Abstract
Objective: To compare efficacy and safety of microbubble contrast agent (SonoVue) and transcatheter arterial chemoembolization (TACE) in combination with high-intensity focused ultrasound (HIFU) for treatment of patients with hepatocellular carcinoma (HCC).
Methods: According to our inclusion criteria, we retrospectively reviewed 52 patients with HCCs, and divided them into SonoVue group and TACE group. Tumors were examined by enhanced magnetic resonance imaging. Change of lesions, alpha-fetoprotein values, hepatic and renal function were measured pre- and postoperatively. Then, adverse events were observed and clinical follow-up was performed.
Results: Clinical efficacy and the majority of treatment parameters were similar, except for time and energy required for the first massive grey-scale changes in SonoVue group, which were significantly lower than those in TACE group (p < .05). For adverse events, only rate of fever (3.85%) in SonoVue group was significantly lower than that in TACE group (50.00%, p < .05). The ‘diagnosis and treatment cycle’ in SonoVue group (11.5 ± 2.9) was remarkably shorter than TACE group (22.7 ± 6.3, p < .05). Energy efficiency factor was positively correlated with distance from the deepest layer of lesion to the hepatic capsule or to the abdominal wall in SonoVue group, while that was negatively correlated with iodized oil deposition in TACE group.
Conclusion: Using microbubble (SonoVue) in HIFU procedure has a similar therapeutic effect compared with TACE, and does not increase the risk of treatment. It might present a new strategy in clinical treatment, especially for patients with a smaller diameter of HCC.
Introduction
Hepatocellular carcinoma (HCC), as one of the five most frequent cancers and the third leading cause of cancer-related deaths worldwide, is the commonest health problem in Asia, with the prevalence of disease set to rise dramatically [Citation1,Citation2]. At present, the primary treatment strategy for HCC is still surgery, however, only 15% of patients with surgical therapy can clinically benefit [Citation3]. Various techniques, such as transcatheter arterial chemoembolization (TACE), high-intensity focused ultrasound (HIFU), radiofrequency ablation, percutaneous ethanol injection, cryoablation, microwave ablation and stereotaxic radiotherapy have been applied to treat HCC patients [Citation4,Citation5].
HIFU is a noninvasive modality for the treatment of localized tumors. The low-energy ultrasound beam in vitro can be focused on tumor targets by ultrasonic focusing and directivity. The focal region can reach a high temperature (65–100 °C) within seconds through thermal, cavitation and mechanical effects, and cause the coagulation necrosis of the tumor without damaging to intervening tissue. Based on our knowledge, the distance traveled by ultrasound increases in tissue, therefore, the energy exponentially decays. Simultaneously, the greater blood flow in lesions, the more energy is scattered, according to the ‘blood cooling’ effect [Citation6]. Therefore, during the treatment of lesions in deep location or with hypervascular supply, it requires more HIFU energy deposition in the target region. Of note, increasing the therapeutic dose, through enhancing sonication power and prolonging duration of sonication, may enhance the risk of treatment [Citation7]. Thereby, changing the acoustic environment of tissue is a feasible and important strategy to improve the efficacy of HIFU. This study retrospectively analyzed the data of 52 patients with HCCs ≤ 5 cm underwent HIFU treatment during January 2015 to January 2018, compared the efficacy and safety between two different approaches to change the acoustic environment (microbubble ultrasound contrast agent vs. TACE), and initially investigated those factors affecting energy delivery.
Materials and methods
Patients and lesions
This study was a retrospective analysis of patients with HCC ≤5 cm treated in a single-center. The study approved by the Ethics Committee of the Second Affiliated Hospital of Chongqing Medical University (Chongqing, China), and a specific written informed consent was obtained from every patient before treatment.
The inclusion criteria were as follows: (1) HCC diagnosis was confirmed [Citation8]; (2) patients had an unresectable tumor or were unwilling to undergo surgery; (3) maximum diameter of tumor was ≤5 cm; (4) no inferior vena cava cancer thrombus; (5) Child-Pugh class A or B cirrhosis; (6) there were no serious diseases in heart, lung, kidney and other important organs and the eligible cases should be able to tolerate general anesthesia; and (7) patients understood HIFU treatment process and possible risks, and agree to undergo HIFU treatment. The exclusion criteria of this study were as follows: (1) metastatic hepatic neoplasm; (2) diffuse HCC; (3) patients with HIFU or TACE contraindications, such as severe heart failure, cerebrovascular disease, renal insufficiency, Child-Pugh class C with no improvement after liver protection therapy, and hemorrhagic tendency; and (4) uncontrolled diabetes.
From January 2015 to January 2018, all patients with HCC who matched the criteria and underwent HIFU treatment were enrolled. Those patients were divided into two groups: the SonoVue group (n = 26, 34 lesions), in which SonoVue was injected before starting HIFU sonication, and the TACE group (n = 26, 39 lesions), in which HIFU ablation was performed after one TACE treatment. This study considered the comparability of sex, age, body mass index (BMI), Child-Pugh class, positive result of alpha-fetoprotein (AFP) screening test, HBsAg (+), cases of cirrhosis, alanine aminotransferase (ALT), aspartate transaminase (AST), creatinine, tumor/node/metastasis (TNM) classification, number of lesions, position of lesions, diameter of lesions, distance from the deepest layer of lesion to the hepatic capsule, distance from the deepest layer of lesion to the abdominal wall and the blood supply of lesions between two groups.
TACE procedure
TACE was performed for patients in the TACE group 1–2 weeks before HIFU ablation. A 5-F angiographic catheter was introduced to perform celiac or common hepatic arteriography, after puncture of the right femoral artery. Feeding vessels of the nodules were selected with microcatheter. Then, an emulsion of iodized oil (Dianhuayou; Yantai Luyin Pharmaceutical Co., Ltd., Yantai, China) and Epirubicin Hydrochloride (Famaxin; Pfizer Pharmaceutical Co., Ltd., Wuxi, China) were administered in the feeding vessels. The intraoperative dosage of iodized oil (2–10 ml) and doxorubicin hydrochloride (10–40 mg) depended on the size of tumors.
HIFU procedure
A Model-JC Focused Ultrasound Tumor Therapeutic System (Chongqing Haifu Medical Technology Co., Ltd., Chongqing, China) equipped with a diagnostic ultrasound probe and a therapeutic transducer was used, which has been previously described [Citation9,Citation10]. A diagnostic ultrasound probe (3.5–5.0 MHz) realized the real-time ultrasound imaging to locate lesions and monitor treatment procedure. HIFU was undertaken by a therapeutic transducer with a diameter of 200 mm, a focal length of 155 mm, and a frequency of 0.96 MHz. The focal region was an ellipsoid with 3 and 8 mm in transverse and longitudinal directions. Radiation force balance method was used to map the acoustic pressure field of the focused transducer, and the focal peak intensities were from 1000 to 20,000 W cm−2.
A single-session HIFU treatment was performed for all patients. Before HIFU, colonic irrigation was carried out by liquid food, catharsis, as well as cleaning enema to protect intestine located in front of the target region. Skin of treatment area was prepared, defatted by 75% alcohol, and degassed by a vacuum extractor to reduce the risk of burn. An injection of normal saline into the pleural cavity was performed to separate the lung, if the acoustic pathway was blocked by lung tissue [Citation11]. In addition, the volume of saline depended on tumor position, which ranged from 200 to 600 ml. Patients, under general anesthesia, were placed in right lateral position or prone position on the treatment table, according to the position of lesions. Moreover, the skin of the target area was fully immersed in the degassed water, which was used to couple the transducer and the body surface. With real-time ultrasonography, the position, size, number, shape and surrounding tissues of the lesion were targeted. The lesion was divided into sections with 5 mm of separation, and ultrasound focus was positioned at deepest layer of a section containing the maximum area of the tumor. Distances from the focal region to the hepatic capsule and to the abdominal wall on that section were measured as well. Before HIFU treatment, each patient in SonoVue group received a single intravenous injection of 10 mg SonoVue (Bracco, Italy, 25 mg of sulfur hexafluoride microbubble mixed with 5 ml of normal saline). Then HIFU sonication was started when target lesion was enriched by SonoVue microbubbles (with an average time of 22.2 ± 6.7 s). The target region was completely ablated from the deepest to superficial, and this process was repeated at every section until the tumor volume was fully ablated. During the process, the spot scan was adopted as the main sonication method, while the line scan was used as supplementary. The target region was exposed with power starting from 200 W that increased stepwise to 400 W in steps of 100 W. If massive gray-scale changes (MGSCs) could be achieved, the power was not further increased. Sonication of 1 or 2 s separated by 3 s were used. When the acoustic pathway was blocked by ribs, the respiratory control [Citation12] was then undertaken. The procedure was turning the respirator off and squeezing the breathing bag to keep the sustained pulmonary inflation for 1–2 min. Sign of an effective HIFU sonication was that MGSCs appeared or the gray-scale had a significant increase in the whole target region. The treatment was completed when the tumor volume was fully covered by MGSCs or the gray-scale was significantly increased in the tumor volume. The treatment mode is shown in .
Radiographic examination
For all patients, dynamic contrast-enhanced MRI was used to evaluate the change of tumor lesions within a month before and after overall treatment. The length (D1), thickness (D2) and width (D3) of tumor-like lesion were preoperatively measured on MRI images; correspondingly, the length (d1), thickness (d2) and width (d3) of the non-perfused region were postoperatively measured. The tumor volume was calculated by V1 = (1/6) × π × D1 × D2 × D3 [Citation13,Citation14], the volume of non-perfused volume (NPV) was calculated by V2 = (1/6) × π × d1 × d2 × d3, and the ablation rate (%) = V2/V1 × 100%. The blood supply of lesions was evaluated by preoperative MRI images, and the proposed method is detailed in the following. Firstly, the section containing a maximum area of tumor was selected. Secondly, according to the ratio of arterial enhancement in this section, the blood supply was divided into four levels as follows: (1) level-0: the ratio of enhanced area was <25%, meaning that the lesion was not substantially enhanced or only annularly strengthened,; (2) level-I: the ratio of enhanced area ranged from 25% to 50%, demonstrating that the lesion was not massively enhanced; (3) level-II: the ratio of enhanced area ranged from 50% to 90%, meaning that the lesion was inhomogeneously enhanced with hypointensity or isodensity signal on the enhanced background; (4) level-III: the ratio of enhanced area was more than 90%, showing that the lesion was homogeneously enhanced. In this study, level-0 and level-I of blood supply were designated for lesions with hypovascular, while other levels were designed to lesions with hypervascular. The rates of iodized oil deposition after TACE were estimated by abdominal computed tomography (CT) as follows: the section containing a maximum lesion was selected on CT images, and the iodized oil was deposited >50%, which was designated for the good-embolization group, and lower than 50% was designated for the poor-embolization group. The lesions’ coagulation necrosis exhibited hypointense or slightly hyperintense on T2-weighted image (T2WI), slightly hypointense or hyperintense on T1-weighted image (T1WI), and invariant hyperintensity after fat suppression on the T1WI in the target area. Meanwhile, there was no enhancement or those just annularly strengthened along with the edge of lesions for the arterial phase, portal vein phase, and late phase on the enhanced MRI images [Citation15].
Data collection
The efficacy was evaluated according to the World Health Organization (WHO)’s standard and is herein outlined as follows [Citation16]: (1) complete response (CR): tumor was completely ablated; (2) partial response (PR): tumor ablation rate ≥ 50%; (3) moderate response (MR): 25% ≤ tumor ablation rate <50%; (4) stable disease (SD): tumor ablation rate < 25%; (5) progressive disease (PD): tumor enlargement or new lesions occur. The rate of clinical efficacy = (cases with CR + cases with PR)/all cases (tumor ablation rate ≥ 50%). The HIFU treatment parameters, such as average sonication power, HIFU sonication time, treatment time, duration of general anesthesia, total energy, energy efficiency factor (EEF), occurrence rate of MGSCs, time and energy required for the first MGSCs, ‘diagnosis and treatment cycle’ and decrease of positive AFP values, were compared between SonoVue group and TACE group. Additionally, in this study, we defined ‘diagnosis and treatment cycle’ for a period starting from conducting the treatment plan to the patients being discharged. The patients were followed-up at 3, 6, 9, 12, 18, 24, 30 and 33 months after the operation. The median survival time and accumulative survival rates of 6, 12, 18 and 24 months were compared between the two groups as well. Patients were followed-up by telephone after being discharged and follow-up was ended at the time of death or at the last follow-up visit, whichever was later.
For safety, ALT, AST and creatinine were postoperatively checked within a week in both groups. Adverse events, such as soft-tissue edema, pain in treated region, fever, transitional elevation of serum transaminase, hepatorrhexis, gallbladder perforation, intestinal perforation, skin’s severe burns and other serious complications, were monitored in each patient by the same observer.
The EEF, indicating the energy required for tissue ablation per unit volume, is considered as the quantitative index of energy delivery. EEF = η · Pt/V (J/mm3). η is focus coefficient (= 0.7); P is sonication power (W); t is HIFU sonication time (s); V is NPV (mm3) [Citation17]. The correlation between EEF and influencing factors, including distance from the deepest layer of the lesion to the hepatic capsule, distance from the deepest layer of the lesion to the abdominal wall, blood supply and iodized oil deposition, was analyzed by statistical methods.
Statistical analysis
Statistical analysis was performed by using SPSS 20.0 software (IBM, Armonk, NY, USA). Normal distribution was performed by Kolmogorov-Smirnov test. Measurement data matched to the normal distribution were presented as the mean ± standard deviation (SD), otherwise, they were reported as the median. Differences between two groups were compared with t-test for the normally distributed data, non-parametric test for the non-normal distribution data, and χ2 test or Fisher’s exact test for the categorical data. The median survival time and the accumulative survival rates were estimated by Kaplan–Meier method. The comparisons of accumulative survival rates between two groups were performed using the Log-rank test. Spearman’s rank correlation was used for conducting the correlation analysis. p values <.05 was statistically considered significant.
Results
Baseline characteristics
The baseline characteristics of patients and lesions in the two groups are shown in . The data were similar between two groups (p > .05), except for that distance from the deepest layer of the lesion to the abdominal wall in the SonoVue group was greater than that in TACE group [(68.2 ± 17.8) mm vs. (58.6 ± 16.8) mm, p < .05].
Table 1. Baseline characteristics of patients and lesions for the two groups.
Tumor response and treatment parameters
The characteristics of tumor response and treatment parameters in the two groups are summarized in . The MRI images showed that different tumor responses in the SonoVue group were 15 (44.1%) for CR, 14 (41.2%) for PR, 5 (14.7%) for MR, 0 (0.0%) for SD and 0 (0.0%) for PD; correspondingly, for the TACE group, the values were 12 (30.8%) for CR, 20 (51.3%) for PR, 3 (7.7%) for MR, 4 (10.2%) for SD and 0 (0.0%) for PD. The rate of clinical efficacy (tumor ablation ratio ≥ 50%) was 85.29% in SonoVue group, and no significant difference was found with TACE group (82.05%, p > .05). In addition, the aforementioned treatment parameters were similar in both groups (p > .05). Meanwhile, the time and energy required for the first MGSCs in the SonoVue group were significantly lower than those in TACE group (p < .05). For the SonoVue group, the positive AFP values after HIFU ablation had a significant decrease compared with positive values before operation (p < .05). In contrast, there was no significant difference in the change of positive AFP values before and after the TACE + HIFU administration (p > .05). The ‘diagnosis and treatment cycle’ in the SonoVue group was significantly shorter than that in TACE group [(11.5 ± 2.9) d vs. (22.7 ± 6.3) d, p < .05]. The lesion’s changes on enhanced MRI images are shown in and .
Figure 2. A 52-year-old male patient with HCC (lesion size: 17.97 × 17.19 × 16.41 mm) in the SonoVue group. By comparing the enhanced MRI images, the target region of lesion was coagulation necrosis and the tumor was completely ablated. (a) Before HIFU sonication, the lesion exhibited slightly hyperintense on the T2WI; (b) After HIFU sonication, the slightly hyperintense of lesion turned into hypointense on the T2WI; (c) The lesion was not enhanced on the T1WI in arterial phase before treatment; (d) The lesion was not enhanced on the T1WI in arterial phase after treatment; (e) An obvious enhancement of lesion was exhibited on the T1WI in the portal vein phase before treatment; (f) The postoperative lesion without enhancement showed a dark space on the T1WI in the portal vein phase.
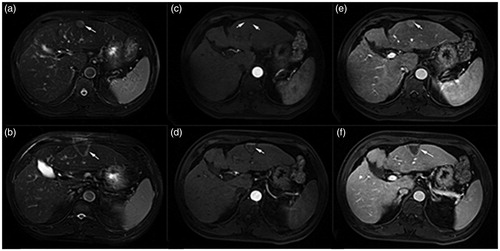
Figure 3. A 70-year-old male patient with HCC in the TACE group. The tumor was lived in right lobe near the diaphragmatic dome (lesion size: 47.81 × 50.00 × 41.25 mm). According to the enhanced MRI images, the therapeutic region of lesion was coagulation necrosis and the tumor was completely ablated compared with preoperative images. (a) Before TACE, the lesion was exhibited hyperintensity on the T2WI; (b) After HIFU sonication, the signal of lesion had a decrease and was exhibited slightly hyperintense on the T2WI; (c) The lesion was homogeneous which enhanced on the T1WI in arterial phase before treatment by TACE; (d) After HIFU sonication, the enhanced lesion had disappeared on the T1WI in the arterial phase after treatment; (e) An obvious enhancement of lesion was exhibited preoperatively on the T1WI in the portal vein phase; (f) The lesion was not enhanced on the T1WI in the portal vein phase after ablation.
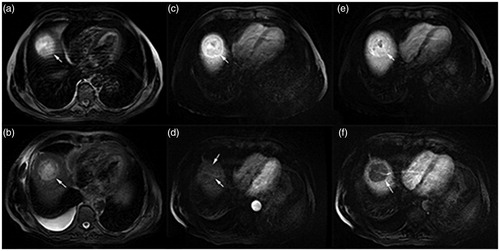
Table 2. Tumor response and treatment parameters for the two groups.
Adverse events
All patients (26 in each group) successfully completed the treatment procedure. There was no significant difference in ALT, AST and creatinine before and after the treatment (p > .05). The major adverse events were soft-tissue edema (17 vs. 20), pain in the treated region (9 vs. 14), fever (1 vs. 13) and transitional elevation of serum transaminase (3 vs. 6). The incidence rates of adverse events between two groups were similar (p > .05), except for that the rate of fever in the SonoVue group was significantly lower than that in TACE group (1 vs. 13, p < .05). In SonoVue group, there were two cases with orange peel-like change on the skin of the treatment area and the size was about 1.0 cm. All the complications were relieved by symptomatic treatment. In addition, no serious complications, such as hepatorrhexis, gallbladder perforation, intestinal perforation and skin’s severe burns were observed, when the patients were discharged.
Survival
The duration of follow-up ranged from 3 to 33 months and the average duration of follow-up was 12.5 and 17.6 months for the SonoVue group and the TACE group, respectively. During the follow-up period, 2 (7.69%) patients were lost and 3 (11.54%) patients died in SonoVue group, while 1 (3.85%) case was lost and 2 (7.69%) cases died in TACE group. The median survival time for the SonoVue group and TACE group was 30 and 33 months, respectively. The accumulative survival rates at 6, 12, 18 and 24 months for the SonoVue group were 100%, 89.4%, 89.4% and 89.4%, respectively; correspondingly, for the TACE group, those rates were 100%, 95.2%, 89.3% and 89.3%, respectively. Differences of the median survival time and accumulative survival rates were not statistically significant (p > .05), as detailed in . The survival curves for the two mentioned groups are shown in .
Figure 4. The accumulative survival curves, calculated with the Kaplan–Meier method, for patients treated with TACE + HIFU (solid line, TACE group, n = 26) and SonoVue + HIFU (dotted line, SonoVue group, n = 26). The median survival time was 30 months in the SonoVue group and 33 months in the TACE group. Neither accumulative survival rates nor median survival time were statistically significant in these two groups (p > .05).
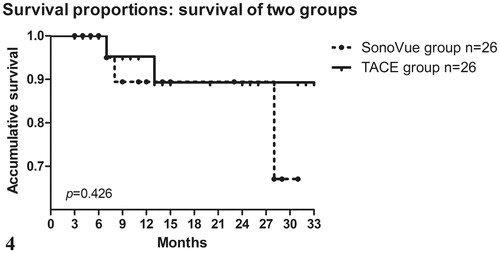
Table 3. The median survival time and the accumulative survival rates for the two groups.
Factors influencing factors of energy delivery
There was no significant difference in EEF among different levels of blood supply, either for SonoVue group or TACE group (p > .05, ). In addition, there was no linear correlation between blood supply and the EEF (p > .05).
Table 4. EEF in different blood supply levels for the two groups.
The EEF for the SonoVue group showed a linear positive correlation with distance from the deepest layer of the lesion to the hepatic capsule and from the deepest layer of the lesion to the abdominal wall (p < .05, ). However, for the TACE group, there was no linear correlation between the distance and the EEF (p > .05, ).
Figure 5. (a) EEF in the SonoVue group showed a linear positive correlation with the distance from the deepest layer of lesion to the hepatic capsule (r = 0.391, p = .022), as well as distance from the deepest layer of lesion to the abdominal wall (r = 0.349, p = .043). (b) EEF in the TACE group had no correlation with the distance from the deepest layer of lesion to the hepatic capsule (r = 0.141, p = .392), as well as distance from the deepest layer of lesion to the abdominal wall (r = –0.011, p = .946).
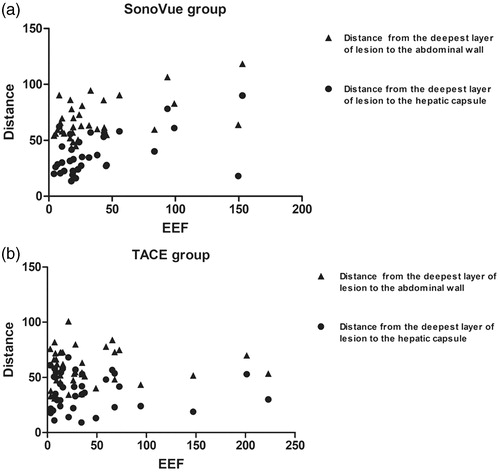
For the TACE group, EEF in good-embolization group was significantly smaller than that in poor-embolization group (p < .05, ). There was a linear negative correlation between iodized oil deposition and EEF (p < .01, ). The more iodized oil deposition in the lesion, the smaller EEF was required for ablation.
Figure 6. A linear correlation between EEF and iodized oil deposition in the TACE group (r = 0.553, p < .001). (1) Good-embolization group. (2) Poor-embolization group.
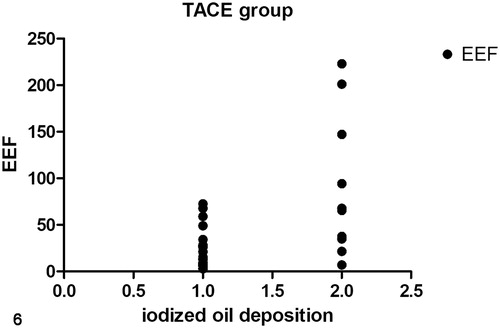
Table 5. EEF in different iodized oil deposition lesions for TACE group.
Discussion
As mentioned above, for lesions in deep location or with hypervascular supply, we need a considerable amount of energy to ablate, and how to improve the therapeutic effect of HIFU is a challenge. Several studies have focused on changing the acoustic environment and showed promising outcomes by introducing high-acoustic impedance substances (iodized oil) [Citation18], changing acoustic properties of target region (ethanol injection) [Citation19], changing blood supply of target region (TACE) [Citation20], and introducing cavitation nuclei to enhance HIFU cavitation (microbubble contrast agent) [Citation21]. Previous studies proved that the TACE + HIFU combination therapy was much more effective and had a superior overall survival rate than treatment only by HIFU or TACE for patients with HCC [Citation22,Citation23]. In addition, another study reported that combined minimally invasive therapy was much more effective than a single therapy that can certainly improve the long-term survival of patients, and among them, HIFU combined with TACE might be a more effective approach [Citation24]. For lesions, iodized oil deposition after TACE treatment can help identify the location of the lesion and decrease blood supply to reduce the ‘blood cooling’ effect. Simultaneously, it can change the acoustic environment of the liver, rise the sound absorption coefficient, and increase the energy deposition, which enhances the effect of HIFU ablation. Similarly, as an approach to change the acoustic environment, when microbubble contrast agents enter the lesion, they help the surgeon to determine the size, position, blood supply, and surrounding tissue, thus reducing the off-target rate. Meanwhile, they can result in acoustic impedance mismatching of the target region, strengthening the ultrasonic scattering and reflectivity and increasing energy deposition. The number of cavitation nuclei in the target region is also increased by exogenous microbubbles, thereby reducing the cavitation threshold and enhancing the cavitation effect [Citation25–27]. In addition, microbubbles enhance the mechanical effects of HIFU, destroy the histiocytic ultrastructure of cells, and cause more severe damage to the target region [Citation13]. At present, multiple clinical trials for benign lesions (uterine fibroids and adenomyosis) have shown that SonoVue can enhance the ablation effect, reduce EEF, and improve the efficacy, reflecting that it is a safe and effective treatment method [Citation14,Citation28–31].
In this study, no significant difference was found in clinical efficiency, accumulative survival rate, EEF, HIFU sonication time, average sonication power, total energy, and adverse events between two groups. Based on previous studies [Citation22,Citation23,Citation26], it is revealed that combination of HIFU with SonoVue or TACE is a safe and effective method to treat patients with HCC, and is more effective than HIFU or TACE alone. Using SonoVue in HIFU therapy can reduce the EEF and do not increase the risk of adverse events as well. On the procedure of HIFU, we found that the energy and time required for the first MGSCs in the SonoVue group were significantly smaller than those in the TACE group. It might be related to the enhancement of cavitation and thermal effects on the microbubbles [Citation14,Citation21,Citation31]. However, there were no significant differences in the average sonication power, total energy, HIFU sonication time, and EEF in the two groups. This may be due to the characteristic of SonoVue. SonoVue is a phospholipid-shell ultrasound contrast agent containing sulfur hexafluoride gas. Within 2 min after bolus intravenous injection, 80% of sulfur hexafluoride gas is exhaled through lungs, and almost all the gas exhaled within 15 min [Citation31]. We found the median treatment time of SonoVue group was 50 (15–120) min, far beyond SonoVue’s metabolic time in the human body. Therefore, there should be more SonoVue microbubbles in the lesion at the beginning of the HIFU procedure than that end of the procedure. As the HIFU treatment mode was from the deepest layer to the superficial layer of lesion. When we treated the deepest layer of lesion, there would be more SonoVue microbubbles. Therefore, the microbubble-related cavitation could fast ablation compared with the TACE group. During treatment in superficial layer, the number of microbubbles was decreased by metabolism and treatment loss with the extension of treatment duration. Thus, the synergistic effect for HIFU was weakened. Based on the achieved findings, we believe that if there were smaller lesions of HCC, the HIFU ablation could be completed with a short procedure, SonoVue microbubbles will have a potential better synergistic effect in treatment by HIFU.
In this study, no serious complication was observed, and no significant difference of adverse events associated with HIFU was found between the SonoVue group and the TACE group. To generate cavitation effects, it is necessary that adequate cavitation nuclei and sufficient level of energy above the cavitation threshold should exist in the acoustic field. The levels of energy are non-uniform in the HIFU acoustic fields. There is an extremely high energy level in the focal region than those in the near- or far-fields. Therefore, the cavitation effect initially happens in the focal region during the HIFU sonication. At the same time, there might be insufficient energy above the cavitation threshold in the near- and far-fields [Citation31]. This reason may demonstrate that the target region could be ablated without increasing intra- and post-procedure adverse events. Two cases of the SonoVue group occurred orange peel-like change on the skin of the treatment area. According to those two patients’ characteristics (), we found that they both had pycnic with narrow intercostal spaces. The lesions located close to the hepatic capsule and the distance from the deepest layer of the lesion to the abdominal wall was only 4–5 cm. Complementary, the incidence of fever in the TACE group was significantly higher than that in SonoVue group and it always occurred in a form of low-grade fever 1–3 days after TACE. The reason may be related to the side effects of chemotherapy drugs and the absorption of tumor necrosis.
Table 6. Characteristics of the patients with orange peel-like change.
On the ‘diagnosis and treatment cycle’, the SonoVue group was remarkably shorter than that in the TACE group [(11.5 ± 2.9) d vs. (22.7 ± 6.3) d]. It may ascribe to the adverse effects such as fever, pain in the target area and elevation of serum transaminase after undergoing TACE. Besides, CT examination is performed to determine the therapeutic effect of TACE and make the treatment plan of HIFU. Eventually, those above-mentioned effects extend the patient's overall ‘diagnosis and treatment cycle’.
Regarding EEF, previous study demonstrated that the EEF negatively correlated with tumor diameter [Citation17]. In the present study, tumor diameter was not included, attributing to the narrow range (d ≤ 5 cm). As mentioned above [Citation6], the energy delivery was related to blood supply. However, there was no clear correlation between blood supply and EEF in the SonoVue group or in the TACE group, which might be related to the small sample size and change of blood supply after TACE. ter Haar et al. [Citation32] found that the ultrasonic radiation energy was decreased with the depth of irradiation under the same conditions in vitro. Wang et al. [Citation33] also demonstrated that the US energy deposition was related to the irradiation depth. Therefore, as the depth of the target area increases, the ultrasonic energy continues to attenuate. We found EEF of the SonoVue group had a linear positive correlation with distance from the deepest layer of the lesion to the abdominal wall, and distance from the deepest layer of the lesion to the hepatic capsule, which was consistent with previous studies. However, this conclusion was not confirmed in the TACE group. It may be related to the deposition or distribution of iodized oil and chemotherapy drugs, and the small sample size. A significant difference in EEF was calculated between lesions with different iodized oil deposition. The EEF of poor-embolization group was significantly higher than that of good-embolization group. There was a linear negative correlation between EEF and iodized oil deposition. Thus, the more iodized oil deposition in lesions, the smaller the EEF is required for ablation. Iodized oil is a high-impedance material that can ‘intercept’ the ultrasound and cause a reflection on its interface, thereby inducing high temperature [Citation18]. However, the deposition and distribution of iodized oil in the lesions are related to the intraoperative dose, the choice of embolization vessels, tumor size, and collateral circulation formation [Citation34–36]. Therefore, the uncontrollability of ablation dose increases in the TACE group, which is not only related to the size of lesions, the blood supply, and the distance but also closely associated with the factors affecting iodized oil. Comparatively, the interference factors on ablation dose have not been increased in the SonoVue group.
It should be clearly mentioned that the present study was a retrospectively non-randomized analysis and was limited by the small sample size, in which only 26 patients were assigned to each group. Secondly, the AFP value was checked within a month after HIFU treatment, which may not reach the period of AFP decline, thus, the AFP values for some patients have not significantly decreased as expected. Eventually, the longest duration of follow-up was only 33 months, and no data regarding more long-term follow-up was provided. In summary, we suggest that more cases and prospective randomized-controlled trials should be provided to verify the achieved results.
In conclusion, the proposed is safe and effective utilizing SonoVue injection in HIFU procedure. Similar to TACE, SonoVue can improve the efficacy of HIFU ablation as well, by changing the acoustic environment in patients with HCCs ≤5 cm, and does not increase the incidence of adverse events. Moreover, SonoVue does not increase the interference factors that affect the EEF, and it can short the ‘diagnosis and treatment cycle’. Therefore, it might present a new strategy in clinical treatment, especially in patients with the smaller diameter of HCC.
Disclosure statement
The authors confirm that there is no conflict of interest regarding the publication of this article.
Additional information
Funding
References
- Are C, Meyer B, Stack A, et al. Global trends in the burden of liver cancer. J Surg Oncol. 2017;115:591–602.
- Kudo M. Surveillance, diagnosis, treatment, and outcome of liver cancer in Japan. Liver Cancer. 2015;4:39–50.
- Chen X, Liu HP, Li M, et al. Advances in non-surgical management of primary liver cancer. World J Gastroenterol. 2014;20:16630–16638.
- Padhya KT, Marrero JA, Singal AG. Recent advances in the treatment of hepatocellular carcinoma. Curr Opin Gastroenterol. 2013;29:285–292.
- Yu H, Burke CT. Comparison of percutaneous ablation technologies in the treatment of malignant liver tumors. Semin Intervent Radiol. 2014;31:129–137.
- Kennedy JE. High-intensity focused ultrasound in the treatment of solid tumours. Nat Rev Cancer. 2005;5:321–327.
- Liu L, Xiao Z, Xiao Y, et al. Combination of high-intensity focused ultrasound irradiation and hydroxyapatite nanoparticle injection to injure normal goat liver tissue in vivo without costal bone incision. Genet Mol Res. 2014;13:8301–8308.
- Ministry of Health of the People’s Republic of China. Standard Specification for diagnosis and treatment of primary Liver cancer (2011). J Clin Oncol. 2011;16:929–946. [Article in Chinese].
- Wu F, Chen WZ, Bai J, et al. Pathological changes in human malignant carcinoma treated with high-intensity focused ultrasound. Ultrasound Med Biol. 2001;27:1099–10106.
- Wu F, Wang ZB, Chen WZ, et al. Advanced hepatocellular carcinoma: treatment with high-intensity focused ultrasound ablation combined with transcatheter arterial embolization. Radiology. 2005;235:659–667.
- Jung SE, Cho SH, Jang JH, et al. High-intensity focused ultrasound ablation in hepatic and pancreatic cancer: complications. Abdom Imaging. 2011;36:185–195.
- Zhang L, Zhu H, Jin C, et al. High intensity focused ultrasound (HIFU): effective and safe therapy for hepatocellular carcinoma adjacent to major hepatic veins. Eur Radiol. 2009;19:437–445.
- Luo W, Zhou X, Ren X, et al. Enhancing effects of SonoVue, a microbubble sonographic contrast agent, on high-intensity focused ultrasound ablation in rabbit livers in vivo. J Ultrasound Med. 2007;26:469–476.
- Chen Y, Jiang J, Zeng Y, et al. Effects of a microbubble ultrasound contrast agent on high-intensity focused ultrasound for uterine fibroids: a randomised controlled trial. Int J Hyperthermia. 2018;34:1311–1315.
- Chinese Medical Association. Clinical application guide for high-intensity focused ultrasound tumor therapy system (trial). Natl Chin J Med. 2005;85:796–798. [Article in Chinese].
- Cheung TT, Fan ST, Chan SC, et al. High-intensity focused ultrasound ablation: an effective bridging therapy for hepatocellular carcinoma patients. World J Gastroenterol. 2013;19:3083–3089.
- Liu Z, Gong C, Liu Y, et al. Establishment of a scoring system for predicting the difficulty level of high-intensity focussed ultrasound ablation of uterine fibroids. Int J Hyperthermia. 2018;34:77–86.
- Cheng SQ, Zhou XD, Tang ZY, et al. Iodized oil enhances the thermal effect of high-intensity focused ultrasound on ablating experimental liver cancer. J Cancer Res Clin Oncol. 1997;123:639–644.
- Chen C, Liu Y, Maruvada S, et al. Effect of ethanol injection on cavitation and heating of tissues exposed to high-intensity focused ultrasound. Phys Med Biol. 2012;57:937–961.
- Wang S, Yang C, Zhang J, et al. First experience of high-intensity focused ultrasound combined with transcatheter arterial embolization as local control for hepatoblastoma. Hepatology. 2014;59:170–177.
- Jiang N, Xie B, Zhang X, et al. Enhancing ablation effects of a microbubble-enhancing contrast agent (“SonoVue”) in the treatment of uterine fibroids with high-intensity focused ultrasound: a randomized controlled trial. Cardiovasc Intervent Radiol. 2014;37:1321–1328.
- Kim J, Chung DJ, Jung SE, et al. Therapeutic effect of high-intensity focused ultrasound combined with transarterial chemoembolisation for hepatocellular carcinoma <5 cm: comparison with transarterial chemoembolisation monotherapy—preliminary observations. Br J Radiol. 2012;85:940–946.
- Wu Y, Li J, Zhang SJ, et al. [Clinical observation on high intensity focused ultrasound combined with transcatheter arterial chemoembolization in the treatment of hepatocellular carcinoma.]. Zhonghua Wai Ke Za Zhi. 2012;50:691–694. [Article in Chinese].
- Zhao J, Zhang H, Wei L, et al. Comparing the long-term efficacy of standard and combined minimally invasive procedures for unresectable HCC: a mixed treatment comparison. Oncotarget. 2017;8:15101–15113.
- Chung DJ, Cho SH, Lee JM, et al. Effect of microbubble contrast agent during high intensity focused ultrasound ablation on rabbit liver in vivo. Eur J Radiol. 2012;81:519–523.
- Kopechek JA, Park EJ, Zhang YZ, et al. Cavitation-enhanced MR-guided focused ultrasound ablation of rabbit tumors in vivo using phase shift nanoemulsions. Phys Med Biol. 2014;59:3465–3481.
- Leon-Villapalos J, Kaniorou-Larai M, Dziewulski P. Full thickness abdominal burn following magnetic resonance guided focused ultrasound therapy. Burns. 2005;31:1054–1055.
- Peng S, Hu L, Chen W, et al. Intraprocedure contrast enhanced ultrasound: The value in assessing the effect of ultrasound-guided high intensity focused ultrasound ablation for uterine fibroids. Ultrasonics. 2015;58:123–128.
- Orsi F, Monfardini L, Bonomo G, et al. Ultrasound guided high intensity focused ultrasound (USgHIFU) ablation for uterine fibroids: Do we need the microbubbles? Int J Hyperthermia. 2015;31:233–239.
- Cheng CQ, Zhang RT, Xiong Y, et al. Contrast-enhanced ultrasound for evaluation of high-intensity focused ultrasound treatment of benign uterine diseases: retrospective analysis of contrast safety. Medicine (Baltimore). 2015;94:729.
- Jingqi W, Lu Z, Jun Z, et al. Clinical usefulness of the microbubble contrast agent SonoVue in enhancing the effects of high-intensity focused ultrasound for the treatment of adenomyosis. J Ultrasound Med. 2018;37(12):2811–2819.
- ter Haar G, Sinnett D, Rivens I. High intensity focused ultrasound-a surgical technique for the treatment of discrete liver tumours. Phys Med Biol. 1989;34:1743–1750.
- Wang Z, Bai J, Li F, et al. Study of a "biological focal region" of high-intensity focused ultrasound. Ultrasound Med Biol. 2003;29:749–754.
- Chen MS, Li JQ, Zhang YQ, et al. High-dose iodized oil transcatheter arterial chemoembolization for patients with large hepatocellular carcinoma. World J Gastroenterol. 2002;8:74–78.
- Miyayama S, Matsui O, Yamashiro M, et al. Iodized oil accumulation in the hypovascular tumor portion of early-stage hepatocellular carcinoma after ultraselective transcatheter arterial chemoembolization. Hepatol Int. 2007;1:451–459.
- Chen CS, Li FK, Guo CY, et al. Tumor vascularity and lipiodol deposition as early radiological markers for predicting risk of disease progression in patients with unresectable hepatocellular carcinoma after transarterial chemoembolization. Oncotarget. 2016;7:7241–7252.