Abstract
Objective: To investigate factors affecting effects of ultrasound guided high intensity focused ultrasound (USgHIFU) in the treatment of single uterine fibroids (UFs) with different magnetic resonance imaging (MRI) features.
Methods: A total of 207 patients with single symptomatic UFs who were treated with HIFU were retrospectively analyzed. All UFs were grouped according to MRI features, and factors affecting HIFU ablation were set as independent variables. Non-perfusion volume ratio (NPVR) and energy efficiency factor (EEF) were set as dependent variables to establish multiple linear regression models with a stepwise method.
Results: All patients had successful HIFU treatment, with the mean NPVR of 74.7 ± 15.1% and the mean EEF of 7.4 ± 5.2 j/mm3. The NPVR was negatively correlated with transmural type of UFs, hyperintense on T2 weighted image (T2WI), enhancement type on T1 weighted image (T1WI), distance from UFs ventral side to skin and posterior location of UFs, but positively correlated with anterior location of UFs, hypointense on T2WI and anteverted uterus (uterine location). The EEF was negatively correlated with size, anterior location of UFs and hypointense on T2WI, but positively correlated with distance from UFs ventral side to skin, enhancement type on T1WI and transmural type of UFs. The UFs size and enhancement type on T1WI were the greatest factors affecting the ablation effect.
Conclusions: The effect of HIFU treatment for single UFs is affected by multiple factors, and the UFs of hypointense on T2WI, large size, mild enhancement on T1WI and anteverted uterus can be easily ablated with high ablation efficiency.
Introduction
As a common reproductive system disease in women of the childbearing age, uterine fibroids (UFs) often cause clinical symptoms such as menorrhagia, pain, increased abdominal pressure and fertility problems, greatly affecting women's health and quality of life. Although there are many therapeutic strategies for UFs, such as hysterectomy [Citation1,Citation2], myomectomy [Citation2], uterine artery embolization (UAE) [Citation3], and pharmacological therapy [Citation1], the demand for non-invasive treatment techniques is increasing with implementation of the two-children policy, improvement of living standards, and optimization of treatment measures in China. High-intensity focused ultrasound (HIFU), as a new technology for local physiotherapy, has been widely used in non-invasive ablation of UFs and has proved to be extremely safe and effective [Citation4–6]. However, according to previous clinical experience and research reports, HIFU ablation of UFs is affected by factors such as internal structure (blood supply, water content and fiber composition), location, type and size of UFs [Citation7,Citation8]. As predictors for the effect of HIFU treatment and symptom relief, these factors play an important role in preoperative evaluation and planning for patients with UFs.
For evaluation of HIFU ablation, the non-perfusion volume ratio (NPVR) is defined as the percentage of non-perfusion volume (NPV) in the UFs volume on postoperative magnetic resonance imaging (MRI). The NPVR is related to symptom relief and can be used to represent the HIFU ablation rate [Citation7].Therefore, the therapeutic goal of HIFU should be to achieve the maximal NPVR as much as possible without increasing complications and side effects, so as to achieve the maximal symptom relief and best prognosis for the patients. The energy efficiency factor (EEF) represents ultrasonic energy required for UFs ablation per unit volume (1mm3). As one of the most accurate quantitative indicators of HIFU ablation, EEF reflects the energy deposition efficiency of HIFU. The smaller the EEF, the less energy needed to ablate a certain volume of UFs tissue and the higher the HIFU ablation efficiency. NPVR and EEF have important values in evaluating the effect of HIFU ablation. Multiple UFs may affect treatment outcomes and statistical results because the ultrasound energy may be absorbed by other fibroids along the acoustic path during HIFU treatment, and consequently, we analyzed in this study factors affecting ablation effects for single UFs, which might help selecting suitable patients, evaluating the treatment difficulty before HIFU and guiding the HIFU treatment.
Materials and methods
Patients
A retrospective analysis was performed of 207 patients with single symptomatic UFs who were treated with HIFU in the First Affiliated Hospital of Kunming Medical University from September 2013 to September 2017. The inclusion criteria for the patients were: (1) the diagnosis of UFs was confirmed by ultrasound and MRI, (2) patients were not currently pregnant, (3) patients were between 18 and 55 years of age, and (4) patients could communicate with doctors. The exclusion criteria were: (1) UFs had signs of calcification, (2) suspected malignant changes in UFs, and (3) surgical scars were found in the lower abdomen. This study was approved by the ethics committee of our hospital with all patients given their written informed consent. Preoperative clinical examinations were conducted, including transvaginal ultrasonography, liver and renal function test, chest radiography, thinprep cytologic test (TCT), human chorionic gonadotropin (HCG), and CAl25. Plain and enhanced MRI was performed preoperatively with a standardized protocol, and the scan sequence included T1- weighted image (T1WI), T2- weighted image (T2WI), and contrast- enhanced T1WI. The size of UFs, distance from UFs ventral side to skin and dorsal side to sacrum was measured by two experienced radiologists, and the volume of UFs (V0) was calculated. The volume of a fibroid or the non-perfused volume of a fibroid on contrast- enhanced MRI was calculated by the ellipsoid formula: length × width × height ×0.5233 [Citation7,Citation8]. According to the characteristics of UFs on T1WI MRI images, patients were grouped into mild, moderate or significant enhancement when the enhancement was lower than, equal to or higher than, respectively, the normal myometrium. The imaging enhancement of UFs could also be divided into uniform and non-uniform enhancement. Compared to the myometrium and skeletal muscle, the signal intensity (SI) on T2WI was classified into hypointense when the SI was similar to that of the normal skeletal muscle, isointense when higher than that of the skeletal muscle but lower than that of myometrium, hyperintense when similar to or higher than that of myometrium, and mixed SI when more than two SI types existed [Citation9]. The location of UFs was divided into anterior, lateral, posterior and fundus, the type of UFs into intramural, subserosa, submucosal and transmural (Intramural fibroids originate in the central myometrium but don’t extend to the endometrium or serosa; transmural fibroids extend through the entire uterine wall, from the endometrial lining to serosa) [Citation10], and the uterine location into anteverted, midposition and retroverted [Citation11].
Equipment
Chongqing JC200 focused ultrasound tumor treatment system (frequency, 0.8 MHz; treatment power, 200–400 W; physical focal field, 3 mm ×3 mm ×8 mm) was used for the ablation of UFs. A B-mode ultrasound (Esaote MyLab70, Genoa, Italy) was used to monitor HIFU treatment. MRI examinations were performed with an Achieva 3.0 T MR system (Philips, Best, Netherlands) or Signa HDxt 3.0 T MR system (GE, Waukesha, USA). Gadolinium-diethylenetetramine pentaacetic acid (GD-DTPA) as a kind of MRI contrast agent was used for contrast-enhanced TIWI. The same sequence and parameters of MRI scan were used for scanning of the patients before and after HIFU.
US-guided HIFU procedure
All patients had a definitive diagnosis of UFs and no contraindications to HIFU treatment. Twelve hours before HIFU, polyethylene glycol was used for catharsis; on the day of treatment, the patients fasted for 12 h, and normal saline (NS) of 500–l000 ml were used for enema. The patients were laid in the prone position on the HIFU treatment bed, with the abdominal wall in contact with degassed water. Based on the patient's weight, fentanyl and midazolam were used for intraoperative sedation and analgesia. When significant grayscale changes were observed or sufficient therapeutic dose was achieved, the focal point was moved to the next location for ablation. During the treatment, the patient's heart rate, respiration, blood pressure, oxygen saturation and other vital signs were monitored, and patients were asked to promptly report of any discomfort. The treatment parameters were recorded, including sonication time (t), treatment time (T), treatment power (P), therapeutic dose (TD), adverse reaction (AE) type and frequency. Antibiotics were routinely used to prevent postoperative infection, and MRI was performed one day after HIFU to observe and evaluate the UFs. At the same time, the three-dimensional diameter of non-perfusion area was measured and the NPV (V1) was calculated. Preoperative UFs volume (V0) and postoperative NPV (V1) were used to calculate NPVR (NPVR = V1/V0 × 100%). EEF was calculated based on treatment parameters and NPV (V1) (EEF = η P t/V1, η = 0.7).
Statistical analysis
The software SPSS 17.0 (IBM, Armonk, NY, USA) was used for all statistical analysis. Normally distributed data were recorded as mean ± standard deviations (SD), and skewed distribution data were expressed as median and interquartile range. Dummy variables were defined respectively because of the introduction of polytomous variables (uterine location, location of UFs, type of UFs, SI on T2WI). NPVR and EEF were set as dependent variables, and all possible factors (each dummy variable, the distance from the UFs dorsal side to the sacrum, the distance from the UFs ventral side to skin, age, volume of UFs, size of UFs, enhancement type on T1WI, enhancement uniformity on T1WI) were set as independent variables. A stepwise regression method was used to establish multiple linear regression models.
In this study, the correlation between predictors and dependent variables was analyzed, and a p values <.05 was considered statistically significant. Considered to be linearly related to the dependent variables, these statistically significant predictors were included in the linear regression model one by one, with the criteria of 0.05 and 0.1 for introducing and eliminating, respectively. As one of the indicators of multiple correlation analysis, the multiple correlation coefficient (R) was used to illustrate the closeness of the linear relationship between the dependent and multiple independent variables. The coefficient of determination (R2, 0 ≤ R2 ≤ 1) was used to show the proportion of the total variation in the dependent variable that can be explained by multiple independent variables in the regression model. As one of the important indicators to measure the quality of the model, adjusted determination coefficient (adjusted R2) took into account the influence of independent variables number in the equation, and the best-fit model had the largest adjusted R2. Analysis of variance (ANOVA) was performed on all models, and a p values of less than .05 indicated statistically significant. In addition, t-test and collinearity diagnosis were also performed on all models, and only the results of best-fit model were shown in the coefficient table. Collinearity means that the independent variables are non-independent or linearly related, so that one or more independent variables can be linearly represented by another independent variable. Many serious problems may arise when collinearity is strong, such as the leakage of important variables in the equation, regression coefficient instability and equation instability. In this study, the diagnosis of collinearity is mainly based on the tolerance, variance inflation factor (VIF), eigenvalue and condition index, and serious collinearity is considered to exist when the tolerance is less than 0.1 or VIF is greater than 10 or eigenvalue tends to be 0 or the condition index is greater than 30.
Results
Baseline patients characteristics
A total of 207 patients with UFs were enrolled, with a mean age of 37.7 ± 6.3 years (range 24–53 years) (). Pretreatment median volume of UFs, mean distance from ventral side to skin and from the dorsal side to the sacrum were 65189.1 mm3, 52.9 ± 22.2 mm and 28.1 ± 15.6 mm, respectively. In this study, the UFs were located in the anterior wall (n = 67), lateral wall (n = 58), posterior wall (n = 58), and fundus (n = 24); fibroid types included subserosal fibroids (n = 43), submucosal fibroids (n = 46), intramural fibroids (n = 87), and transmural fibroids (n = 31). Signal characteristics were hypointense (n = 52), isointense (n = 68), hyperintense (n = 46) and mixed signal (n = 41); degree of enhancement was mild enhancement (n = 45), moderate or significant enhancement (n = 162) on T1WI. The uterus positions in those patients were anteverted uterus (n = 98), midposition uterus (n = 65) and retroverted uterus (n = 44).
Table 1. Patients baseline characteristics and treatment data.
Treatment outcome
All patients successfully completed the HIFU treatment with a success rate of 100%. The treatment time, which was defined as the time from the first sonication to the last sonication, was 127.7 ± 59.8 min (range 15.0 – 270.0 min). Preoperative contrast-enhanced MRI showed different degrees of enhancement of UFs, and postoperative contrast-enhanced MRI showed perfusion defects in the effective ablation area ().
Figure 1. Contrast enhanced magnetic resonance imaging (CE-MRI) before and after treatment: (A1) anterior wall and mildly enhanced uterine fibroids; (B1) lateral wall and moderately enhanced uterine fibroids; (C1) posterior wall and significantly enhanced uterine fibroids; CE-MRI after treatment shows that the ablation area is not enhanced (A2, B2 and C2).
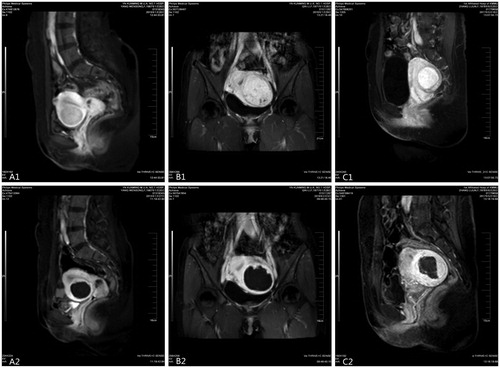
Relationship between affecting factors and NPVR
Twelve statistically significant predictors (p < .05) were identified including age, distance from UFs ventral side to skin, distance from UFs dorsal side to the sacrum, anteverted uterine location, anterior and posterior location of UFs, intramural, subserosal, and transmural type of UFs, hypointense and hyperintense SI on T2WI, enhancement type and uniformity on T1WI. These 12 statistically significant predictors were included into the linear regression model or eliminated one by one according to the inspection levels of 0.05 and 0.1. A total of eight statistically significant predictors were included into the linear regression model: transmural type, anterior location, hyperintense SI on T2WI, enhancement type on T1WI, distance from UFs ventral side to skin, hypointense SI on T2WI, posterior location and anteverted uterine position (). Among the total variation of the dependent variable of NPVR, 42% could be explained by these 8 predictors (R2 = 0.420) (). With the greatest value of adjusted R2 (0.397), model 8 was considered to be the best-fitting model. All models were statistically significant (p < .001) (). According to , the multiple linear regression equation is ŷ = 0.904 – 0.136 X1 + 0.071 X2 − 0.071 X3 − 0.063 X4 − 0.001 X5 + 0.050 X6 − 0.043 X7 + 0.034 X8, where variable ŷ = NPVR; X1 = transmural; X2 = anterior; X3 = hyperintense; X4 = enhancement type on T1WI; X5 = distance from UFs ventral side to skin; X6 = hypointense; X7 = posterior and X8 = anteverted uterus. Therefore, NPVR was negatively correlated with posterior location of UFs, transmural type of UFs, hyperintense SI on T2WI, enhancement type on T1WI and distance from UFs ventral side to skin, but positively correlated with anterior location, hypointense SI and anteverted uterine position. The collinearity analysis showed no collinearity in the eight predictors with a well-matched model ().
Table 2. The multivariable regression model and analysis of varianceTable Footnotei.
Table 3. Coefficient of multivariable regression modelTable Footnotea of NPVR.
Relationship between the affecting factor and EEF
When EEF was set as a dependent variable, a total of 11 predictors had linear correlation with EEF, including distance from UFs ventral side to skin, anteverted uterine position, posterior location of UFs, enhancement uniformity on T1WI, intramural, subserosal and transmural type of UFs, size and volume of UFs, hypointense SI on T2WI and enhancement type on T1WI. These 11 predictors were included one by one into the linear regression model when a p values was less than or equal to .05 and eliminated when a p values was greater than or equal to .1. In the stepwise regression process, the first variable introduced was distance from UFs ventral side to skin, followed by enhancement type on T1WI. As a result, six independent variables were introduced into a multiple linear regression model (). The R, R2 and adjust R2 of Model 6 was 0.610, 0.373 and 0.354, respectively, making it the best-fit model. The results of the ANOVA for all models showed that they were statistically significant (p < .001) (). According to the regression equation table (), the multiple linear regression equation is ŷ = 5.354 + 0.049 X1 + 3.192 X2 − 0.101 X3 + 3.160 X4 − 1.483 X5 −1.689 X6 (ŷ = EEF, X1 = distance from UFs ventral side to skin, X2 = enhancement type on T1WI, X3 = size, X4 = transmural, X5 = anteverted uterus, and X6 = hypointensity). EEF had a negative correlation with size of UFs, anteverted uterine position and hypointensity SI on T2WI, but a positive correlation with distance from UFs ventral side to skin, enhancement type on T1WI and transmural type of UFs. Therefore, a higher HIFU ablation efficiency was easier to achieve in UFs with a large size, anteverted uterus and hypointensity SI on T2WI compared with the other groups. In the standard partial regression coefficients of model 6 with the largest positive value (0.254), T1 enhancement type was considered the greatest positive correlation factor for EEF whereas predictor size of UFs had the greatest negative value (−0.298). The larger the size of UFs, the smaller the EEF. According to the value of tolerance, VIF, eigenvalue and condition index, no collinearity was found in the 6 predictors and the model matched well (). The other independent variables had no significant (p > .05) relation with the EEF.
Table 4. The multivariable regression model and analysis of varianceTable Footnoteg.
Table 5. Coefficient of multivariable regression modelTable Footnotea of EEF.
Discussion
In the past ten years, HIFU has been widely used for ablation of solid tumors in China, especially in non-invasive treatment of UFs. HIFU can converge low-energy ultrasonic beams in the focal point to agitate tissue ions and cause ion friction, producing thermal effects (60–100 °C) and cavitation effects to destroy tumor parenchymal cells and vascular endothelial cells and resulting in coagulative necrosis to fibroid tissue [Citation4]. As a non-invasive, targeted, safe, non-radioactive and reproducible local physical therapy, HIFU can eliminate or reduce the physical symptoms caused by UFs while retaining genital organs and their functions [Citation12–14].
However, significant differences existed in the efficacy of HIFU ablation for UFs with different MRI characteristics. According to previous studies [Citation7,Citation8], since the ultrasonic wave underwent energy decay due to refraction, reflection, absorption and scattering in non-target tissue in the penetrating route, required dose of ultrasonic wave for ablation treatment was more likely reached for UFs located in the anterior wall or with a short distance from UFs ventral side to skin. During the ablation treatment, the fibroids with large back-field distance are more suitable for ablation in order to avoid secondary damage to the sacrum. What’s more, as the most common type of UFs in our study (42%), intramural UFs have better ablation effects probably because of fewer penetrated tissue layers and poor blood supply compared with transmural and submucosal UFs. In addition, T1WI contrast-enhanced scanning can reflect the blood perfusion of UFs, and flowing blood can absorb HIFU energy and may result in low energy deposition efficiency and high EEF. For T2WI hypointensity UFs, HIFU energy is more easily deposited because of great acoustic impedance caused by poor water content and rich fiber components. Therefore, these patients are more suitable for HIFU ablation [Citation9,Citation15]. For UFs with large sizes, HIFU treatment is easier to perform because of the acoustic environment of focal tissue that is dynamically affected by rise of focal temperature and expansion of necrotic areas of ablation, and these UFs may have smaller EEF compared with smaller UFs [Citation7,Citation16].
In order to exclude the impact of multiple UFs on the statistical results, we selected single UFs as the study sample to analyze all factors that may affect the ablation effect. A total of 207 patients with single UFs who underwent USgHIFU in our hospital met the inclusion criteria. What’s more, the established equation is very unstable when the sample size is not large enough relative to the number of independent variables, and the generally appropriate sample size should exceed 5–10 times the number of independent variables in the equation. The results of collinearity diagnostics also showed that the models matched well, and the sample size of this study met the statistical requirements. The UFs shrinkage rate and symptom relief are associated with the extent of ablation, and the higher the NPVR, the smaller the volume of the UFs after HIFU [Citation4,Citation17,Citation18]. As an accurate quantitative index of dose delivery, EEF can directly reflect the relationship between dosage and the efficiency of HIFU ablation [Citation8]. Therefore, both NPVR and EEF were selected as dependent variables to establish the multiple linear regression models. According to the results of multi-factor analysis, anterior location of UFs, hypointensity SI on T2WI and anteverted uterine position were positively correlated with NPVR, whereas transmural type of UFs, hyperintensity SI on T2WI, enhancement type on T1WI, distance from UFs ventral side to skin and posterior location of UFs were negatively correlated with NPVR. As described above, the primary reason for those results is likely related to energy attenuation during transmission of the ultrasonic wave and a cooling effect due to blood flow during ablation treatment. These UFs patients with higher NPVR suitable for HIFU ablation usually have UFs located in the anteverted uterus, anterior wall, hypointensity on T2WI, mild enhancement type on T1WI and short distance from UFs ventral side to skin, and the physical symptoms associated with the UFs are easier to eliminate. Moreover, the EEF was negatively correlated with size of UFs, anterior location of UFs and hypointensity SI on T2WI, but positively correlated with distance from UFs ventral side to skin, enhancement type on T1WI and transmural type of UFs. Therefore, UFs with a long distance from UFs ventral side to skin, retroverted uterine position, significant enhancement on T1WI, hyperintensity SI on T2WI, small size, transmural type and posterior location are harder to ablate, which is consistent with previous studies [Citation7,Citation8,Citation11].
The internationally more prevalent technique magnetic resonance-guided high-intensity focused ultrasound (MRgFUS) can provide accurate anatomical details and real-time temperature measurement to effectively control energy delivery and ablation range, making the ablation process more assured[Citation17,Citation19]. One study conducted by Kim et al. [Citation20] demonstrated that the ablation efficiency and ablation quality of MRgFUS were negatively correlated with subcutaneous fat thickness, relative peak enhancement and T2 signal intensity ratio, and our research is in line with it. What’s more, the study by Keserci and Duc [Citation21] showed that NPVR is very strongly correlated with T1 perfusion based classification and that blood perfusion may be a major factor affecting temperature rise in MRgFUS. However, the results of our study are more comprehensive due to consideration of more affecting factors. The affecting factors discussed in this study may also be applied to MRgFUS, and further large-scale comparative studies of MRgFUS and USgHIFU are expected to address this issue.
Our study has some limitations. First, as one of the possible affecting factors, the patient's BMI has not been recorded and analyzed, which may affect the result of HIFU treatment. Second, our study was a retrospective analysis of a small sample size with the possibility of selection bias. Therefore, further studies with larger sample sizes and optimization methods are needed to explore this issue.
Conclusions
In conclusion, HIFU treatment of single UFs is affected by multiple factors, and UFs of hypointense SI on T2WI, large size, mild enhancement type on T1WI and anteverted uterus are easier to ablate with high ablation efficiency.
Disclosure statement
No potential conflict of interest was reported by the authors.
References
- Olejek A, Olszak-Wąsik K, Czerwinska-Bednarska A. Long-term intermittent pharmacological therapy of uterine fibroids - a possibility to avoid hysterectomy and its negative consequences. 2016;1:48–51.
- Taniguchi F, Koike N, Kikukawa T, et al. An evaluation of laparoscopic hysterectomy alone versus in combination with laparoscopic myomectomy for patients with uterine fibroids. Eur J Obstet Gynecol Reprod Biol. 2017;210:132–138.
- Froeling V, Meckelburg K, Schreiter NF, et al. Outcome of uterine artery embolization versus MR-guided high-intensity focused ultrasound treatment for uterine fibroids: long-term results. Eur J Radiol. 2013;82:2265–2269.
- Zhao WP, Han ZY, Zhang J, et al. A retrospective comparison of microwave ablation and high intensity focused ultrasound for treating symptomatic uterine fibroids. Eur J Radiol. 2015;84:413–417.
- Cho JY, Kim SH, Kim SY, et al. Efficacy and safety of daily repeated sonographically guided high-intensity focused ultrasound treatment of uterine fibroids: preliminary study. J Ultrasound Med. 2013;32:397–406.
- Zhang C, Jacobson H, Ngobese ZE, et al. Efficacy and safety of ultrasound-guided high intensity focused ultrasound ablation of symptomatic uterine fibroids in Black women: a preliminary study. BJOG. 2017;124 (Suppl 3): 12–17.
- Gong C, Yang B, Shi Y, et al. Factors influencing the ablative efficiency of high intensity focused ultrasound (HIFU) treatment for adenomyosis: a retrospective study. Int J Hyperthermia. 2016;32:496–503.
- Peng S, Zhang L, Hu L, et al. Factors influencing the dosimetry for high-intensity focused ultrasound ablation of uterine fibroids: a retrospective study. Medicine (Baltimore). 2015;94:e650.
- Funaki K, Fukunishi H, Funaki T, et al. Magnetic resonance-guided focused ultrasound surgery for uterine fibroids: relationship between the therapeutic effects and signal intensity of preexisting T2-weighted magnetic resonance images. Am J Obstet Gynecol. 2007;196:184.e1–1e6.
- Lacayo EA, Richman DL, Acord MR, et al. Leiomyoma Infarction after Uterine Artery Embolization: Influence of EmbolicAgent and Leiomyoma Size and Location on Outcome. J Vasc Interv Radiol. 2017;28:1003–1010.
- Zhang W, He M, Huang G, et al. A comparison of ultrasound-guided high intensity focused ultrasound for the treatment of uterine fibroids in patients with an anteverted uterus and a retroverted uterus. Int J Hyperthermia. 2016;32:623–629.
- Yang Z, Zhang Y, Zhang R, et al. A case-control study of high-intensity focused ultrasound combined with sonographically guided intratumoral ethanol injection in the treatment of uterine fibroids. J Ultrasound Med. 2014;33:657–665.
- Lee JS, Hong GY, Lee KH, et al. Changes in anti-müllerian hormone levels as a biomarker for ovarian reserve after ultrasound-guided high-intensity focused ultrasound treatment of adenomyosis and uterine fibroid. BJOG: Int J Obstet Gy. 2017;124:18–22.
- Cheung VY, Lam TP, Jenkins CR, et al. Ovarian reserve after ultrasound-guided high-intensity focused ultrasound for uterine fibroids: preliminary experience. J Obstet Gynaecol Can. 2016;38:357–361.
- Zhao WP, Chen JY, Zhang L, et al. Feasibility of ultrasound-guided high intensity focused ultrasound ablating uterine fibroids with hyperintense on T2-weighted MR imaging. Eur J Radiol. 2013;82:e43–e49.
- Chen L, ter Haar G, Hill CR. Influence of ablated tissue on the formation of high-intensity focused ultrasound lesions. Ultrasound Med Biol. 1997;23:921–931.
- Thiburce AC, Frulio N, Hocquelet A, et al. Magnetic resonance-guided high-intensity focused ultrasound for uterine fibroids: Mid-term outcomes of 36 patients treated with the Sonalleve system. Int J Hyperthermia. 2015;31:764–770.
- Himabindu Y, Sriharibabu M, Nyapathy V, et al. Early evaluation of magnetic resonance imaging guided focused ultrasound sonication in the treatment of uterine fibroids. Indian J Med Res. 2014;139:267–272.
- Babashov V, Palimaka S, Blackhouse G, et al. Magnetic resonance-guided high-intensity focused ultrasound (MRgHIFU) for treatment of symptomatic uterine fibroids: an economic analysis. Ont Health Technol Assess Ser. 2015;15:1–61.
- Kim YS, Lim HK, Park MJ, et al. Screening magnetic resonance imaging–based prediction model for assessing immediate therapeutic response to magnetic resonance imaging–guided high-intensity focused ultrasound ablation of uterine fibroids. Invest Radiol. 2016;51:15–24.
- Keserci B, Duc NM. The role of T1 perfusion-based classification in predicting the outcome of magnetic resonance-guided high-intensity focused ultrasound treatment of adenomyosis. Int J Hyperthermia. 2017;19:1–9.