous ablation offers the advantage of combining bone consolidation with ablation within the same intervention, thus significantly reducing the risk of secondary fracture at the treated site. Such combined approach, which is a common practice for interventional radiologists [Citation31], allows rapid patient’s mobilization [Citation34–37] and optimal pain control, which can be additionally improved if RT is added [Citation12]. This possibility of combining ablation and consolidation represents a major advantage of percutaneous locoregional treatments over non-percutaneous techniques, particularly SBRT, which results in secondary fractures in 9–11% cases [Citation23]. In the spine, where most of the SBRT experience lies, vertebral compression fractures can occur in more than 1/3 of the treated vertebrae [Citation23]; and increased risk is seen in patients with a history of prior vertebral fracture, single vertebral level treated, high dose of radiation, and lytic BM [Citation38,Citation39]. Nevertheless, it should be noted that aside from the spine and the pelvic area where percutaneous consolidation is highly effective, sub-optimal results are obtained with percutaneous consolidation in long bones [Citation40,Citation41], especially when diaphysis or peri-articular tumor involvement is present. In such cases, surgery still remains the preferred treatment from a biomechanical point of view.
Percutaneous locoregional treatments performed with a radical intent in the present series have shown to be safe; most (N = 8/10; 80%) of the complications were minor and merely required analgesics for the management of post-operative pain, which typically occurs within the first few days after ablation. As to the other two (N = 2/10; 20%) grade 3 complications encountered in this series, tumor seeding was not foreseeable; and post-ablation fracture of the scapula stresses the importance of combined ablation and consolidation within the same intervention.
Limitations of the present study include its retrospective nature and the small sample size (including also various tumor histologies), which may have limited the possibility to investigate factors other than BM size in predicting local tumor progression.
In conclusion, percutaneous image-guided locoregional therapies used in the treatment of BM with a radical intent are associated with significant rates of LPFS providing BM size ≤2 cm. These results may be also related to the reduced aggressiveness of the included tumors, as suggested by the high OS and the relatively high BDFS and DFS. Nevertheless, based on these results and the possible combination of ablation and consolidation in the same interventional session, it is reasonable to include percutaneous locoregional treatments into the current therapeutic armamentarium available to the multidisciplinary tumor board for the radical treatment of BM.
Disclosure statement
No potential conflict of interest was reported by the authors.
Authors 1, 9 and 10 are proctors for Medtronic. Authors 9 and 10 are Proctors for Galil Medical.
References
- Hage WD, Aboulafia AJ, Aboulafia DM. Incidence, location, and diagnostic evaluation of metastatic bone disease. Orthop Clin North Am. 2000;31:515–528.
- Kelly M, Lee M, Clarkson P, et al. Metastatic disease of the long bones: a review of the health care burden in a major trauma centre. Can J Surg. 2012;55:95–98.
- Saad F, Lipton A, Cook R, et al. Pathologic fractures correlate with reduced survival in patients with malignant bone disease. Cancer. 2007;110:1860–1867.
- Cazzato RL, Bonichon F, Buy X, et al. Over ten years of single-institution experience in percutaneous image-guided treatment of bone metastases from differentiated thyroid cancer. Eur J Surg Oncol. 2015;41:1247–1255.
- Cazzato RL, Buy X, Grasso RF, et al. Interventional Radiologist's perspective on the management of bone metastatic disease. Eur J Surg Oncol. 2015;41:967–974.
- Gangi A, Buy X. Percutaneous bone tumor management. Semin Intervent Radiol. 2010;27:124–136.
- Gangi A, Tsoumakidou G, Buy X, et al. Quality improvement guidelines for bone tumour management. Cardiovasc Intervent Radiol. 2010;33:706–713.
- Barile A, Arrigoni F, Zugaro L, et al. Minimally invasive treatments of painful bone lesions: state of the art. Med Oncol. 2017;34:53
- Barile A, Arrigoni F, Bruno F, et al. Present role and future perspectives of interventional radiology in the treatment of painful bone lesions. Future Oncol. 2018. doi: 10.2217/fon-2017-0657.
- Goetz MP, Callstrom MR, Charboneau JW, et al. Percutaneous image-guided radiofrequency ablation of painful metastases involving bone: a multicenter study. JCO. 2004; 22:300–306.
- Callstrom MR, Dupuy DE, Solomon SB, et al. Percutaneous image-guided cryoablation of painful metastases involving bone: multicenter trial. Cancer. 2013;119:1033–1041.
- Cazzato RL, Garnon J, Caudrelier J, et al. Percutaneous radiofrequency ablation of painful spinal metastasis: a systematic literature assessment of analgesia and safety. Int J Hyperthermia. 2018;1. doi: 10.1080/02656736.2018.1425918.
- Cazzato RL, Garnon J, Caudrelier J, et al. Low-power bipolar radiofrequency ablation and vertebral augmentation for the palliative treatment of spinal malignancies. Int J Hyperthermia. 2018;1. doi: 10.1080/02656736.2017.1422557.
- Deschamps F, Farouil G, Ternes N, et al. Thermal ablation techniques: a curative treatment of bone metastases in selected patients? Eur Radiol. 2014;24:1971–1980.
- Ma Y, Wallace AN, Waqar SN, et al. Percutaneous image-guided ablation in the treatment of osseous metastases from non-small cell lung cancer. Cardiovasc Intervent Radiol. 2018;41:726–733.
- Tsoumakidou G, Buy X, Garnon J, et al. Percutaneous thermal ablation: how to protect the surrounding organs. Tech Vasc Interv Radiol. 2011;14:170–176.
- Cazzato RL, Garnon J, Koch G, et al. Percutaneous bone and spine consolidation in cancer patients: who and how? Poster (awarded Magna cum Laude) session presented at: Education Exhibit 103rd Scientific Assembly and Annual Meeting of the Radiological Society of North America; Nov 26–Dec 1; Chicago, Illinois.
- Spratt DE, Beeler WH, de Moraes FY, et al. An integrated multidisciplinary algorithm for the management of spinal metastases: an International Spine Oncology Consortium report. Lancet Oncol. 2017;18:e720–e730.
- Quan GMY, Pointillart V, Palussière J, et al. Multidisciplinary treatment and survival of patients with vertebral metastases from thyroid carcinoma. Thyroid. 2012;22:125–130.
- Capanna R, Piccioli A, Di Martino A, et al. Management of long bone metastases: recommendations from the Italian Orthopaedic Society bone metastasis study group. Expert Rev Anticancer Ther. 2014;14:1127–1134.
- Horn SR, Dhillon ES, Poorman GW, et al. Epidemiology and national trends in prevalence and surgical management of metastatic spinal disease. J Clin Neurosci. 2018;53:183–187.
- Tseng CL, Eppinga W, Charest-Morin R, et al. Spine stereotactic body radiotherapy: indications, outcomes, and points of caution. Global Spine J. 2017;7:179–197.
- Husain ZA, Sahgal A, De Salles A, et al. Stereotactic body radiotherapy for de novo spinal metastases: systematic review. J Neurosurg Spine. 2017;27:295–302.
- Greco C, Pares O, Pimentel N, et al. Spinal metastases: from conventional fractionated radiotherapy to single-dose SBRT. Rep Pract Oncol Radiother. 2015;20:454–463.
- Wang XS, Rhines LD, Shiu AS, et al. Stereotactic body radiation therapy for management of spinal metastases in patients without spinal cord compression: a phase 1–2 trial. Lancet Oncol. 2012;13:395–402.
- de Baère T, Aupérin A, Deschamps F, et al. Radiofrequency ablation is a valid treatment option for lung metastases: experience in 566 patients with 1037 metastases. Ann Oncol. 2015;26:987–991.
- Palussiere J, Lagarde P, Aupérin A, et al. Percutaneous lung thermal ablation of non-surgical clinical N0 non-small cell lung cancer: results of eight years’ experience in 87 patients from two centers. Cardiovasc Intervent Radiol. 2015;38:160–166.
- Lanza E, Palussiere J, Buy X, et al. Percutaneous image-guided cryoablation of breast cancer: a systematic review. J Vasc Interv Radiol. 2015;26:1652–1657.e1.
- Psutka SP, Feldman AS, McDougal WS, et al. Long-term oncologic outcomes after radiofrequency ablation for T1 renal cell carcinoma. Eur Urol. 2013;63:486–492.
- Shady W, Petre EN, Gonen M, et al. Percutaneous radiofrequency ablation of colorectal cancer liver metastases: factors affecting outcomes–a 10-year experience at a single center. Radiology. 2016;278:601–611.
- Solbiati L, Ahmed M, Cova L, et al. Small liver colorectal metastases treated with percutaneous radiofrequency ablation: local response rate and long-term survival with up to 10-year follow-up. Radiology. 2012;265:958–968.
- Napoli A, Anzidei M, Marincola BC, et al. Primary pain palliation and local tumor control in bone metastases treated with magnetic resonance-guided focused ultrasound. Invest Radiol. 2013;48:351–358.
- Huisman M, ter Haar G, Napoli A, et al. International consensus on use of focused ultrasound for painful bone metastases: Current status and future directions. Int J Hyperthermia. 2015;31:251–259.
- Cazzato RL, Koch G, Buy X, et al. Percutaneous image-guided screw fixation of bone lesions in cancer patients: double-centre analysis of outcomes including local evolution of the treated focus. Cardiovasc Intervent Radiol. 2016;39:1455–1463.
- Cazzato RL, Garnon J, Tsoumakidou G, et al. Percutaneous image-guided screws meditated osteosynthesis of impeding and pathological/insufficiency fractures of the femoral neck in non-surgical cancer patients. Eur J Radiol. 2017;90:1–5.
- Anselmetti GC, Manca A, Ortega C, et al. Treatment of extraspinal painful bone metastases with percutaneous cementoplasty: a prospective study of 50 patients. Cardiovasc Intervent Radiol. 2008;31:1165–1173.
- Tsoumakidou G, Too CW, Koch G, et al. CIRSE guidelines on percutaneous vertebral augmentation. Cardiovasc Intervent Radiol. 2017;40:331–342.
- Boyce-Fappiano D, Elibe E, Schultz L, et al. Analysis of the factors contributing to vertebral compression fractures after spine stereotactic radiosurgery. Int J Radiat Oncol Biol Phys. 2017;97:236–245.
- Jawad MS, Fahim DK, Gerszten PC, et al. on behalf of the Elekta Spine Radiosurgery Research Consortium. Vertebral compression fractures after stereotactic body radiation therapy: a large, multi-institutional, multinational evaluation. J Neurosurg Spine. 2016;24:928–936.
- Cazzato RL, Buy X, Eker O, et al. Percutaneous long bone cementoplasty of the limbs: experience with fifty-one non-surgical patients. Eur Radiol. 2014;24:3059–3068.
- Cazzato RL, Palussière J, Buy X, et al. Percutaneous long bone cementoplasty for palliation of malignant lesions of the limbs: a systematic review. Cardiovasc Intervent Radiol. 2015;38:1563–1572.