Abstract
Purpose: We investigated the relationships between KRAS gene status and local tumor progression (LTP) of colorectal liver metastases (CLMs) after treatment with percutaneous ultrasound-guided radiofrequency ablation (RFA).
Materials and methods: Clinical and imaging data from 76 patients (154 lesions) with CLM who underwent percutaneous ultrasound-guided RFA and had KRAS gene test results between January 2012 and June 2016 were analyzed. The average lesion size was 2.3 ± 1.0 cm (range 0.9–5.7 cm); 38 cases (82 lesions) had wild-type KRAS, and 38 cases (72 lesions) had KRAS mutations.
Results: The technique effectiveness was 98.1% (151/154), and the LTP rate was 18.2% (28/154) after RFA, which was performed between January 2012 and November 2017. The mean and median follow-up were 32.7 ± 2.5 and 32.0 ± 2.6 months (range 1–70 months), respectively. Cumulative LTP rates at 6 months and 1, 2 and 3 years post-RFA for all patients were 7.4, 14.5, 17.8 and 19.2%, respectively. The LTP rate for patients with mutant KRAS (27.8% [20/72]) was significantly higher than that in patients with wild-type KRAS (9.8% [8/82]; p = .004). The cumulative LTP rates at 6 months and 1, 2 and 3 years post-RFA were 4.0, 11.1, 11.1 and 11.1%, respectively, for patients with wild-type KRAS and 11.2, 18.4, 25.2 and 36.2%, respectively, for patient with mutant KRAS (p = .011). Univariate (p = .011) and multivariate analyses (p = .005) showed that KRAS genotype in liver metastases was predictive of LTP. Multivariate analysis also showed that ablation margin size (p< .001) and modified clinical risk score (CRS; p = .033) were independent prognostic factors for LTP.
Conclusions: KRAS gene status of liver metastatic lesions was associated with LTP rates after RFA of CLM. Ablation margin size and modified CRS were also independent prognostic factors for LTP.
Introduction
The liver is a common metastatic site for colorectal cancer [Citation1], and approximately 20–50% of patients with colorectal cancer have liver metastases [Citation2,Citation3]. Surgical resection is the standard treatment for colorectal liver metastases (CLMs). Due to tumor burden and clinical complications, only 17–20% of patients with CLMs can be treated with surgical resection [Citation4,Citation5]. Tanis et al. [Citation6] compared the resection arm of a randomized controlled trial (RCT) of chemotherapy with the radiofrequency ablation (RFA) arm of a similar RCT and showed that when lesion size was limited to 30 mm, the local recurrence rates of surgical resection and RFA were 5.5 and 2.9% per lesion, respectively. There were no major differences in outcomes between resection and RFA for smaller tumors.
In a previous report, for patients with CLMs, local tumor progression (LTP) after treatment with RFA (10%) was lower than that after wedge resection (19%) [Citation7]. Similarly, in an RCT describing RFA (with or without resection) [Citation8], 119 patients with unresectable CLMs received systemic treatment alone or systemic treatment plus aggressive local treatment by RFA. The results showed that 3-, 5- and 8-year overall survival (OS) rates were 56.9, 43.1 and 35.9%, respectively, in the RFA + chemotherapy group and 55.2, 30.3 and 8.9%, respectively, in the chemotherapy-only group. Moreover, aggressive local treatment prolonged OS, and the LTP rate was higher in the chemotherapy-only group. Their results also suggested that RFA may be an effective treatment for CLM [Citation9,Citation10].
The factors affecting LTP rates have been investigated in patients with CLMs who were treated with RFA. For example, Shady et al. [Citation11] showed that a margin of over 5 mm was critical for local tumor control with tumors ablated. The same investigators [Citation12] indicated that no LTP was observed for tumors ablated with margins over 10 mm, regardless of the use of RFA or microwave ablation. Additionally, Gu et al. [Citation13] proposed that the location of the primary tumor and size of the tumor may be independent prognostic factors for patients with CLMs treated with RFA; patients with left-sided lesions or tumors less than 3 cm in size had low LTP rates. Other studies [Citation14–16] demonstrated the presence of tumor cells in biopsy samples taken from ablation areas. Sotirchos et al. [Citation14] reported that there was only one residual tumor of 67 original tumors (2%) at the first post-RF ablation contrast-enhanced CT scan, which was performed within 4–8 weeks after ablation. Image-guided biopsy was performed from the tumor center and the suspected minimal margin of the ablation zone using coaxial 18–20-gauge core needles, and 16 viable tumors were identified by immunohistochemical analysis. LTP occurred in 11 (69%) of 16 lesions classified as viable and in 10 (20%) of 51 lesions classified as necrotic. Facciorusso et al. [Citation17] demonstrated that the ratio of lymphocytes to monocytes in patients with CLMs treated with RFA was an important factor affecting LTP. Additionally, Odisio et al. [Citation18] found that patients with CLMs who had prior hepatectomy had much lower LTP rates.
KRAS gene status is a potential prognostic marker for patients with CLMs [Citation19,Citation20]. Mutations in KRAS can promote tumorigenesis [Citation21,Citation22], possibly through vascular invasion and hematogenous metastasis [Citation23,Citation24]. Various studies have described the relationships between KRAS mutations and postoperative LTP rates in patients with CLMs; however, the results have varied. For example, Kemeny et al. [Citation25] and Vauthey et al. [Citation26] showed that patients with KRAS mutations had higher cumulative LTP rates. Others [Citation27] found that patients with KRAS mutations in codon 13 had high rates of extrahepatic recurrence. However, Osumi et al. [Citation28] and Margonis et al. [Citation29] showed that KRAS mutations were not associated with LTP.
Few studies have examined the relationships between gene mutations and LTP post-RFA in patients with CLMs. Odisio et al. [Citation18,Citation30] suggested that the RAS gene is an important factor affecting LTP in CLMs after RFA. This is consistent with the results of Shady et al. [Citation31], who found that KRAS mutation is a poor prognostic factor of LTP that becomes extremely important when associated with margins under 5 mm.
Accordingly, the purpose of this study was to analyze the relationships between KRAS gene status and LTP in patients with CLMs treated with percutaneous ultrasound-guided RFA.
Materials and methods
Patient selection and eligibility criteria
The patient database was searched for those with CLM whose records were created between January 2012 and June 2016. In total, 187 patients with CLM who underwent ultrasound-guided RFA were identified; 13 patients who were treated with open ultrasound-guided RFA were excluded. Among the 174 patients who had percutaneous ultrasound-guided RFA, 93 patients did not have genetic test results. For the remaining 81 patients, KRAS gene status was not available in five patients; therefore, 76 patients (154 lesions) were included in the current analysis ().
Ablation procedure
The treatment plan was decided by at least three physicians who were RFA specialists. The RFA device was selected based on the size, shape and location of the tumor. Patients received general anesthesia and then underwent ultrasound-guided RFA. All RFA procedures were performed by four radiologists (CMH, YK, WW and YW), who each had more than 10 years of experience in ultrasound-guided interventional procedures. The ablation procedure was performed by two physicians together. One physician operated the ultrasound instrument to locate and guide lesions in real time and performed enhanced ultrasound if necessary; the other physician was the operator, placing the electrode needle into the tumor. In this study, the Celon Lab Power ablation system (Olympus, Germany) was used for 132 lesions, the Valleylab system (Tyco Healthcare, North Haven, CT) was used for 11 lesions, and the RITA Model 1500X ablation system (AngioDynamics, Latham, NY) was used for 6 lesions. The MedSphere system (Medsphere Healthcare Corporation, Shanghai, China) was used for five lesions. In the pre-operative protocol, ablation margins were planned to be at least 5 mm, except for tumors adjacent to major structures, such as the diaphragm, bowel, gallbladder, kidney or large blood vessels. Collateral thermal injury to the adjacent structures is still a technical complication of percutaneous RFA [Citation32]. A prior study showed that hydrodissection is technically feasible and effective for improving protection of the adjacent structures by separating them away from the liver capsule [Citation33,Citation34]. In this study, 62 lesions (42 patients) were subjected to hydrodissection due to their location near the diaphragm, bowel, gallbladder or kidney. Forty lesions (19 patients) were adjacent to the diaphragm, 11 lesions (10 patients) were adjacent to the bowel, four lesions (four patients) were adjacent to the gallbladder, and seven lesions (six patients) were adjacent to the kidney. Patients were closely monitored for any complications postablation. There were no differences in therapies received by patients harboring wild-type or mutant KRAS after RFA.
KRAS mutation profiling
The tumor samples were surgically resected from the primary tumor and subjected to hematoxylin and eosin (HE) staining and DNA extraction. KRAS genotype (including codons 12 and 13) was determined by direct sequencing or using spectrometry.
Assessment of therapeutic efficacy and follow-up
Enhanced computerized tomography (CT) or magnetic resonance imaging (MRI) and ultrasound of the abdomen within 1 month prior to RFA were performed. All related preprocedural examinations were performed within 1 month prior to RFA. At 1 month after RFA, enhanced CT or contrast-enhanced ultrasonography (CEUS) was performed to determine whether the lesions remained in order to evaluate the effectiveness of RFA. As described in a previous report [Citation35], anatomic landmarks around the tumor were chosen. The pre- and 1-month postablation CT images were reviewed side by side to measure the distances from the edge of the tumors to the landmarks, allowing us to select the minimum of multiple margin values on CT as the minimal margin. The margin size was assessed and categorized as 0–5, 6–10, or >10 mm.
Patients were examined with CEUS, enhanced CT, or MRI every 3 months in the first 2 years after RFA and every 6 months at 2 years after RFA. LTP rates and OS were recorded. The time between the diagnosis of liver metastasis and RFA ranged from 0 to 63 months (mean: 11.2 ± 10.4 months), and the time between the diagnosis of primary cancer and RFA ranged from 3 to 92 months (mean: 16.5 ± 14.1 months).
Definitions
Technical efficacy [Citation36] refers to treatment of the tumor and complete replacement by ablation zones, with assessment of tumor coverage during the first enhanced imaging follow-up 4 weeks post-RFA. LTP [Citation36] describes the appearance of tumor foci at the edge of the ablation zones after at least one contrast-enhanced follow-up study has documented adequate ablation and covered by the ablation zone in the target tumor and surrounding ablation margin using imaging criteria. OS [Citation36] was the time from the start of ablation treatment to death or the last follow-up. Major complications [Citation36] were events leading to substantial morbidity and disability (e.g., resulting in unexpected loss of an organ), necessitating increased levels of care, hospital admission or lengthened hospital stay. All other complications were considered minor.
Statistics analysis
Statistical analysis was performed using SPSS 19.0 (SPSS Inc., Chicago, IL). In this study, chi-square tests were used to compare the clinical characteristics and LTP rates of patients with wild-type and mutant KRAS. The Kaplan–Meier method and log-rank method were used for LTP analysis and univariate analysis. The variables of interest identified in the univariate analysis were further analyzed in the multivariate analysis using the Cox regression model. OS was analyzed using the Kaplan–Meier method. Variables included in the LTP analysis were as follows: sex and age of the patient, primary site of the tumor, pathological differentiation of the primary lesion, lymph node metastasis, depth of invasion, time of liver metastasis, ablative margin size, carcinoembryonic antigen, clinical risk score (CRS), presence or absence of extrahepatic metastases before RFA, number of liver metastases, history of liver metastasis resection, KRAS genotype and lesion size after treatment with RFA.
Results
Technique effectiveness
Technique effectiveness was 98.1% (151/154) for lesions treated during the first enhanced imaging follow-up 1 month post-RFA. Residual lesions were found in three lesions (three patients) that were adjacent to blood vessels, the biliary tract, or the gastrointestinal tract. Wild-type KRAS was found in two of three patients. In this study, three of 154 lesions were found to have residual tumors, and many new metastases were found in the liver at 1 month after ablation. The clinician decided to administer systemic chemotherapy without repeated ablation. In contrast, eight of 28 locally progressed lesions were treated with repeated ablation. Moreover, 26.5% (9/34) of patients were found to have new liver metastases within 30 d after ablation. Additionally, 44.4% (4/9) of patients had multiple metastases, and 55.6% (5/9) of patients had solitary metastases. There were no extrahepatic metastases within 30 d after ablation.
LTP analysis
Baseline characteristics and clinical data of the 76 (154 lesions) patients with wild-type or mutant KRAS are shown in . No significant differences were found in the age, sex, location of primary lesions, extrahepatic metastases or time of metastasis between patients with wild-type and mutant KRAS.
Table 1. Baseline characteristics of patients with colorectal liver metastases.
The overall LTP rate was 18.2% (28/154) after RFA, and patients were regularly followed up until November 2017. The median follow up was 32.0 ± 2.6 months (range: 1–70 months). The cumulative LTP rates at 6 months, 1 year, 2 years and 3 years post-RFA for all patients were 7.4, 14.5, 17.8 and 19.2%, respectively. The LTP rate was 27.8% (20/72) for mutant KRAS, which was significantly higher than that in patients with wild-type KRAS (9.8% [8/82], p = .004). When the ablation margin size of the tumor lesions was less than or equal to 5 mm, the LTP rates for wild-type and mutant KRAS were 44.4% (4/9) and 77.8% (7/9), respectively; the difference was not significant (p = .335). When the ablation margin size of the tumor lesions was greater than 5 mm, the LTP rates for wild-type and mutant KRAS were 5.5% (4/73) and 20.6% (13/63), respectively; the difference was significant (p = .019).
The cumulative LTP rates at 6 months, 1 year, 2 years and 3 years post-RFA were 4.0, 11.1, 11.1, and 11.1%, respectively, for patients with wild-type KRAS and 11.2, 18.4, 25.2 and 36.2%, respectively, for those with mutant KRAS; the difference was significant (p = .011).
Univariate analysis showed that KRAS genotype in liver metastases (p = .011), tumor size (p = .002), ablation margin size (p< .001) and CRS (p = .038) were predictors of LTP (). Using the multivariate regression model, wild-type KRAS (p = .005, hazard ratio [HR] = 0.309), margin size (p< .001, HR = 0.180), and modified CRS (p = .033, HR = 2.692) were found to be independent prognostic factors of LTP (). Patients with mutant KRAS, CRS 3–5, and margins of 5 mm or less had higher LTP rates than the patients with wild-type KRAS, CRS 0–2, and margins of greater than 5 mm (p < .001).
Figure 2. (a) Local tumor progression and KRAS gene status (p=.011). (b) Local tumor progression and tumor size (p=.002). (c) Local tumor progression and ablation margin size (p<.001). (d) Local tumor progression and CRS (p=.038).
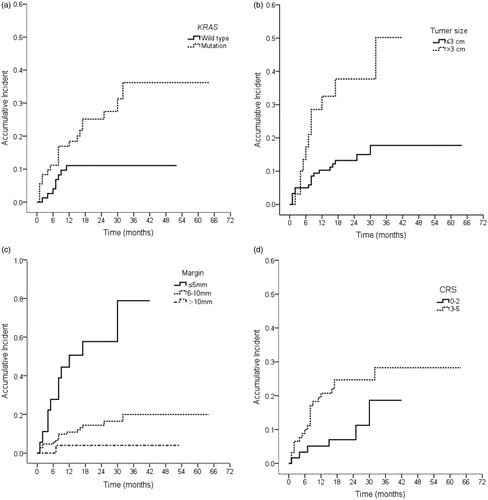
Table 2. Univariate and multivariate analyses for factors affecting local tumor progression.
New liver metastases
New liver metastases were found in 44.7% (34/76) of patients, including 39.5% (15/38) of patients with wild-type KRAS and 50.0% (19/38) of patients with mutant KRAS; the difference was not significant (p = .356). The 1-, 2- and 3-year cumulative survival rates in patients without new liver metastases were 63.4, 60.1 and 54.6%, respectively, for patients with wild-type KRAS and 57.0, 53.9 and 40.4%, respectively, for patients with mutant KRAS (p = .629). New liver metastases were not identified as important factors affecting LTP (p = .956) and OS (p = .647). New extrahepatic metastasis was a prognostic factor for OS (p = .007).
Survival
The 1-, 2- and 3-year survival rates were 89.5, 65.0 and 34.9%, respectively; the median survival time was 32.0 months. KRAS gene status (p = .228) did not predict OS.
Complications
In total, 154 lesions were treated with RFA. Major complications occurred in five patients. Three patients developed hepatic hematoma, which improved after conservative treatment; one patient developed proteinuria and improved after alkalinization of the urine; and one patient had abdominal pain, fever and biliary tract infection after ablation. The lesion was adjacent to the hilar bile duct, and the pre-RFA ultrasound showed expansion of the bile duct behind the tumor. Cephalosporin combined with metronidazole was used for treatment. Additional symptoms were alleviated after conservative treatment.
Discussion
RFA is a safe and effective method for the treatment of CLMs [Citation37,Citation38]. Ruers et al. [Citation8] demonstrated a survival benefit with the use of RFA in addition to chemotherapy. However, approximately 12–40% of patients had LTP post-RFA [Citation9,Citation39], and managing LTP remains a clinical challenge. In this study, we found that KRAS gene status was an independent factor affecting the prognosis of patients with CLMs.
KRAS protein is a basic component of the epidermal growth factor receptor-signaling cascade. Activating mutations in the KRAS gene cause constitutive activation of Ras GTPase, which leads to excessive activation of the downstream Raf/extracellular signal-regulated kinase/mitogen-activated protein kinases and other signaling pathways, thereby resulting in cell transformation and tumorigenesis.
Many studies have reported the relationship between the KRAS gene and LTP in patients who underwent surgical resection. Vincenzi et al. [Citation20] and Vauthey et al. [Citation26] suggested that KRAS mutations could predict the rate of LTP in patients with colorectal cancer who underwent surgical resection. Renaud et al. [Citation40] proposed that KRAS gene status may be used as a predictive marker for patients with colorectal lung metastases who received surgical treatment. In another study [Citation41], analysis of KRAS gene status in 918 patients with CLMs showed that 477 patients had wild-type KRAS and 441 had KRAS mutations, and higher cumulative LTP rates were found at specific sites, such as the brain, bones and lungs, for patients with mutant KRAS.
In this study, we found that patients with KRAS mutations had a higher cumulative LTP rate than patients with wild-type KRAS. Similarly, Calandri et al. [Citation30] reported that mutant RAS was an independent predictor of LTP; patients with mutant KRAS had higher LTP rates than patients with wild-type KRAS. Shady et al. [Citation31] added a significant threshold: a margin of 5 mm was found to be particularly important for KRAS mutant tumors. In the subset of patients with KRAS mutation, a suboptimal margin carried a significant risk (HR: 16.8) for LTP when compared with wild-type KRAS ablated with margins of greater than 5 mm, highlighting the importance of modifying technique based on KRAS status. Adequate safety margins are a critical determinant of LTP after ablation of liver tumors [Citation11,Citation12,Citation14,Citation30]. In this study, margin size was a significant predictor of LTP for CLMs. Consistent with these findings, Shady et al. [Citation12] proposed that a margin of over 5 mm was critical for local tumor control with tumor ablation, a margin of 10 mm is associated with no recurrence, regardless of the use of RFA or MWA. Additionally, Shady et al. [Citation11] demonstrated that if the margins were over 10 mm, local tumor control of over 90% could be achieved at more than 4 years after ablation. Moreover, a previous study [Citation35] revealed that a minimal margin of greater than 5 mm was a critical factor for local tumor control.
In our study, the modified CRS was a predictor of LTP. The modified CRS was determined based on the original surgical CRS, which included node-positive primary tumors, the disease-free interval from primary tumor resection to CLM detection, the number of liver tumors, the size of the largest tumor, and carcinoembryonic antigen (CEA) levels. We defined the modified CRS as described in a previous report [Citation11], in which the modified CRS was also found to be a predictor of LTP. Additionally, Sofocleous et al. [Citation42] indicated that patients with a modified CRS (not including CEA levels) of 0–2 had higher LTP than those with a modified CRS of 3–4 at 1 year after a single ablation. However, Shady et al. [Citation12] demonstrated that there was no significant difference in tumor progression between patients with CRS 0–2 and CRS 3–5. Thus, more studies involving greater numbers of patients are needed to confirm these findings. If the application of such a CRS may help identify patients at high risk, patient outcomes may be improved via appropriate use of follow-up schedules or combined therapies.
There are many factors affecting LTP in patients with CLMs who were treated with RFA [Citation14,Citation35,Citation43], including tumor size, surviving tumor cells, lymphocyte to monocyte ratio and history of liver metastasis resection pre-RFA. In our study, univariate analyses showed that lesion size was predictive of LTP. However, the improvement of ablation instruments has increased the maximum range of ablation. Pre-operative planning, multipoint ablation and multiple needles can be used for large tumors. Technical success can be achieved for tumors that are not located in special sites. Accordingly, lesion size was not found to be an independent predictor, similar to previous findings [Citation12,Citation31], and there were no significant associations between LTP and lesion size.
There were no significant differences in new liver metastases between patients with wild-type KRAS and mutant KRAS post-RFA. This was different from the findings reported by Shady et al. [Citation31]. Other studies [Citation40,Citation41] suggested that KRAS gene status is associated with tumor recurrence at specific sites, such as the lungs, bones and brain. Further studies are needed to elucidate the relationships between the KRAS gene and new liver metastases.
The 1-, 2- and 3-year survival rates were 89.5, 65.0 and 34.9%, respectively, consistent with a recently published report [Citation11]. However, Shady et al. [Citation31] suggested that KRAS gene status was related to OS for CLMs treated with RFA. In contrast, our findings showed that there were no significant differences in OS between patients with wild-type KRAS and mutant KRAS. Therefore, the relationship between KRAS gene and OS in patients with CLMs who received RFA is still unclear and should be studied in greater detail using larger sample sizes.
The KRAS genotype in liver metastases was treated similarly to the genotype of the primary lesions in this study. Loes et al. [Citation44] showed that 13 of 94 patients exhibited mutation heterogeneity across metastatic deposits for at least one gene. However, Voutsina et al. [Citation45] and Tie et al. [Citation46] found that the genes of the primary tumor lesions were highly consistent with the genes of the metastatic sites. Knijn et al. [Citation47] studied 305 patients with colorectal cancer and found that the genes were consistent from the primary tumor lesions and metastatic sites in 98% of patients. Thus, this study considered that the KRAS genotype of the primary tumor and the metastatic sites were the same; further studies on the genotypes of liver metastases have been planned.
This study had some limitations. Although we found that the baseline characteristics of patients with wild-type KRAS and mutant KRAS were comparable and without significant differences, it was difficult to avoid bias since this study was a single-center retrospective study with a small sample size. Second, previous studies have shown that the KRAS gene is associated with metastases at specific sites among patients with CLMs who received surgical treatment. Because this was not explored in this study, various consecutive research projects have been planned to further investigate the relationships between the KRAS gene and specific site metastases. Finally, BRAF and NRAS genes have been reported to be associated with prognosis in patients with CLMs who underwent surgical treatment [Citation28,Citation48]. In this study, we only analyzed the relationships of the KRAS gene with OS and LTP rate. Relevant data are being collected and analyzed in order to elucidate the associations between other genes and prognosis in patients who treated with RFA.
Conclusions
KRAS gene status in the liver metastatic lesions was found to be associated with the rate of LTP after RFA in CLMs. KRAS mutation status in the ablated lesion significantly predicted LTP. In addition, ablation margin size and modified CRS were also independent prognostic factors for LTP.
Disclosure statement
The authors declare that they have no conflicts of interest concerning this article.
Additional information
Funding
References
- Elferink MA, de Jong KP, Klaase JM, et al. Metachronous metastases from colorectal cancer: a population-based study in North-East Netherlands. Int J Colorectal Dis. 2015;30:205–212.
- Hackl C, Gerken M, Loss M, et al. A population-based analysis on the rate and surgical management of colorectal liver metastases in Southern Germany. Int J Colorectal Dis. 2011;26:1475–1481.
- Leporrier J, Maurel J, Chiche L, et al. A population-based study of the incidence, management and prognosis of hepatic metastases from colorectal cancer. Br J Surg. 2006;93:465–474.
- Angelsen JH, Horn A, Sorbye H, et al. Population-based study on resection rates and survival in patients with colorectal liver metastasis in Norway. Br J Surg. 2017;104:580–589.
- Noren A, Eriksson HG, Olsson LI. Selection for surgery and survival of synchronous colorectal liver metastases; a nationwide study. Eur J Cancer. 2016;53:105–114.
- Tanis E, Nordlinger B, Mauer M, et al. Local recurrence rates after radiofrequency ablation or resection of colorectal liver metastases. Analysis of the European organisation for research and treatment of cancer #40004 and #40983. Eur J Cancer. 2014;50:912–919.
- Vietti Violi N, Duran R, Demartines N, et al. Local recurrence rate in patients with colorectal cancer liver metastasis after wedge resection or percutaneous radiofrequency ablation. International journal of hyperthermia: the official journal of European Society for Hyperthermic Oncology. North Am Hyperthermia Group. 2018;34:1020–1028. .
- Ruers T, Van Coevorden F, Punt CJ, et al. Local treatment of unresectable colorectal liver metastases: results of a randomized phase II trial. J Natl Cancer Inst. 2017;109:1–10.
- Solbiati L, Ahmed M, Cova L, et al. Small liver colorectal metastases treated with percutaneous radiofrequency ablation: local response rate and long-term survival with up to 10-year follow-up. Radiology. 2012;265:958–968.
- Garrean S, Hering J, Saied A, et al. Radiofrequency ablation of primary and metastatic liver tumors: a critical review of the literature. Am J Surg. 2008;195:508–520.
- Shady W, Petre EN, Gonen M, et al. Percutaneous radiofrequency ablation of colorectal cancer liver metastases: factors affecting outcomes–A 10-year experience at a single center. Radiology. 2016;278:601–611.
- Shady W, Petre EN, Do KG, et al. Percutaneous microwave versus radiofrequency ablation of colorectal liver metastases: ablation with clear margins (A0) provides the best local tumor control. J Vasc Intervent Radiol. 2018;29:268–275 e1.
- Gu Y, Huang Z, Gu H, et al. Does the site of the primary affect outcomes when ablating colorectal liver metastases with radiofrequency ablation? Cardiovasc Intervent Radiol. 2018;41:912–919.
- Sotirchos VS, Petrovic LM, Gonen M, et al. Colorectal cancer liver metastases: biopsy of the ablation zone and margins can be used to predict oncologic outcome. Radiology. 2016;280:949–959.
- Sofocleous CT, Nascimento RG, Petrovic LM, et al. Histopathologic and immunohistochemical features of tissue adherent to multitined electrodes after RF ablation of liver malignancies can help predict local tumor progression: initial results. Radiology. 2008;249:364–374.
- Sofocleous CT, Garg S, Petrovic LM, et al. Ki-67 is a prognostic biomarker of survival after radiofrequency ablation of liver malignancies. Ann Surg Oncol. 2012;19:4262–4269.
- Facciorusso A, Del Prete V, Crucinio N, et al. Lymphocyte-to-monocyte ratio predicts survival after radiofrequency ablation for colorectal liver metastases. World J Gastroenterol. 2016;22:4211–4218.
- Odisio BC, Yamashita S, Huang SY, et al. Impact of prior hepatectomy history on local tumor progression after percutaneous ablation of colorectal liver metastases. J Vasc Intervent Radiol. 2018;29:395–403.e1.
- Vogelstein B, Fearon ER, Hamilton SR, et al. Genetic alterations during colorectal-tumor development. N Engl J Med. 1988;319:525–532.
- Vincenzi B, Cremolini C, Sartore-Bianchi A, et al. Prognostic significance of K-Ras mutation rate in metastatic colorectal cancer patients. Oncotarget. 2015;6:31604–31612.
- Wood KW, Sarnecki C, Roberts TM, et al. Ras mediates nerve growth factor receptor modulation of three signal-transducing protein kinases: MAP kinase, Raf-1, and RSK. Cell. 1992;68:1041–1050.
- Troppmair J, Bruder JT, App H, et al. Ras controls coupling of growth factor receptors and protein kinase C in the membrane to Raf-1 and B-Raf protein serine kinases in the cytosol. Oncogene. 1992;7:1867–1873.
- Nash GM, Gimbel M, Shia J, et al. KRAS mutation correlates with accelerated metastatic progression in patients with colorectal liver metastases. Ann Surg Oncol. 2010;17:572–578.
- Varghese HJ, Davidson MT, MacDonald IC, et al. Activated ras regulates the proliferation/apoptosis balance and early survival of developing micrometastases. Cancer Res. 2002;62:887–891.
- Kemeny NE, Chou JF, Capanu M, et al. KRAS mutation influences recurrence patterns in patients undergoing hepatic resection of colorectal metastases. Cancer. 2014;120:3965–3971.
- Vauthey JN, Zimmitti G, Kopetz SE, et al. RAS mutation status predicts survival and patterns of recurrence in patients undergoing hepatectomy for colorectal liver metastases. Ann Surg. 2013;258:619–626.
- Margonis GA, Kim Y, Sasaki K, et al. Codon 13 KRAS mutation predicts patterns of recurrence in patients undergoing hepatectomy for colorectal liver metastases. Cancer. 2016;122:2698–2707.
- Osumi H, Shinozaki E, Suenaga M, et al. RAS mutation is a prognostic biomarker in colorectal cancer patients with metastasectomy. Int J Cancer. 2016;139:803–811.
- Margonis GA, Spolverato G, Kim Y, et al. Effect of KRAS mutation on long-term outcomes of patients undergoing hepatic resection for colorectal liver metastases. Ann Surg Oncol. 2015;22:4158–4165.
- Calandri M, Yamashita S, Gazzera C, et al. Ablation of colorectal liver metastasis: interaction of ablation margins and RAS mutation profiling on local tumour progression-free survival. Eur Radiol. 2018;28:2727–2734.
- Shady W, Petre EN, Vakiani E, et al. Kras mutation is a marker of worse oncologic outcomes after percutaneous radiofrequency ablation of colorectal liver metastases. Oncotarget. 2017;8:66117–66127.
- Chen TM, Huang PT, Lin LF, et al. Major complications of ultrasound-guided percutaneous radiofrequency ablations for liver malignancies: single center experience. J Gastroenterol Hepatol. 2008;23:e445–e450.
- Hinshaw JL, Laeseke PF, Winter TC, 3rd, et al. Radiofrequency ablation of peripheral liver tumors: intraperitoneal 5% dextrose in water decreases postprocedural pain. Am J Roentgenol. 2006;186:S306–S310.
- Liu CH, Yu CY, Chang WC, et al. Computed tomographic-guided percutaneous radiofrequency ablation with hydrodissection of hepatic malignancies in the subcapsular location: evaluation of safety and technical efficacy. J Chin Med Assoc. 2016;79:93–100.
- Wang X, Sofocleous CT, Erinjeri JP, et al. Margin size is an independent predictor of local tumor progression after ablation of colon cancer liver metastases. Cardiovasc Intervent Radiol. 2013;36:166–175.
- Ahmed M, Solbiati L, Brace CL, et al. Image-guided tumor ablation: standardization of terminology and reporting criteria–a 10-year update. Radiology. 2014;273:241–260.
- Vyslouzil K, Klementa I, Stary L, et al. [Radiofrequency ablation of colorectal liver metastases]. Zentralbl Chir. 2009;134:145–148.
- Weng M, Zhang Y, Zhou D, et al. Radiofrequency ablation versus resection for colorectal cancer liver metastases: a meta-analysis. PLoS One. 2012;7:e45493.
- Livraghi T, Solbiati L, Meloni F, et al. Percutaneous radiofrequency ablation of liver metastases in potential candidates for resection: the “test-of-time approach”. Cancer. 2003;97:3027–3035.
- Renaud S, Romain B, Falcoz PE, et al. KRAS and BRAF mutations are prognostic biomarkers in patients undergoing lung metastasectomy of colorectal cancer. Br J Cancer. 2015;112:720–728.
- Yaeger R, Cowell E, Chou JF, et al. RAS mutations affect pattern of metastatic spread and increase propensity for brain metastasis in colorectal cancer. Cancer. 2015;121:1195–1203.
- Sofocleous CT, Petre EN, Gonen M, et al. CT-guided radiofrequency ablation as a salvage treatment of colorectal cancer hepatic metastases developing after hepatectomy. J Vasc Intervent Radiol. 2011;22:755–761.
- Mahnken AH, Bruners P, Tacke JA, et al. [CT-guided radiofrequency ablation of liver metastases from colorectal cancer]. Dtsch Med Wochenschr. 2009;134:976–980.
- Loes IM, Immervoll H, Sorbye H, et al. Impact of KRAS, BRAF, PIK3CA, TP53 status and intraindividual mutation heterogeneity on outcome after liver resection for colorectal cancer metastases. Int J Cancer. 2016;139:647–656.
- Voutsina A, Tzardi M, Kalikaki A, et al. Combined analysis of KRAS and PIK3CA mutations, MET and PTEN expression in primary tumors and corresponding metastases in colorectal cancer. Mod Pathol. 2013;26:302–313.
- Tie J, Lipton L, Desai J, et al. KRAS mutation is associated with lung metastasis in patients with curatively resected colorectal cancer. Clin Cancer Res. 2011;17:1122.
- Knijn N, Mekenkamp LJ, Klomp M, et al. KRAS mutation analysis: a comparison between primary tumours and matched liver metastases in 305 colorectal cancer patients. Br J Cancer. 2011;104:1020–1026.
- Schirripa M, Cremolini C, Loupakis F, et al. Role of NRAS mutations as prognostic and predictive markers in metastatic colorectal cancer. Int J Cancer. 2015;136:83–90.