Abstract
Objective: To assess the clinical efficiency and feasibility of ultrasound-guided percutaneous microwave ablation (US-PMWA) assisted by a three-dimensional (3D) visualization platform for treating a single large hepatocellular carcinoma (HCC) (diameter ≥5 cm).
Methods: We included 40 patients with a single large HCC from May 2015 to August 2017. Nineteen patients who underwent US-PMWA assisted by a 3D visualization platform formed the 3D group; 21 patients without 3D visualization assistance formed the control group. Preoperative planning and post-procedural evaluation were performed successfully with the 3D visualization treatment platform. Thermal ablative parameters, survival and recurrence were compared between groups.
Results: The primary efficacy rate and recurrence-free survival (RFS) rate in the 3D group were higher than those in the control group (p = .007 and p = .014, respectively). The number of inserted probes in the 3D group was higher than that in the control group (p = .016). The overall survival (OS) rate in the 3D group was comparable to that of controls (p = .745). Estimated 3-year OS and RFS rates were 38.5% and 64.6% in the 3D group and 34.4% and 18.8% in the control group, respectively. Treatment effects were evaluated using a tumor map and confirmed by traditional contrast-enhanced ultrasound in the 3D group. After the first treatment, nine patients (47.4%) achieved complete ablation with a 5-mm safety margin. No major complications related to ablation occurred.
Conclusions: US-PMWA assisted by a 3D visualization treatment platform can improve treatment efficiency and appears to be a safe, effective and innovative technique to manage a single large HCC.
Introduction
Hepatocellular carcinoma (HCC) is one of the most common malignancies worldwide, with an incidence of 28.71/100 000 in China [Citation1–3]. HCC has a dismal prognosis, with exceptionally high mortality rates. At present, surgical resection and hepatic transplantation are the first-line curative treatment options for HCC. Unfortunately, most HCC patients do not have the opportunity for surgery or transplantation due to poor liver function, advanced tumor progression or scarce organ resources. Over the past decade, alternative interventional treatments have rapidly developed and have been applied in the treatment of different tumors [Citation4,Citation5]. Among them, local thermal ablative techniques, including microwave ablation (MWA) and radiofrequency ablation (RFA), have been confirmed as safe and effective for the treatment of patients with HCC in very early stages, based on the Barcelona Clinic Liver Cancer (BCLC) staging system [Citation6]. Meanwhile, as another local minimally invasive therapy, transarterial chemoembolization (TACE) has become one of the most widely used treatment modalities for patients with hypervascular BCLC stage B HCC [Citation7]. All of the aforementioned interventional treatments have distinctive therapeutic characteristics for unresectable HCC, which include high efficiency, minimal invasiveness and relatively short treatment duration [Citation8,Citation9].
Although thermal ablation can achieve satisfactory clinical results for smaller HCC, it is difficult to achieve complete ablation with relatively large HCC. Many patients with HCC are diagnosed with very advanced stages of disease and tumor size greater than 5 cm, owing to patients’ ignorance of the importance of routine physical examinations [Citation10]. For these patients, the overall survival (OS) rate and tumor recurrence rate remain very high owing to the inability to achieve a 5-mm ablative margin and the uneven distribution of heat [Citation11]. To solve these problems, in recent years, strategies for the treatment of large tumors, such as repeated thermal ablation, surgical elimination after RFA and combined interventional therapies (e.g., thermal ablation and TACE) [Citation12,Citation13] have been developed. These approaches can achieve better survival results than a single thermal ablative method [Citation14,Citation15].
It is important to understand the spatial relationship between the tumor and surrounding tissues, especially for thermal ablation of larger HCC. Reasonable arrangement of needles and the achievement of an ablative margin are important to the patient’s prognosis. Previous reports suggest that the ablative margin is closely related to local tumor progression (LTP) [Citation16]. However, conventional thermal ablative methods do not quantify the ablation range, and the approach of the puncture path is dependent on the subjective judgment and clinical experience of the radiologist, which may result in residual tumor after MWA or failure to obtain the ablative margin. In addition, treatment effects can only be judged by traditional imaging methods, such as side-by-side computed tomography (CT)/magnetic resonance imaging (MRI) images, which can easily result in omission of the residual tumor [Citation17–19]. In this study, a three-dimensional (3D) visualization treatment platform was used to improve the precision of interventional treatment [Citation20]. This platform has been found to be useful in developing a clear preoperative plan and for post-procedural quantitative evaluation after thermal ablation.
Therefore, the aim of this retrospective study was to assess the clinical utility of 3D visualization treatment platforms in improving ultrasound-guided percutaneous microwave ablation (US-PMWA), pre-procedural planning and post-procedural assessment of treatment response, when treating a single large HCC.
Materials and methods
Patients and tumors
The study protocol was approved by the Ethics Committee of the Chinese PLA General Hospital (Beijing, China) and was conducted in accordance with the principles of the Declaration of Helsinki. A detailed verbal and written description of the procedure was provided to all patients and informed written consent was obtained before treatment. The medical records of all patients with a single large primary HCC (diameter ≥5 cm) who underwent US-PMWA between May 2015 and August 2017 were retrospectively reviewed. All patients with HCC were discussed at multidisciplinary meetings that included interventional radiologists (L.P. and Y.X.L., each with 25 years of experience), oncologists, hepatologists and pathologists to determine treatment methods, which included surgical resection, liver transplantation, local thermal ablation, TACE and systemic therapies. The inclusion criteria for this study were (1) non-resectable tumor (i.e., insufficient liver remnant, high risk for complications of resection associated with old age or tumor location) or patient refusal to undergo surgery; (2) clear visualization of the entire tumor on ultrasound imaging; (3) maximum tumor diameter of ≥5 cm and <10 cm; (4) post-intervention MRI performed not more than 1 week after MWA; (5) prothrombin time of <25 s; (6) prothrombin activity of >40%; and (7) platelet count of >50 × 109/L. The exclusion criteria for this study were (1) Child–Pugh class C; (2) severe comorbidity including renal failure or cardiopulmonary disease; and (3) severe ascites. The patient screening process is summarized in .
Figure 1. Flow diagram shows study patient accrual process. US-PMWA: ultrasound-guided percutaneous microwave ablation; HCC: hepatocellular carcinoma; TACE: transcatheter arterial chemoembolization.
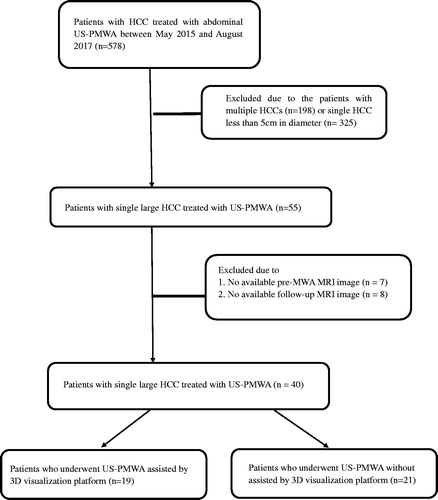
During the study period, 19 patients (average age, 58.6 ± 10.2 years; range, 41–79 years), with a single large HCC underwent US-PMWA assisted by the three-dimensional (3D) visualization platform. Among them, 18 patients were male and one was female. The average HCC maximum diameter was 6.1 ± 1.3 cm (range, 5–8.5 cm). A total 21 patients (19 males and 2 females; average age, 61.4 ± 8.4 years; range, 43–78 years), who underwent US-PMWA without 3D visualization assistance were selected as the control group. Among controls, the average HCC maximum diameter was 5.9 ± 1.6 cm (range, 5–7.7 cm). Thirty-one (77.5%) patients who underwent TACE before MWA had a median interval between the two types of treatment of 5.4 months (range, 2.1–8.5 months). A total of 14 patients received TACE before US-PMWA, with a median interval time of 4.8 months (range, 2.1–7.2 months) in the 3D group. A total of 17 patients in the control group received TACE before MWA, with a median interval time of 5.8 months (range, 4.3–8.5 months).
The diagnosis was established before treatment according to (1) hepatitis history, (2) laboratory testing and/or (3) contrast-enhanced ultrasound (CEUS) and dynamic MRI. The final diagnosis was confirmed by histological analysis of needle biopsy specimens.
3D visualization preoperative planning
A desktop computer (Lenovo PC International, Beijing, China) with an Intel Core i5 processor originally obtained for an empirical study in our department was used to perform 3D visualization preoperative planning. MRI data (2.5-mm-thick slices) of HCC before MWA were converted to DICOM format and then imported into the 3D visualization treatment platform (Hokai Medical Instruments Co., Ltd., Zhuhai, China). The current commercially available 3D visualization tools are described in . In this study, the 3D visualization treatment platform included the following novel functions: (1) rapid segmentation of the target (within 2 min), (2) target volume calculation, (3) simulation of the thermal field, (4) a manually operated interactive pre-ablative planning strategy, and (5) generation of a tumor map to evaluate the ablation effect.
Table 1. 3D visualization soft summary.
HCC was detected by MRI in the arterial phase, and the region of interest (ROI) of each tumor was outlined in red; areas to be deleted around the ROI were outlined in blue (). 3D images were created in three orthogonal views (axial, coronal and sagittal) and processed for image translation and rotation. The liver, tumor and surrounding structures can be displayed in stereo using the 3D visualization treatment platform, with automatic generation of the 3D volumes (). Through interactive manual operation, the number, embedding path and angles of the antennas and thermal field scope can be simulated to improve interventional therapy accuracy (). One week after the operation, the thermal ablation field was segmented and displayed in stereo using the 3D visualization treatment platform ().
Figure 2. Screenshots show procedure for automatic generation of the 3D volume in the 3D visualization treatment platform and preoperative plan. (A) A HCC tumor was shown in the MRI axial image and we sketched a region of interest (ROI) in the tumor and delete areas around ROI. (B) The tumor was shown in 3D style. (C) Pre-operation 3D visualization treatment platform showed the location of the tumor and the close relationship with the surrounding structures, and six antennas were needed to ablate the tumor completely and safely. (D) The ablative area was shown in 3D style.
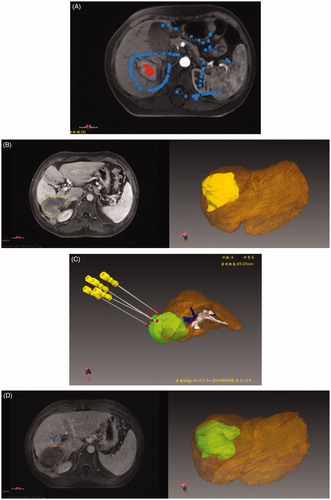
TACE for large HCC
TACE was performed by two interventional radiologists in the interventional radiography department, each with 10 years’ experience. Hepatic angiography was performed using a 4-F guiding catheter with a right common femoral approach, to map the vascular anatomy of the liver, arterial tumor supply, and eventual arteriovenous shunts. Lipiodol and chemotherapeutic drugs including oxaliplatin combined with fluoropyrimidine were advanced into the segmental hepatic artery feeding the lesion. When the target lesion was contracted and the lipiodol was well deposited in the contrast-enhanced computed tomography image, MWA treatment was begun.
US-PMWA for treatment of large HCC
MWA was performed by three interventional radiologists (P.L., 20 years’ experience; X.L.Y., 20 years’ experience; and J.Y., 10 years’ experience in MWA). The microwave system (KY-2000; Kangyou Medical Instrument Co. Ltd., Nanjing, China) was composed of a flexible coaxial cable, an MW generator and cooled-shaft antenna. Power at 1–100 W and 2450 MHz is simultaneously produced from the two antennas by the generator. For each patient, local anesthesia of 1% lidocaine (Yiyou Shengshi (Beijing) Technology Co., Ltd., Beijing, China) was administered and a 2-cm skin incision was made with a scalpel. An automatic biopsy gun fitted with an 18 G cutting needle was used for US-guided biopsy before ablation. Consequently, two 15 G antennas were simultaneously inserted into the large tumor with US guidance, to percutaneously target the mass in accordance with the preoperative plan. Multisite ablation was performed with a power range of 50–60 W. The antenna was then repeatedly inserted according to preoperative planning until the ablation zone covered the entire tumor area, with an ablative margin of >0.5 cm. The high electromagnetic energy generated from the power source was allowed to penetrate 2 mm into the tumor tissue, which represents the active microwave heating zone. From this zone, the microwaves rotate causing the water molecules to heat rapidly, which ultimately coagulates the tumor tissue owing to protein denaturation. When the hyperecho overlapped the entire lesion, the antennas were withdrawn.
The following ancillary procedures were enacted to avoid non target ablative injury: (1) a hydrodissection procedure was applied under US guidance, with 0.9% saline injected into thoracic or abdominal cavity to separate the liver from other vital organs; and (2) a temperature probe was inserted to measure the ablation temperature in real time.
Post-procedural evaluation of 3D visualization image fusion
3D visualization image registration was also used with MRI data (2.5-mm-thick slices) before and after MWA. The obtained images were segmented using 3D visualization software that was based on the Medical Imaging Interaction Toolkit. The segmentation procedure of the ablative areas in the delayed phase was similar to that described above. The registration process was initialized by automatic position matching of the center of gravity of the lesions with their nonlinear volumes and was based on appropriate landmarks, such as branching vessels. To achieve more exact results, the registered images were manually adjusted. Next, the post-interventional image of the ROI was shown as a colored overlay on the pre-interventional image; it could also be moved using a computer mouse to improve the correlation between both images. Finally, the resulting registration matrix was applied to the coagulation mask. shows the actual segmentation and registration processes of a representative case.
Figure 3. A 53-years male with single HCC (8.4 × 6.2 × 6.4 cm) Screenshots shows procedure for three-dimensional visualization imaging fusion and tumor map generation. (A) The junction between the portal vein and the hepatic fissure as an appropriate landmark was selected (circle cross indicate). (B) During the procedure of -dimensional visualization imaging fusion, a part of the tumor appear outside the ablation area . (C) Tumor map showed the proportion of various parts of the tumor in the ablation area. (D) According to 3D fused image and tumor map, a new preoperative planning is performed again.
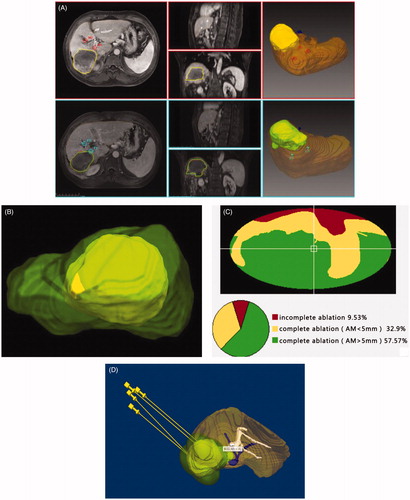
In this study, the 3D surface of the tumor was transferred onto a 2 D map to reduce the high amount of interactions. Aspherical parameterization of the surface was used and subsequently flattened onto a 2D map. For the ablative effect to be assessed, 3D coagulation zones over the spatial extent of the tumor were identified (), and a traffic light color scheme was used to emphasize the ablation state (), as follows. (1) The zone of complete cell destruction was marked with green. This zone is the optimal ablation result inside the intentional safety margin (>5 mm). (2) The zone of cell destruction outside of the safety margin (>5 mm) was marked with yellow. This zone is critical because the safety margin is not sufficiently large, leading to potential cancer recurrence. (3) The zone of tumor cells that could not be ablated is the worst case and was marked with red, an established warning color in medical applications. In this zone, the ablation strategy has failed and reablation of the tumor is probably unavoidable. If there was residual tumor, additional ablation was arranged within 7 days, to permit restoration of liver function. If there was no residual tumor, treatment was considered to be completed successfully. The next US-PMWA was again performed with 3D visualization preoperative planning, according to 3D images based on registration between pre-interventional and post-interventional images ().
Follow-up
The follow-up included routine physical examinations, laboratory tests (blood count and total bilirubin, serum albumin, prothrombin time, and tumor marker levels), and CEUS and enhanced MRI at 1 and 3 months after treatment, and then at 6-month intervals for patients with large HCC. Technique effectiveness was defined as the absence of enhancement of any areas of the mass on follow-up contrast-enhanced images at 1 month after treatment. Local tumor recurrence was defined as the appearance of an irregular nodular, scattered or eccentric pattern of peripheral enhancement around the ablation zone after MWA. Mortality related to thermal ablation was defined as death within 30 days after ablation. Major complications were defined as events that caused substantial morbidity and disability leading to an increased level of care or hospital admission or substantially prolonged hospital stay [Citation21]. Follow-up was conducted until the last visit or the time of death or recurrence.
Statistical analysis
Quantitative data were expressed as mean ± standard deviation, and qualitative data were expressed as frequency. OS and RFS rates were assessed using the Kaplan–Meier method with the log-rank test. Statistical analyses were performed using SPSS 19.0 software (SPSS Inc., Chicago, IL, USA). For all tests, a probability (p-values) of <.05 was considered statistically significant.
Results
Patient characteristics
The characteristics of patients are shown in . The mean age and sex were comparable between the two groups (p = .592 and p = .756, respectively). The mean maximum diameter and location of HCC were comparable between the groups (p = .497 and p = .432, respectively). Comorbidities were also comparable between the two groups (p = .446). A total of 13 patients in the 3D group had liver function scores of Child-Pugh class A and 6 had scores of Child-Pugh B; in the control group, 16 patients had scores of Child-Pugh class A and 5 had scores of Child-Pugh B. There were no significant differences (p = .782). Median alpha-fetoprotein was 221.8 μmol/L in the 3D group and 173.8 μmol/L in the control group, with no significant difference (p = .126). All large HCCs (diameter ≥5 cm) were successfully scanned by contrast-enhanced MRI before MWA and within 1 week after MWA, which was planned and evaluated with a 3D visualization platform in the 3D group. A thermal monitoring system was successfully implemented to display the temperature during ablation in real time.
Table 2. The characters of patients and tumors.
In this study, the duration of 3D visualization image fusion cost 127.5 ± 10.1 s (range, 77.1–150.3 s). The registration error was 1.9 ± 0.4 mm. The median volumes of tumors and ablative areas were 74.1 ml and 149.0 ml, respectively. According to the 3D visualization preoperative plan, all operative procedures were successfully completed. Treatment effects were evaluated using a tumor map. After the first treatment, the tumor map showed that nine patients (47.4%) achieved a 5-mm safety margin; five patients (26.3%) achieved complete ablation but failed to reach a 5-mm safety margin and five (26.3%) patients failed to achieve complete ablation. A second treatment was arranged for patients with residual tumor, until complete ablation. Among these patients, two had tumors that were too close to the diaphragm to be clearly visualized by us, and one tumor was adjacent to the gastrointestinal tract.
Treatment parameters
The parameters related to simulated ablation in preoperative planning and actual ablation are shown in . The mean number of antennas was 2 in both the 3D and control groups. The median number of insertions was 6.6 in the 3D group and 5.0 in the control group, with a significant difference (p = .016). The median ablation time was 2830.5 s in the 3D group and 2727.1 s in the control group, with no significant difference (p = .711). The median ablative power was 55.5 W in the 3D group and 55.5 W in the control group, which was not significant (p = .975). The median number of sessions was 1.4 and the primary efficacy rate was 47.4% in the 3D group. In the control group, the median number of sessions was 2.0 and the primary efficacy rate was 14.3%. There were statistically significant differences between the two groups (p = .001 and p = .007, respectively).
Table 3. Thermal ablative parameters.
Midterm oncologic outcome and recurrence
The median follow-up period was 18.7 months (range, 5.2–42.5 months). On the basis of follow-up imaging, 100% technique effectiveness was achieved in both groups (3D group: 19 of 19 treatments; control group: 21 of 21 treatments). Local tumor recurrence was observed in six (31.6%) patients at 9.2, 21.2, 22.9, 27.5, 29.2 and 32.8 months after MWA in the 3D group and in eight (38.1%) patients at 7.3, 7.5, 9.4,11.2 11.4, 11.9, 14.6, 15.8 and 37.2 months after MWA in the control group. Thrombus of the left portal vein was observed in one (2.5%) patient at 12.1 months after MWA. Intrahepatic distant recurrence was observed in eight (42.1%) patients at 11.3, 22.1, 22.3, 24.3, 27.6, 27.7, 29.6 and 32.8 months after MWA in the 3D group and in nine (42.9%) patients at 11.8, 17.5, 19.7,21.4 21.4, 23.9, 27.7, 30.8 and 35.8 months after MWA in the control group. Distant metastasis to the adrenal gland, lung and bone was observed in three (14.3%) patients at 11.7, 21.4 and 13.1 months after MWA in the control group. HCC progression resulting in death occurred in four (21.1%) patients at 5.2, 6.4, 21.3 and 52 months after MWA in the 3D group and in four (19.0%) patients at 5.0, 7.3, 15.3 and 34.2 months after MWA in the control group. The 1-, 2- and 3-year OS rates in the 3D and control groups were 94.7%, 75.8% and 38.5%; and 100%, 67.6% and 34.4%, respectively (), with no significant difference (p = .745). The 1-, 2- and 3-year RFS rates in the 3D and control groups were 100%, 72.7% and 64.6%; and 89.5%, 37.6% and 18.8%, respectively (), with a statistically significant difference (p = .014). There were no major complications (intestinal perforation, reactive hydrothorax and skin burn) related to MWA. Minor complications were fever (50.0%), abdominal pain (32.5%) and hemoglobinuria (12.5%). All side effects resolved with conservative treatment within 24–72 h.
Figure 4. (A) The 1-, 2- and 3-year OS rates in 3D group and control group were 94.7%, 75.8% and 38.5% and 100%, 67.6% and 34.4%, respectively, showing no significant statistical difference (p = .745). (B) The 1-, 2- and 3-year RFS rates in 3D group and control group were 100%, 72.7% and 64.6% and 89.5%, 37.6% and 18.8%, respectively, showing significant statistical difference (p = .014).
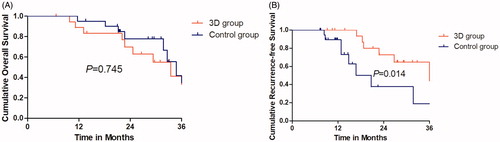
Discussion
Many studies have reported the therapeutic equivalence between image-guided thermal ablation and surgical resection for small HCC [Citation6]. However, these treatments have not produced satisfactory efficacy for larger tumors because of limited heat conduction, severe impairment of liver function and adjacent vital organs. It is difficult to achieve complete eradication of large tumors by relying solely on thermal ablative techniques [Citation14,Citation22]. In order to solve the above problems, a new strategy of 3D visualization assist with US-PMWA following TACE was adopted for treatment of 14 patients with large HCC in this study. The treatment principle is that TACE decreases arterial flow to the tumor, thereby increasing the effectiveness of MWA and needle planning and multipoint ablation are performed, assisted by a 3D visualization treatment platform. In fact, several studies have demonstrated the synergistic cytotoxic effects of TACE combined with thermal ablation for large HCC [Citation23]. In addition, the 3D visualization treatment platform serves to assist the interventional radiologist to institute the treatment protocol, including number, embedding path, angles of the antennas and thermal field scope, which improves the accuracy of interventional therapy.
Preoperative treatment planning and accurate post-procedural evaluation are important to optimize the treatment strategy. Treatment of large HCC is challenging because of the complex anatomy involved. In addition, the spatial relationships between the tumor and surrounding structures are difficult to discern on 2D images and are thus subject to the perception of the operator. By contrast, 3D images have stereoscopic characteristics and have been used widely in surgery and interventional therapy [Citation24]. Our team began to use a 3D visualization operative treatment planning system for US-guided MWA of HCC in 2012 [Citation25], which improved the rate of complete ablation by one session. In addition, larger hepatic hilum HCC (diameter ≥3 cm) was treated with US-PMWA and the 3D visualization operative treatment planning system in a recent study, which resulted in good oncological outcomes [Citation26]. In the present study, a new function module was used in US-PMWA for large HCC, which was post-procedural evaluation based on a tumor map generated by 3D visualization image fusion. In the 3D visualization treatment platform, the spatial relationship between the tumor and vital organs can be displayed in three dimensions, the range of the thermal field and insertion route can be simulated and the number of multiple antennas and ablation sites can be calculated. Moreover, a 3D fusion image can facilitate accurate assessment and more objective judgment than conventional side-by-side image assessment. The tumor map was simpler and more quantitative owing to the following advantages: the 3D surface of the tumor covered by the ablative area was represented on a 2D map, and a traffic light color scheme was used to emphasize and quantitatively describe the ablation status and differences in the ablative effect within the entire tumor.
In consideration of the hepatic reserve function, 3D visualization preoperative planning is useful to interventional radiologists when calculating the number of antennas, insertion sites and sessions, as well as the distance between the tumor and vital organs and vessels [Citation26]. If the tumor is found to be incompletely ablated by CEUS after MWA, an additional session can be scheduled to allow for the recovery of liver function. To achieve a good treatment outcome, enhanced MRI should be performed as soon as possible. 3D fusion images can then be acquired to construct a tumor map. According to the location and proportion of residual tumors on the tumor map, additional preoperative 3D visualization can be planned. In this study, the tumor map demonstrated the ablative effect of the tumors and showed the proportion and distribution of the residual tumor. Among the patients in the 3D group, according to an accurate plan of insertion, the number of insertions was increased and was greater than the number in the control group. Moreover, complete ablation was achieved in 11 (57.9%) patients in one session; the remaining patients achieved complete ablation in two sessions. In contrast, patients underwent more sessions and had a higher recurrence rate in the control group, which mainly originated from the absence of preoperative planning and the subjectivity and random actions of the radiologist.
In recent studies, Zuo et al. [Citation27] reported that the control rates of large HCC were 100.0%, 92.4%, 87.9% and 70.1% at 1, 3, 6 and 12 months after TACE combined with RFA, respectively. Ke et al. [Citation28] reported on repeated radiofrequency ablation combined with TACE/TAE in solitary large HCC. The cumulative OS rates at 1, 2 and 3 years were 53.3%, 29.2% and 16.7%, respectively, and the cumulative LTP-free rates at 1, 2 and 3 years were 82.9%, 73.9% and 18.8%, respectively. In our study, patients with a single large HCC who underwent US-PMWA assisted by the 3D visualization platform seemed to achieve more ideal outcomes, comparable to previous results. The functionality of the 3D visualization platform was fully utilized, which improved treatment efficiency in actual clinical operation.
There were some limitations to this study that should be addressed. First, this was a preliminary study with a small sample size. Therefore, further randomized controlled multicenter studies with larger sample sizes and longer follow-up periods are necessary to verify these results. Second, the accuracy of the proposed method with use of a tumor map should be further examined. Third, all operators had extensive experience with MWA; thus, a different 3D visualization treatment platform may be needed for less experienced operators.
US-PMWA with a 3D visualization treatment platform appears to be a safe and effective technique for the management of large HCC (diameter ≥5 cm). 3D visualization treatment platforms reveal the spatial structure of the tumor and the surrounding structures, and a tumor map can provide objective assessment, to improve the effectiveness of MWA. The proposed combination treatment meets [Citation27] the requirements of precise medical treatment that is easier for interventional radiologists to operate and achieve a 5-mm ablative margin.
Disclosure statement
No potential conflict of interest was reported by the authors.
Additional information
Funding
References
- El-Serag HB. Hepatocellular carcinoma. N Engl J Med. 2011;365:1118–1127.
- Zuo TT, Zheng RS, Zhang SW, et al. Incidence and mortality of liver cancer in China in 2011. Chin J Cancer. 2015;34:508–513.
- Tang A, Hallouch O, Chernyak V, et al. Epidemiology of hepatocellular carcinoma: target population for surveillance and diagnosis. Abdom Radiol (NY). 2018;43:13–25.
- Liu X, Wang Z, Chen Z, et al. Efficacy and safety of transcatheter arterial chemoembolization and transcatheter arterial chemotherapy infusion in hepatocellular carcinoma: a systematic review and meta-analysis. Oncol Res. 2017;26:231.
- Wang YM, Qian GJ, Xu Y, et al. Efficacy of microwave ablation in treatment of hepatocellular carcinoma within the Milan criteria: a report of 696 cases. Zhonghua Gan Zang Bing Za Zhi. 2017;25:344–348.
- Lucchina N, Tsetis D, Ierardi AM, et al. Current role of microwave ablation in the treatment of small hepatocellular carcinomas. Ann Gastroenterol. 2016;29:460–465.
- Sacco R, Tapete G, Simonetti N, et al. Transarterial chemoembolization for the treatment of hepatocellular carcinoma: a review. Jhc. 2017;4:105–110.
- Liang P, Yu J, Yu XL, et al. Percutaneous cooled-tip microwave ablation under ultrasound guidance for primary liver cancer: a multicentre analysis of 1363 treatment-naive lesions in 1007 patients in China. Gut. 2012;61:1100–1101.
- Liang P, Yu J, Lu MD, et al. Practice guidelines for ultrasound-guided percutaneous microwave ablation for hepatic malignancy. Wjg. 2013;19:5430–5438.
- Oliveri RS, Wetterslev J, Gluud C. Hepatocellular carcinoma. Lancet. 2012;380:470.
- Lin YH, Chiou YY, Chou YH, et al. Radiofrequency ablation for recurrent hepatocellular carcinoma in postresectional patients: prognostic factors analysis. Eur J Gastroenterol Hepatol. 2011;23:1239–1244.
- Ke S, Gao J, Kong J, et al. Repeated radiofrequency ablation combined with ablated lesion elimination and transarterial chemoembolization improves the outcome of solitary huge hepatocellular carcinomas 10 m or larger. Medicine (Baltimore). 2016;95:e3393.
- Saviano A, Iezzi R, Giuliante F, et al. Liver resection versus radiofrequency ablation plus transcatheter arterial chemoembolization in cirrhotic patients with solitary large hepatocellular carcinoma. J Vasc Interv Radiol. 2017;28:1512–1519.
- Groeschl RT, Pilgrim CH, Hanna EM, et al. Microwave ablation for hepatic malignancies: a multiinstitutional analysis. Ann Surg. 2014;259:1195–1200.
- Kim SS, Kang TW, Kim M, et al. Initial radiofrequency ablation failure for hepatocellular carcinoma: repeated radiofrequency ablation versus transarterial chemoembolisation. Clin Radiol. 2018;73:216.e1–216.e8.
- Facciorusso A, Serviddio G, Muscatiello N. Local ablative treatments for hepatocellular carcinoma: an updated review. Wjgpt. 2016;7:477–489.
- Makino Y, Imai Y, Igura T, et al. Usefulness of the extracted-overlay function in CT/MR-ultrasonography fusion imaging for radiofrequency ablation of hepatocellular carcinoma. Dig Dis. 2013;31:485–489.
- Passera K, Selvaggi S, Scaramuzza D, et al. Radiofrequency ablation of liver tumors: quantitative assessment of tumor coverage through CT image processing. BMC Med Imaging. 2013;13:3.
- Wang XL, Li K, Su ZZ, et al. Assessment of radiofrequency ablation margin by MRI-MRI image fusion in hepatocellular carcinoma. Wjg. 2015;21:5345–5351.
- Beigi P, Malenfant P, Rasoulian A, et al. Three-dimensional ultrasound-guided real-time midline epidural needle placement with epiguide: a prospective feasibility study. Ultrasound Med Biol. 2017;43:375–379.
- Ahmed M, Solbiati L, Brace CL, et al. Image-guided tumor ablation: standardization of terminology and reporting criteria-a 10-year update. Radiology. 2014;273:241–260.
- Medhat E, Abdel AA, Nabeel M, et al. Value of microwave ablation in treatment of large lesions of hepatocellular carcinoma. J Dig Dis. 2015;16:456–463.
- Tamai T, Oshige A, Tabu K, et al. Utility of percutaneous radiofrequency ablation alone or combined with transarterial chemoembolization for early hepatocellular carcinoma. Oncol Lett. 2017;14:3199–3206.
- Hansen C, Wieferich J, Ritter F, et al. Illustrative visualization of 3D planning models for augmented reality in liver surgery. Int J Cars. 2010;5:133–141.
- Liu F, Liang P, Yu X, et al. A three-dimensional visualisation preoperative treatment planning system in microwave ablation for liver cancer: a preliminary clinical application. Int J Hyperthermia. 2013;29:671–677.
- Lopresto V, Pinto R, Farina L, et al. Microwave thermal ablation: effects of tissue properties variations on predictive models for treatment planning. Med Eng Phys. 2017;46:63–70.
- Zuo TY, Liu FY, Wang MQ, et al. Transcatheter arterial chemoembolization combined with simultaneous computed tomography-guided radiofrequency ablation for large hepatocellular carcinomas. Chin Med J. 2017;130:2666–2673.
- Yan JY, Zhang JL, Wang MQ, et al. Combined transcatheter arterial chemoembolization and radiofrequency ablation in single-session for solitary hepatocellular carcinoma larger than 7 cm. Asia Pac J Clin Oncol. 2017.