Abstract
Purpose: We previously developed a novel cryo-thermal therapy to treat malignant mammary carcinoma and melanoma in a mouse model; long-term survival and CD4+ T cell orchestrating anti-tumor immune memory response were achieved. Moreover, cryo-thermal-induced CD4+ T cell differentiation into Th1 and CD4+CTL sub-lineages, in which M1 macrophage polarization played a direct, important role. In particular, cryo-thermal therapy triggered M1 macrophage polarization with up-regulated expression of C–X–C motif ligand 10 (CXCL10) and Interleukin 6 (IL-6). But whether CXCL10 and IL-6 contribute to CD4+ T cell-mediated anti-tumor immunity remains unclear. In this study, the role of cryo-thermal-induced CXCL10 and IL-6 in anti-tumor immunity was determined.
Methods: The level of CXCL10 and IL-6 in spleen and serum was determined by RT-PCR and ELISA on day 14 after cryo-thermal therapy. Splenic dendritic cells (DCs) and macrophages were isolated from cryo-thermal-treated mice on day 5 and 14, and the level of CXCL10 and IL-6 in macrophages and DCs was determined by ELISA. The transwell migration assay was performed to study immune cell migration. In vivo neutralization of CXCL10 or IL-6 was performed to investigate the phenotypic changes of immune cells.
Results: Cryo-thermal therapy induced M1 macrophage polarization with up-regulation of CXCL10 and IL-6 expression in spleen. CXCL10 and IL-6 promoted DCs migration and maturation, and subsequently promoted CD4+ T cell migration and differentiation into Th1 and CD4+ CTL, moreover, reduced myeloid-derived suppressor cells (MDSCs) accumulation.
Conclusions: Cryo-thermal-induced CXCL10 and IL-6 created acute inflammatory environment to initiate a systemically cascading innate and adaptive anti-tumor immunity, which was more permissive for tumor eradication.
Introduction
In recent years, researchers learn more about how the body fights cancer by its own, and anti-tumor immunotherapy has been developed. Others have shown that the anti-tumor effect requires robust inflammation [Citation1–3].
Acute inflammation caused by physical, chemical injury or an infectious agent can initiate an inflammatory cascade to help activation of innate and adaptive immunity [Citation4], and skew the immune response toward Th1 type immunity, which is a characteristic of immune cell-mediated cytotoxic killing [Citation5,Citation6]. However, chronic inflammation can create immunosuppressive environment to diminish the capacity of immune effector cells to recognize and kill tumor cells leading to tumor progression and metastasis [Citation4,Citation7].
Interleukin 6 (IL-6) has a dual effect in chronic and acute inflammation [Citation8–10]. The activity of IL-6 is thought to be immunosuppression or tumor-promoting by supporting tumor evasion of immune surveillance, however, recent findings indicate that IL-6 participates in regulating acute inflammatory responses and restoring T cell immunity [Citation10–12], thus playing an important role in linking innate and adaptive immune response. C–X–C motif ligand 10 (CXCL10), also known as γ-interferon-induced protein of 10 kDa (IP10), is a potent pro-inflammatory chemokine which mainly regulates immune cell migration, differentiation and activation [Citation13]. CXCL10 acts as a chemoattractant for T cells, natural killer (NK) cells, dendritic cells (DCs) and macrophages [Citation14]; it attracts Th1 cells while blocking the migration of Th2 cells [Citation15]. CXCL10 is also involved in macrophage and Th1 polarization, and promotes anti-tumor immunity by activating T cells, NK, DCs and macrophages [Citation13,Citation16,Citation17]. However, the role of CXCL10 and IL-6 in inflammation and immunity needs further be evaluated.
In our previous study, we develop a novel cryo-thermal therapy through applying local rapid cooling followed by rapid heating of tumor tissue, highlighting its effect on the suppression of distal metastasis and leading to long-term survival in 4T1 murine mammary carcinoma and murine B16 melanoma model [Citation18–24]. Cryo-thermal therapy induces CD4+ T cells to orchestrate anti-tumor immune memory response leading to long-term tumor-free survival [Citation25]. Moreover, cryo-thermal-induced M1 macrophage polarization was essential to CD4+ T cell-mediated anti-tumor immunity (submitted). As demonstrated previously, cryo-thermal therapy triggered M1 macrophage polarization, along with the marked up-regulation of CXCL10 and IL-6 expression. In addition, acute IL-6 induced by cryo-thermal therapy promotes DCs maturation and CD4+ T cell differentiation [Citation24]. In this regard, we hypothesized that cryo-thermal systematically induced CXCL10 and IL-6 expression to create a favorable environment for driving immune clearance of tumor cells by modulating inflammation and anti-tumor immunity. In this study, we further studied the role of CXCL10 and IL-6 in cryo-thermal-triggered anti-tumor immune response. Levels of CXCL10 and IL-6 in spleen and serum from cryo-thermal-treated mice bearing B16 melanoma were determined. In vivo neutralization of CXCL10 or IL-6 was performed, and phenotypic changes of immune cells were analyzed to study whether CXCL10 and IL-6 mediated M1 macrophage polarization, DCs maturation, CD4+ and CD8+ T cell differentiation. Our studies showed that cryo-thermal-induced M1 macrophage polarization with up-regulation expression of CXCL10 and IL-6. Via the robust release of CXCL10, M1 macrophages stimulated DCs migration, and then recruited CD4+ T cells through DCs indirectly. Under CXCL10 and IL-6-created acute inflammatory environment in spleen, the host immune environment was reshaped contributing to subsequent DCs maturation, CD4+ T cell differentiation into Th1 and CD4+ CTL sub-lineages. Our study suggested that the interaction of macrophages with DCs and T cells under cryo-thermal-induced CXCL10 and IL-6-rich acute inflammatory environment triggered CD4+ T cell-mediated anti-tumor immunity.
Materials and methods
Animal model
Female C57BL/6 mice were obtained from Shanghai Slaccas Experimental Animal Co., Ltd. (Shanghai, China) and used for experimental study at the age of 6–8 weeks. They were housed in isolated cages and a 12 h light/dark cycle environment, feeding with sterile food and acidified water with pH value kept at 7.5–7.8. All animal experiments were approved by the Animal Welfare Committee of Shanghai Jiao Tong University and experimental methods were performed in accordance with the guidelines of Shanghai Jiao Tong University Animal Care (approved by Shanghai Jiao Tong University Scientific Ethics Committee). Murine B16F10 cells (donated by Prof. Weihai Yin at Med-X Research Institute, Shanghai Jiao Tong University) were cultured in RPMI 1640 medium (Hyclone, Logan, UT) supplemented with 10% FBS, plus 100 U/mL penicillin, and 100 g/mL streptomycin (Shanghai Sangon, Shanghai, China). To prepare the tumor-bearing mice, approximately 5 × 105 cells were injected subcutaneously into the right femoral region of each mouse. Tumor sizes were measured every 2–3 days and its volume were estimated using the following formula: V (cm3) = π × L (major axis) × W (minor axis) × H (vertical axis)/6.
The thermal therapy procedures
The system developed in our laboratory was composed of liquid nitrogen for cooling and radiofrequency (RF) for heating [Citation26]. To reduce the effect of contact thermal resistance and obtain a constant thermal delivery during the treatment, a probe was designed with a cylinder-shaped tip of 10 mm in diameter for the thermal therapy of subcutaneous tumor [Citation27]. Subcutaneous injection of B16F10 melanoma cells into C57BL/6 mice leads to form primary tumor in 7–9 days and spontaneous metastasis in lungs [Citation28]. A total of 12 days after tumor inoculation, when the tumor volume reached about 0.2 cm3, mice were divided into three groups: tumor-bearing group without the treatment (control); cryo-thermal group with freezing at the temperature of −20 °C for 5 min followed by RF heating at the temperature of 50 °C (the simulated temperature distribution during the RF heating process was performed previously[Citation22]) for 10 min on primary tumor (cryo-thermal); hyperthermia with RF heating on primary tumor at the temperature of 50 °C for 15 min. The mice were anesthetized with intraperitoneal (i.p.) injection of 1.6% pentobarbital sodium (0.5 mL/100 g, Sigma-Aldrich, St. Louis, MO). The tumor site was sanitized with alcohol and iodine tincture before the treatment. All of the procedures were performed aseptically.
CXCL10 and IL-6 neutralization experiment
For CXCL10 and IL-6 neutralization experiments, 50 µg anti-CXCL10 mAb (R&D system, Minneapolis, MN) [Citation29] and 100 µg anti-IL-6 mAb (Sanjian, Tianjin, China) [Citation24] was i.p. injected 24 h before cryo-thermal therapy, respectively, then the injection was repeated on day 5 and 10 after cryo-thermal therapy. Mice were also given an isotype IgG mAb at the same time. The untreated tumor-bearing mice were used as the control. The immune cells in spleen were analyzed by using flow cytometry and qRT-PCR.
Preparation of a single-cell suspension and FACS analysis
Mice were sacrificed on day 5 and 14 after cryo-thermal therapy, and spleen was collected (n = 4 per group at each time point). Single-cell suspension of splenocytes was prepared using GentleMACS™ dissociator (Miltenyi Biotec, Bergisch Gladbach, Germany), and then treated with erythrocyte-lysing reagent containing 0.15 M NH4Cl, 1.0 M KHCO3 and 0.1 mM Na2EDTA to remove red blood cells. Staining antibodies including CD11b-FITC (clone M1/70), Gr-1-PE (clone RB6-8C5), CD3-FITC (clone 145-2C11), CD4-APC (clone GK1.5), CD8-APC (clone 53–6.7), F4/80-APC (clone BM8), CD86-PE (clone GL-1), IA-IE-percp-cy5.5 (clone M5/114.15.2) and CD11c-FITC (clone N418) were purchased from Biolegend (San Diego, CA). For cell surface staining, splenocytes were stained with antibodies as described above for 30 min at 4 °C. The cells were analyzed on a FACS Aria II cytometer (BD Biosciences, Franklin Lakes, NJ) and the data were analyzed using FlowJo software (FlowJo, LLC, Ashland, OR).
Isolation of CD68+macrophages, CD11c+ DCs, CD4+ and CD8+ T cells
For isolation of T cells, spleens from the treated and tumor-bearing C57BL/6 mice were harvested on day 14 after the treatment (n = 4 mice per group), and splenocytes were prepared using GentleMACS™ dissociator (Miltenyi Biotec, Bergisch Gladbach, Germany) and passed through a 40-μm nylon filter. CD4+ and CD8+ T cells were purified from splenocytes using Easysep mouse CD4+ and CD8+ T cell Enrichment Kits (StemCell Technologies, Vancouver, Canada) according to the manufacturer’s instructions. CD4+ and CD8+ T cells with a purity of > 90% were used for experiments. For isolation of DCs and macrophages, spleens from the treated and tumor-bearing C57BL/6 mice were harvested on day 5 and 14 after the treatment (n = 4 mice per group at each time point), respectively, and splenocytes were prepared using GentleMACS™ dissociator (Miltenyi Biotec, Bergisch Gladbach, Germany) and passed through a 40-μm nylon filter. DCs were isolated from splenocytes by using DC isolation micro-bead kit (EasysepTM CD11c positive selection kit, StemCell Technologies, Vancouver, Canada) according to the manufacturer’s instructions. For CD68+ macrophage isolation, splenocytes were plated in DMEM supplemented with 10% FBS at 37 °C in a humidified 5% CO2 incubator for 1 h, then the supernatant fraction was poured off and the adherent splenocyte fraction was processed for CD68+ macrophage isolation by using EasySepTM PE positive selection kit (StemCell Technologies, Vancouver, Canada) according to the manufacturer’s instructions.
Migration assay
Transwell migration assay was performed by using 8 μm pore size transwell chambers (Costar Corporation, Greenwich, CT). 2.5 × 105 splenic CD68+ macrophages isolated from the cryo-thermal treated mice on day 14 after the treatment were seeded in the lower chamber with DMEM (10% FBS). 2.5 × 105 splenic myeloid-derived suppressor cells (MDSC), DCs, CD4+ T cells or CD8+ T cells from tumor-bearing mice on day 26 after tumor inoculation were added in the upper chamber with DMEM (0.2% BSA), respectively. To verify whether macrophage-derived CXCL10 could recruit MDSC, DCs, CD4+ T cells and CD8+ T cells, anti-CXCL10 mAb (20 µg/mL) was added to lower chambers. After incubation at 37 °C overnight, cells in the lower chamber were stained with corresponding surface antibodies for analyzing the migration of MDSC, DCs, CD4+ and CD8+ T cells. Each experiment was performed in triplicate. In another experiment, 2.5 × 105 splenic CD11c+ DCs isolated from the cryo-thermal treated mice on day 14 after the treatment were seeded in the lower chamber, 2.5 × 105 splenic CD4+ T cells or CD8+ T cells from tumor-bearing mice on day 26 after tumor inoculation were added in the upper chamber, respectively. Anti-CXCL10 mAb (20 µg/mL) was also added to lower chambers. After incubation at 37 °C overnight, cells in the lower chamber were stained with anti-CD3, anti-CD4 and anti-CD8 antibodies for analyzing T cell migration. Each experiment was performed in triplicate.
RNA isolation and real-time PCR
Total RNA was prepared from spleen tissues, purified CD68+macrophages, CD11c+ DCs, CD4+ and CD8+ T cells, respectively, using TRIzol Reagent (TaKaRa, Dalian, China). Absorbance at 260/280 nm for mRNA purity at a ratio above 1.9 was achieved for all samples used. cDNA was made using a PrimerScript RT reagent kit with gDNA Eraser (TaKaRa, Dalian, China). Quantitative real-time PCR (RT-PCR) was performed on ABI 7900HT sequence detection system, and SDS software (Applied Biosystems, Foster City, CA) using SYBR Premix Ex Taq (TaKaRa) and samples were amplified in 384-well plates. The mRNA in spleen tissues, splenic CD68+macrophages, CD11c+ DCs, CD4+ and CD8+ T cells from tumor-bearing mice were used as the control to compare the marker profiles of splenic CD68+macrophages, CD11c+ DCs, CD4+ and CD8+ T cells from the treated mice, expressed as a fold difference, respectively. The primer sequences of mouse genes present in Table S1. Relative expression levels of mRNA for each gene were normalized to GAPDH determined by using the Ct value and assessed using relative quantification (delta–delta Ct method). All experiments were performed in triplicates.
ELISA analysis
To evaluate the level of CXCL10 and IL-6 in spleen after cryo-thermal therapy, the spleen tissues were collected from cryo-thermal-treated mice or hyperthermia-treated mice on day 14 after the treatment, and tumor-bearing mice on day 26 after tumor inoculation, and tissue homogenate was prepared using 1 × PBS. To evaluate the level of CXCL10 and IL-6 in serum after cryo-thermal therapy mice, the peripheral blood was collected from cryo-thermal-treated mice or hyperthermia-treated on day 14 after the treatment and tumor-bearing mice on day 26 after tumor inoculation. The peripheral blood samples were centrifuged and the serum was obtained. Then the CXCL10 and IL-6 concentration in the supernatant of spleen tissue homogenate and serum were analyzed using mouse CXCL10/IP-10 ELISA kit and mouse IL-6 ELISA kit (all from Boster, Pleasanton, CA), respectively. A standard curve was established according to the manufacture’s instruction. Experimental values were computed with the use of regression analysis.
To evaluate the level of CXCL10 and IL-6 in DCs and macrophage after cryo-thermal therapy, splenic CD11c+ DCs and CD68+ macrophage were isolated from cryo-thermal-treated mice on day 5 and 14 after the treatment. The splenic CD11c+ DCs and CD68+ macrophage isolated from tumor-bearing mice on day 17 and 26 after tumor inoculation were used as control. Then the isolated CD11c+ DCs and CD68+ macrophage were lysed in RIPA lysis buffer (with cocktail proteinase inhibitor, 0.1% SDS and added PMSF to 1 mM before use) and the extracted protein was subject to for the examination of CXCL10 and IL-6 concentration using ELISA kit.
Statistical analysis
The Student’s t-test and one-way ANOVA were used for statistical comparisons using Graph Pad Prism version 6 (Graph Pad Software, La Jolla, CA). Figures denoted statistical significance of p < .05 as *, p < .01 as **, p < .001 as *** or &p < .05 or &&p < .01 or &&&p < .001. Results were expressed as mean ± SD.
Results
The cryo-thermal-induced CXCL10 and IL-6-rich acute inflammatory environment
As demonstrated previously, cryo-thermal therapy (pre-freezing at the temperature of −20 °C for 5 min followed by RF heating at the temperature of 50 °C for 10 min) is superior to hyperthermia (50 °C for 15 min) in acquiring a significant inhibition of distant lung metastasis and long-term survival in 4T1 murine mammary carcinoma and murine B16 melanoma model [Citation22,Citation23,Citation25]. Cryo-thermal therapy triggered M1 macrophage polarization and DCs phenotypic maturation, along with up-regulation of CXCL10 and IL-6 from day 5 to 14 after the treatment (submitted). To evaluate whether cryo-thermal therapy could specifically promote systemic production of CXCL10 and IL-6 as compared to hyperthermia, the mRNA and protein levels of CXCL10 and IL-6 in spleen and serum of treated mice on day 14 were determined by RT-PCR and ELISA, as compared to the untreated mice of the control group. We found that cryo-thermal therapy and hyperthermia substantially increased both mRNA and protein levels of CXCL10 in spleen on day 14 as compared to the control group (). But, circulating levels of CXCL10 in serum from mice treated by cryo-thermal therapy and hyperthermia were markedly lower (). Moreover, only cryo-thermal therapy substantially increased both mRNA and protein levels of IL-6 in spleen and serum in treated mice as compared to the control and hyperthermia groups (). These data suggested that cryo-thermal therapy created CXCL10 and IL-6-rich acute inflammatory environment, however, hyperthermia only created CXCL10-rich, but IL-6-scanty inflammatory environment in spleen.
Figure 1. The mRNA and protein levels of CXCL10 and IL-6 in spleen and serum were determined by RT-PCR and ELISA on day 14 after cryo-thermal therapy. (A and B) (spleen) and (C) (serum). The mRNA and protein levels of CXCL10. (D and E) (spleen) and (F) (serum). The mRNA and protein levels of IL-6. Data were shown as mean ± SD. Data for bar graphs were calculated using one-way ANOVA. **p < .01 and ***p < .001 was considered to be statistically significant compared with the control group, &p < .05 was considered to be statistically significant compared with cryo-thermal group.
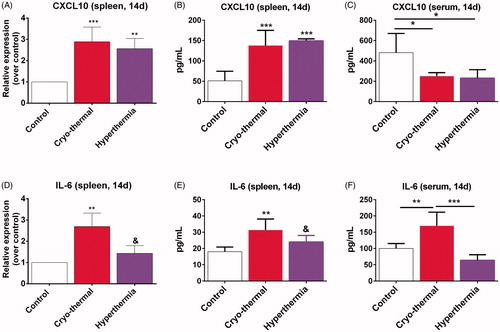
To evaluate what kind of APCs (DC and macrophage) was major source of CXCL10 and IL-6 in spleen after cryo-thermal therapy, splenic CD11c+ DCs and CD68+ macrophages were isolated from cryo-thermal-treated mice on day 5 and 14 after the treatment, and the concentrations of CXCL10 and IL-6 in macrophages and DCs were determined by ELISA, respectively. We found that the concentrations of CXCL10 and IL-6 in macrophages from cryo-thermal-treated mice were substantially increased from day 5 to 14 after the treatment, as compared to the untreated mice, whereas the concentrations of CXCL10 and IL-6 in DCs were not significantly different from day 5 to 14 (Figure S1 (A–D)). Altogether, these results indicated that strong pro-inflammatory responses with high concentrations of CXCL10 and IL-6 produced by splenic macrophages were systemically induced on day 14 after cryo-thermal therapy.
Macrophage-derived CXCL10 recruited DCs and DCs-derived CXCL10 promoted CD4+ T cells migration
CXCL10 is an inflammatory mediator that attracts activated T cells, macrophages and DCs [Citation13,Citation17,Citation30,Citation31]. The above results showed that the expression level of CXCL10 was significantly higher in splenic macrophages from cryo-thermal-treated mice from day 5 to 14 after the treatment. Furthermore, CXCL10 production was also increased in spleen of cryo-thermal-treated mice. Therefore, we supposed that CXCL10 production by macrophage could mediate immune cell trafficking in spleen. Then transwell migration assay was performed as described in Methods. We found that, when CXCL10 neutralizing antibody and isolated splenic macrophages from cryo-thermal-treated mice on day 14 were placed into the lower chamber, cell migration of MDSC, CD4+ and CD8+T cells was not obviously affected as compared to the control group (), however, DCs migration was significantly inhibited (). These results suggested that DCs were recruited by CXCL10 production by splenic macrophages after cryo-thermal therapy, but MDSC, CD4+ and CD8+ T cells could not be recruited by macrophage-derived CXCL10.
Figure 2. CXCL10 production by splenic macrophages after cryo-thermal therapy promoted DCs migration in vitro. The total migrated cells were counted in CD4+T cells (A), CD8+T cells (B), DCs (C) and MDSCs (D). All data were shown as mean ± SD. *p < .05. Data for bar graphs were calculated using Student’s t-test.
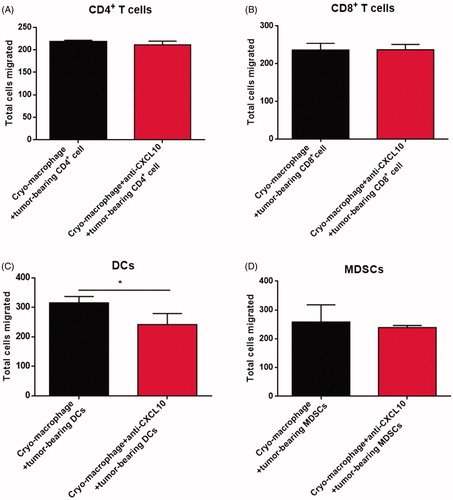
Next, we supposed whether isolated DCs from cryo-thermal-treated mice could recruit T cells. Therefore, a transwell migration assay was also performed and we found that, when CXCL10 neutralizing antibody and isolated splenic DCs from cryo-thermal-treated mice on day 14 were placed into the lower chamber, only CD4+ T cell migration was significantly inhibited (Figure S2), which suggested that CXCL10 produced by splenic DCs mediated CD4+ T cell migration after cryo-thermal therapy. Above all, through high level of CXCL10 in spleen, macrophages promoted DCs migration, and indirectly recruited CD4+ T cells via DCs after cryo-thermal therapy.
Cryo-thermal-induced CXCL10 and IL-6 medicated M1 macrophage polarization
CXCL10 can regulate macrophage polarization [Citation17]. IL-6 is an essential factor in promotion of macrophage differentiation [Citation32]. Whether cryo-thermal-induced CXCL10 and IL-6 could modulate macrophage polarization was addressed. In vivo neutralization experiments were performed, and the percentage of M1 macrophages (CD11b+F4/80+CD86+MHC II+) in spleen was increased on day 14 after cryo-thermal therapy + anti-IgG, which was much higher than that in the control group, while there was no significant difference on the percentage of splenic M1 macrophages from cryo-thermal treated mice with CXCL10 or IL-6 neutralizing antibody ().
Figure 3. CXCL10 and IL-6 induced by cryo-thermal therapy modulated M1 macrophages polarization. (A and B) On day 14 after the treatment, the splenocytes were harvested from the mice in cryo-thermal + anti-IgG, cryo-thermal + anti-CXCL10, cryo-thermal + anti-IL-6 and the control groups, then the percentage of CD11b+F4/80+CD86+MHC II+ macrophage was analyzed by flow cytometry. All data were shown as mean ± SD. *p < .05. Data for bar graphs were calculated using one-way ANOVA. (C) The mRNA expression level of proinflammatory cytokines in splenic CD68+ macrophages was measured by real-time PCR. (D) The mRNA expression level of immunosuppressive molecules in splenic CD68+ macrophages was measured by real-time PCR. Data were shown as mean ± SD. Data for bar graphs were calculated using two-way ANOVA. Each value of *p < .05 or **p < .01 or ***p < .001 was considered to be statistically significant compared with the control group, &p < .05, &p < .01 and &&&p < .001 was considered to be statistically significant compared with cryo-thermal + anti-IgG group.
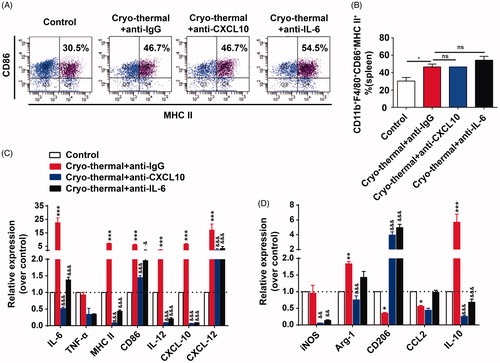
To address whether cryo-thermal-induced CXCL10 or IL-6 could change gene expression profiles of macrophages, thus, the mRNA level of several cytokines in the splenic macrophages was assessed by RT-PCR. The relative mRNA expression of co-stimulatory molecules (CD86 and MHC II), pro-inflammatory cytokines (IL-6 and IL-12) and chemokines (CXCL10 and CXCL12) in isolated macrophages from cryo-thermal-treated mice with in vivo CXCL10 neutralization was significantly down-regulated in comparison to that in the isotype IgG group (). The relative mRNA expression of co-stimulatory molecules (CD86 and MHC II), pro-inflammatory cytokines (IL-6 and IL-12) and chemokines (CXCL10 and CXCL12) in isolated macrophages from cryo-thermal-treated mice with in vivo IL-6 neutralization was also significantly down-regulated in comparison to that in the isotype IgG group (). Furthermore, the mRNA expression of M2-associated transcripts CD206 was significantly up-regulated in isolated macrophages from cryo-thermal-treated mice with in vivo CXCL10 or IL-6 neutralization. The mRNA expression of IL-10, Arg-1 and iNOS in macrophages from cryo-thermal treated mice with in vivo CXCL10 neutralization was significantly decreased as compared to isotype IgG group, whereas no significant change of CCL2 mRNA was observed. The expression of IL-10 and iNOS mRNA in macrophages from cryo-thermal treated mice with in vivo IL-6 neutralization was significantly decreased as compared to isotype IgG group, whereas no significant change of Arg-1 and CCL2 mRNA was observed (). These results indicated that cryo-thermal-induced CXCL10 or IL-6 modulated M1 macrophage polarization with up-regulated expression of pro-inflammatory cytokines and co-stimulatory molecules.
Cryo-thermal-induced CXCL10 and IL-6 medicated DCs maturation
Except that CXCL10 recruits DCs, and the elevation of CXCL10 level in DCs promotes DCs maturation and enhances anti-tumor activity [Citation30,Citation33–35]. Our previous study indicates that cryo-thermal-induced IL-6 promotes DCs phenotypic maturation [Citation24]. We investigated whether activation and maturation of splenic DCs attributed to cryo-thermal-induced CXCL10 or IL-6 production. On day 14 after the treatment, cryo-thermal therapy markedly up-regulated mRNA expression level of CD86 and MHC II on splenic DCs compared with that in the control group (on day 26 after tumor inoculation). No significant difference in proportion of splenic mature DCs (CD11c+CD86+MHC II+) after cryo-thermal therapy with CXCL10 neutralizing antibody, however, mature DCs were abrogated after the treatment with IL-6 neutralizing antibody (). The data suggested that cryo-thermal-induced IL-6, but not CXCL10, promoted DCs phenotypic maturation.
Figure 4. CXCL10 and IL-6 induced by cryo-thermal therapy regulated functional and phenotypic characterization of DCs. (A and B) On day 14 after the treatment, the splenocytes were harvested from the mice in cryo-thermal + anti-IgG, cryo-thermal + anti-CXCL10, cryo-thermal + anti-IL-6 and the control groups, then the percentage of CD11c+CD86+MHC II+ DCs was analyzed by flow cytometry. All data were shown as mean ± SD. *p < .05. Data for bar graphs were calculated using one-way ANOVA. (C) The mRNA expression level of proinflammatory cytokines in splenic CD11c+ cells was measured by real-time PCR. (D) The mRNA expression level of immunosuppressive molecules in splenic CD11c+ cells was measured by real-time PCR. Data were shown as mean ± SD. Data for bar graphs were calculated using two-way ANOVA. Each value of *p < .05 or **p < .01 or ***p < .001 was considered to be statistically significant compared with the control group, &p < .05, &p < .01 and &&&p < .001 was considered to be statistically significant compared with cryo-thermal + anti-IgG group.
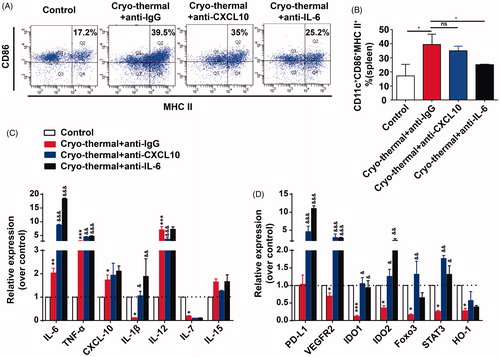
To address whether cryo-thermal-induced CXCL10 or IL-6 regulated the function of DCs, changes of mRNA expression in splenic CD11c+ DCs were assessed by RT-PCR. The mRNA expression of IL-6, IL-1β and TNF-α in DCs from the treated mice with in vivo CXCL10 neutralization was significantly increased as compared to isotype IgG group, whereas significantly decreased expression of IL-12 mRNA was observed. There was no significant change of CXCL10, IL-7 and IL-15 mRNA expression in DCs from the treated mice with in vivo CXCL10 neutralization (). As compared to isotype IgG group, the mRNA expression of IL-6, IL-1β and TNF-α in DCs from the treated mice with in vivo IL-6 neutralization was significantly up-regulated, whereas the expression level of CXCL10, IL-12, IL-7 and IL-15 mRNA was not affected (). Furthermore, the mRNA expression of representative immuno-inhibitory or regulatory molecules in splenic DCs was assessed by RT-PCR. As compared to isotype IgG group, PD-L1, VEGFR2, IDO1, IDO2, STAT3 and FOXO3 mRNA expression in DCs were markedly up-regulated, however, the mRNA expression of HO-1 was not affected in DCs from the treated mice with in vivo CXCL10 neutralization (). PD-L1, VEGFR2, IDO1, IDO2 and STAT3 mRNA expression in DCs were also markedly up-regulated, however, the mRNA expression of FOXO3 and HO-1 were not affected in DCs from the treated mice with in vivo IL-6 neutralization (). These results showed that CXCL10 did not affect the population of mature DCs, but modulated the expression of pro-inflammatory cytokines and immuno-inhibitory molecules in DCs. While IL-6 did not only affect the phenotype of DCs, but also affected DCs-secreted pro-inflammatory cytokines, co-stimulatory molecules and immuno-inhibitory molecules, which suggested that cryo-thermal-induced CXCL10 and, especially IL-6 modulated DCs activation and maturation.
Cryo-thermal-induced CXCL10 and IL-6 inhibited splenic MDSCs accumulation
MDSCs are negative regulators of anti-tumor immune response and usually inhibit the activity of T-lymphocyte [Citation36]. Our previous study shows that cryo-thermal therapy reduces the percentage of MDSCs in spleen [Citation25], therefore, we addressed whether the level of MDSCs in spleen would be affected after the treatment with in vivo IL-6 or CXCL10 neutralization. shows the representative flow cytometry diagram of splenic MDSC (MDSC was characterized as CD11b+Gr-1+ cells). The results showed that significant reduction of MDSCs in cryo-thermal treated mice with isotype IgG, whereas the percentage of MDSCs was markedly increased in cryo-thermal-treated mice with in vivo IL-6 or CXCL10 neutralization (). The data suggested that cryo-thermal-induced IL-6 and CXCL10 reduced MDSCs accumulation in spleen, which was more permissive for tumor eradication mediated by systemic anti-tumor immunity.
Figure 5. CXCL10 and IL-6 induced by cryo-thermal therapy inhibited MDSCs accumulation in spleen. (A and B) On day 14 after the treatment, the splenocytes were harvested from the mice in cryo-thermal + anti-IgG, cryo-thermal + anti-CXCL10, cryo-thermal + anti-IL-6 and the control groups, then the percentage of MDSCs was analyzed by flow cytometry. All data were shown as mean ± SD. *p < .05. Data for bar graphs were calculated using one-way ANOVA.
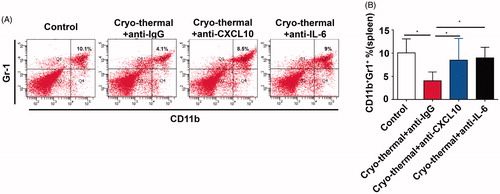
Cryo-thermal-induced IL-6, but not CXCL10 boosted CD4+T cells differentiation into dominant Th1 and CTL
CXCL10 can not only attract activated Th1 cells, but also lead to Th1 polarization [Citation13]. In addition, our previous study shows that IL-6 induced by cryo-thermal therapy promotes CD4+ T cell differentiation [Citation24]. Therefore, the percentages of splenic CD4+ T cells and mRNA expression profiles in different CD4+ T cell subsets were analyzed by flow cytometry and RT-PCR, respectively. The significantly increased proportion of CD3+CD4+ T cells was found on day 14 after cryo-thermal therapy with isotype IgG, while the proportion of CD3+CD4+ T cells was not affected after the treatment with in vivo IL-6 or CXCL10 neutralization ().
Figure 6. CXCL10 and IL-6 induced by cryo-thermal therapy affected splenic CD4+T cell differentiation. (A and B) On day 14 after the treatment, the splenocytes were harvested from the mice in cryo-thermal + anti-IgG, cryo-thermal + anti-CXCL10, cryo-thermal + anti-IL-6 and the control groups, then the percentage of CD3+CD4+ T cells was analyzed by flow cytometry. All data were shown as mean ± SD. *p < .05. Data for bar graphs were calculated using one-way ANOVA. (C–H) The mRNA expression level of transcription factors and cytokines in splenic CD4+T cells was measured by real-time PCR. Data were shown as mean ± SD. Data for bar graphs were calculated using two-way ANOVA. Each value of *p < .05 or **p < .01 or ***p < .001 was considered to be statistically significant compared with the control group, &p < .05, &p < .01 and &&&p < .001 was considered to be statistically significant compared with cryo-thermal + anti-IgG group.
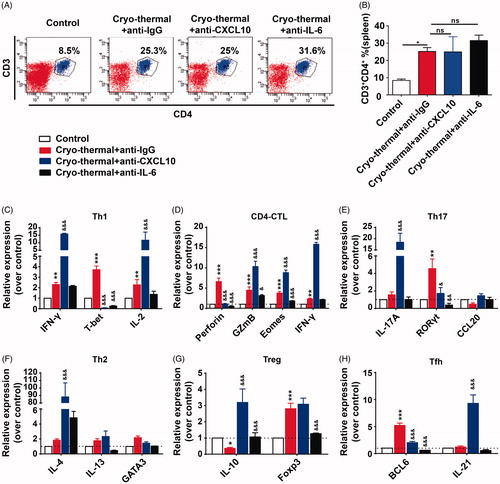
Then, the mRNA expression of different CD4+ T cell subsets was analyzed using RT-PCR. As compared to the control, the increased mRNA expression of Th1-associated genes (T-bet, IFN-γ and IL-2) and CTL-associated genes (Eomes, IFN-γ, GzmB and perforin) was observed after the treatment with isotype IgG, and mRNA expression of transcription factor in Th17 (RORγt), Treg (FoxP3) and Tfh (Bcl6) was also higher than that in the control group, whereas its representative cytokines (IL-17A, CCL20 and IL-21) were not affected and mRNA expression of IL-10 was decreased (). Th2-associated genes (GATA3, IL-4 and IL-13) were also not yet affected (). The data indicated that cryo-thermal therapy promoted CD4+T cell differentiation into dominant Th1 and CD4+ CTL. After the treatment with in vivo CXCL10 neutralization, the increased mRNA expression of Th1-associated genes (IFN-γ and IL-2), CTL-associated genes (Eomes, IFN-γ and GzmB), Th17 (IL-17A), Th2 (IL-4), Tregs (IL-10) and Tfh (IL-21) was noticed (), which indicated that cryo-thermal-induced CXCL10 did not regulate CD4+ Th1 and CTL polarization, but also modulated other CD4+ T cell subset differentiation. But, after the treatment with in vivo IL-6 neutralization, Th1 and CD4+ CTL polarization were completely abrogated (). These data suggested that cryo-thermal-induced IL-6 would promote CD4+ T cell differentiation into dominant Th1 and CD4+ CTL. All together, these results suggested that cryo-thermal-induced CXCL10 restricted CD4+ T cell excessive differentiation, but IL-6 promoted CD4+ T cell differentiation into dominant Th1 and CD4+ CTL.
Cryo-thermal-induced CXCL10 and IL-6 modulated cytotoxic function of CD8+T cells
In addition to splenic CD4+ T cells, we also accessed the percentage of splenic CD8+ T cells and the cytotoxic function of CD8+ T cells. The significantly increased proportion of CD3+CD8+ T cells was found following cryo-thermal therapy with isotype IgG. Interestingly, the markedly increased proportion of CD3+CD8+ T cells was observed after cryo-thermal therapy with in vivo CXCL10 neutralization, whereas the proportion of CD3+CD8+ T cells was not affected by in vivo IL-6 neutralization (). The gene expression of cytotoxic cytokines in splenic CD8+ T cells in the treated mice was determined using RT-PCR. Cryo-thermal therapy resulted in higher mRNA expression levels of IFN-γ, GzmB, and perforin in splenic CD8+ T cells, but much higher expression of IFN-γ mRNA in splenic CD8+ T cells was found after the treatment with in vivo CXCL10 neutralization, though GzmB and perforin mRNA expression was not affected yet (). Furthermore, the mRNA expression of IFN-γ and perforin was not affected yet after the treatment with in vivo IL-6 neutralization; however, the mRNA expression of GzmB was down-regulated (). Our findings showed that in vivo CXCL10 neutralization resulted in the increased proportion of CD3+CD8+ T cells with high expression of IFN-γ, which suggested that cryo-thermal-induced CXCL10 modulated the level of CD8+ T cells and cytotoxic function, while IL-6 only regulated the expression of GzmB in CD8+ T cells.
Figure 7. CXCL10 and IL-6 induced by cryo-thermal therapy modulated functional phenotype of CD8+T cells. (A and B) On day 14 after the treatment, the splenocytes were harvested from the mice in cryo-thermal + anti-IgG, cryo-thermal + anti-CXCL10, cryo-thermal + anti-IL-6 and the control groups, then the percentage of CD3+CD8+ T cells was analyzed by flow cytometry. All data were shown as mean ± SD. *p < .05 or **p < .01. Data for bar graphs were calculated using one-way ANOVA. (C) The mRNA expression level of cytotoxic cytokines in splenic CD8+T cells was measured by real-time PCR. Data were shown as mean ± SD. Data for bar graphs were calculated using two-way ANOVA. Each value of *p < .05 or **p < .01 or ***p < .001 was considered to be statistically significant compared with the control group, &p < .05, &p < .01 and &&&p < .001 was considered to be statistically significant compared with cryo-thermal + anti-IgG group.
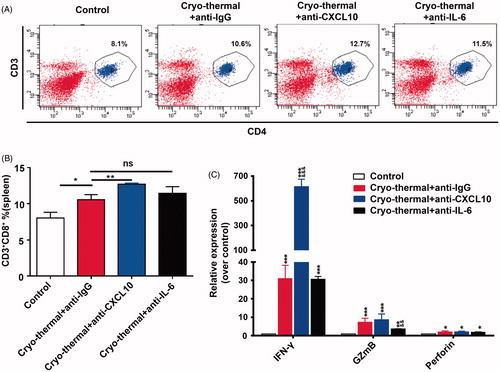
Discussion
Our previous study reports that cryo-thermal therapy leads to long-term tumor-free survival that is dependent on CD4+ T cell-mediated anti-tumor immune response [Citation25]. Our recent study revealed that cryo-thermal-induced M1 macrophage polarization modulated the phenotypic and functional maturation of DCs, which contributed to CD4+ T cell differentiation into Th1 and CD4+ CTL sub-lineages. In particular, cryo-thermal therapy triggered M1 macrophage polarization with up-regulated expression of CXCL10 and IL-6 (submitted). But whether CXCL10 and IL-6 involved in CD4+ T cell-mediated anti-tumor immune memory response elicited by cryo-thermal therapy did not understand. In this study, our findings showed that cryo-thermal therapy increased CXCL10 and IL-6 production by macrophage to create acute inflammatory environment in spleen to initiate a systemically cascading innate and adaptive anti-tumor immunity, which induced M1 macrophage polarization, DCs maturation, reduced MDSCs accumulation and subsequently promoted CD4+T cell differentiation into Th1 and CD4+ CTL.
Cytokines and chemokines are required for activation, differentiation and migration of immune cells [Citation37,Citation38]. In an immunostimulatory environment, pro-inflammatory immune cells, especially DCs and macrophages can activate CD4+ and CD8+T cells to mediate tumor killing [Citation39,Citation40]. Acute inflammatory response is directly associated with anti-tumor immunity, while chronic inflammation is associated with tumor development [Citation9,Citation10]. Therefore, we proposed that the phase, duration and extent of cryo-thermal-induced CXCL10 and IL-6 were the key factors for durable anti-tumor immunity. CXCL10 and IL-6 were rich in splenic environment after cryo-thermal therapy, and the level of IL-6 was strikingly higher than that in hyperthermia group. Because IL-6 played the key role of M1 macrophage polarization and DCs maturation, we supposed that IL-6 production by hyperthermia was relatively devoid, which could not effectively drive CD4+ T cell differentiation into Th1 and CD4+ CTL subsets.
DCs are able to stimulate T-cell proliferation and secretion of IFN-γ [Citation41]. Systemic DC activation modulates immunosuppression and shapes the long-lived memory T cells [Citation42]. However, matured DCs would be generated when the right cells and soluble factors are presented [Citation37]. CXCL10 acts as a chemoattractant for DCs and contributes to DCs maturation and activation [Citation34,Citation35]. In cryo-thermal-induced CXCL10-rich inflammatory environment, splenic macrophage-derived CXCL10 attracted DCs migration, and induced DCs maturation, which subsequently promoted CD4+ T cell recruitment and differentiation. Previously, our results revealed that cryo-thermal-induced acute IL-6 promotes DCs phenotypic maturation in vitro [Citation24]. In this study, in vivo IL-6 neutralization resulted in less CD11c+ CD86+ MHC II+ matured DCs and up-regulated the expression of immuno-inhibitory molecules, which suggested that cryo-thermal-induced IL-6 mediated DCs maturation.
CXCL10 can mediate recruitment and activation of macrophages [Citation17,Citation31]. Cryo-thermal-induced CXCL10 modulated M1 macrophage polarization along with up-regulation of proinflammatory cytokines (IL-6, TNF-α, IL-12 and CXCL12), while down-regulating expression of M2 macrophage-associated cytokines, which indicated that CXCL10 promoted M1 macrophage polarization. In addition, high-level production of IL-6 can promote polarization of M1 macrophages [Citation43,Citation44]. In this study, IL-6 modulated expression of inflammatory cytokines in M1 macrophages. Altogether, our studies indicated that CXCL10 and IL-6 were key inflammatory factors in M1 macrophage polarization.
Inflammatory factors in the immune environment are required for T cell-mediated anti-tumor immunity [Citation45]. After cryo-thermal therapy with in vivo CXCL10 neutralization, CD4+ T cell subsets (Th1, CTL, Th17, Th2, Tregs and Tfh) exhibited excessive differentiation, and could not present dominant subsets of Th1 and CD4+ CTL. CXCL10 induces an upregulation of T-bet mRNA expression, while a down-regulation of GATA-3 mRNA expression, leading to Th1 polarization [Citation13,Citation46]. As CXCL10.blockade, the mRNA expression of T-bet was decreased while the mRNA expression of IL-4 was increased, which is associated with tumor progression [Citation47], moreover, the up-regulated mRNA expression of IL-10 in CD4+T cells after cryo-thermal therapy with in vivo CXCL10 neutralization indicates T cell immunosuppression [Citation48]. Therefore, we speculated that, though CD4+ T cell subsets exhibited excessive differentiation after cryo-thermal therapy with in vivo CXCL10 neutralization, the host would be in T cell immunosuppressive state. Th17 cells are involved with autoimmunity and contribution of Th17 cells in anti-tumor immune protection or tumor progression remains controversial [Citation49]. The role of up-regulated IL-17A expression in CD4+T cells after the treatment with in vivo CXCL10 neutralization need to be further studied. Cryo-thermal therapy with in vivo CXCL10 neutralization promoted much higher mRNA expression of IFN-γ in splenic CD8+ T cells. IFN-γ involves in promoting outgrowth of tumor cells with immunosuppressive phenotype when a chronic IFN-γ presents in the immune environment [Citation50]. Thus, cryo-thermal-induced CXCL10 could restrict excessive differentiation of CD4+ T cells and excessive expression of IFN-γ in CD8+ T cells.
In this study, Th1-associated genes (IFN-γ and IL-2) and CTL-associated genes (Eomes, IFN-γ and GzmB) were significantly up-regulated after cryo-thermal therapy with in vivo CXCL10 neutralization, but transcription of T-bet was inhibited after CXCL10 blockade. When transcription of T-bet is blocked, transcriptional factor Eomes appears to complement T-bet in mediating T cell differentiation and promoting the expression of IFN-γ, IL-2 and GzmB in T cells [Citation51].
Effectively remodeling immunosuppression to immunostimulation was one of the key factors to promote anti-tumor immunity. In vivo CXCL10 and IL-6 neutralization increased the proportion of MDSCs in spleen. High level of CXCL10 may contribute to the suppression of MDSCs development [Citation6]. CCL2 is one of the primary chemokines to attract MDSCs, the transcript of CCL2 mRNA in spleen was significantly reduced on day 14 after cryo-thermal therapy, CCL2 blockade leads to up-regulate CXCL10 expression [Citation52]. After cryo-thermal therapy with in vivo IL-6 or CXCL10 neutralization, the expression of proinflammatory cytokines (TNF-α, IL-6 and IL-1β) in DCs was up-regulated. However, previous study found that the expression of these cytokines peaked on day 5, but was down-regulated on day 14 after cryo-thermal therapy (submitted). We speculated that the overexpression pattern of these pro-inflammatory cytokines on day 14 after cryo-thermal therapy with in vivo IL-6 or CXCL10 neutralization would be a chronic inflammatory profile, which mediated MDSCs accumulation in spleen. Therefore, we suggested that cryo-thermal therapy triggered M1 macrophage polarization with up-regulated expression of CXCL10 and IL-6 to build immune environment, which subsequently led to the differentiation of CD4+T cells to Th1 and CD4+ CTL subsets to orchestrate anti-tumor immune response.
Conclusion
We demonstrated that cryo-thermal therapy inducing MI macrophage polarization created a CXCL10 and IL-6-rich pro-inflammatory environment, in which promoted DCs maturation and CD4+ T cell differentiation into Th1 and CD4+ CTL. It was clear that pro-inflammatory responses induced by cryo-thermal therapy were critical for modulating anti-tumor immunity.
Figures S1-S2 and Table S1
Download PDF (467.3 KB)Disclosure statement
No potential conflict of interest was reported by the authors.
Additional information
Funding
References
- Modiano JF, Bellgrau D, Cutter GR, et al. Inflammation, apoptosis, and necrosis induced by neoadjuvant fas ligand gene therapy improves survival of dogs with spontaneous bone cancer. Mol Ther. 2012;20:2234–2243.
- Shimizu M, Fontana A, Takeda Y, et al. Induction of antitumor immunity with fas/apo-1 ligand (cd95l)-transfected neuroblastoma neuro-2a cells. J Immunol. 1999;162:7350–7357.
- Hohlbaum AM, Gregory MS, Ju ST, et al. Fas ligand engagement of resident peritoneal macrophages in vivo induces apoptosis and the production of neutrophil chemotactic factors. J Immunol. 2001;167:6217–6224.
- Atsumi T, Singh R, Sabharwal L, et al. Inflammation amplifier, a new paradigm in cancer biology. Cancer Res. 2014;74:8.
- Bowen DG, Walker CM. Adaptive immune responses in acute and chronic hepatitis C virus infection. Nature. 2005;436:946–952.
- Thakur A, Sarkar SH, Al-Khadimi Z, et al. A Th1 cytokine–enriched microenvironment enhances tumor killing by activated T cells armed with bispecific antibodies and inhibits the development of myeloid-derived suppressor cells. Cancer Immunol Immunother. 2012;61:497–509.
- Wang D, DuBois RN. Immunosuppression associated with chronic inflammation in the tumor microenvironment. Carcinogenesis. 2015;36:1085–1093.
- Gabay C. Interleukin-6 and chronic inflammation. Arthritis Res Ther. 2006;8:S3.
- Fisher DT, Appenheimer MM, Evans SS. The two faces of IL-6 in the tumor microenvironment. Semin Immunol. 2014;26:38–47.
- Dmitrieva OS, Shilovskiy IP, Khaitov MR, et al. Interleukins 1 and 6 as main mediators of inflammation and cancer. Biochemistry (Mosc). 2016;81:80–90.
- Dong C. TH17 cells in development: an updated view of their molecular identity and genetic programming. Nat Rev Immunol. 2008;8:337.
- Tanaka T, Narazaki M, Kishimoto T. IL-6 in inflammation, immunity, and disease. Cold Spring Harb Perspect Biol. 2014;6:a016295.
- Tokunaga R, Zhang W, Naseem M, et al. CXCL9, CXCL10, CXCL11/CXCR3 axis for immune activation – a target for novel cancer therapy. Cancer Treat Rev. 2018;63:40–47.
- Lunardi S, Lim SY, Muschel RJ, et al. IP-10/CXCL10 attracts regulatory T cells: implication for pancreatic cancer. Oncoimmunology. 2015;4:e1027473.
- Xanthou G, Duchesnes CE, Williams TJ, et al. CCR3 functional responses are regulated by both CXCR3 and its ligands CXCL9, CXCL10 and CXCL11. Eur J Immunol. 2003;33:2241–2250.
- Schoenborn JR, Wilson CB. Regulation of interferon-gamma during innate and adaptive immune responses. Adv Immunol. 2007;96:41–101.
- Oghumu S, Varikuti S, Terrazas C, et al. CXCR3 deficiency enhances tumor progression by promoting macrophage M2 polarization in a murine breast cancer model. Immunology. 2014;143:109–119.
- Sun J, Zhang A, Xu LX. Evaluation of alternate cooling and heating for tumor treatment. Int J Heat Mass Transf. 2008;51:5478–5485.
- Sun JQ, Xu CC, Wei GH, et al. Tumor treatment system with alternate cooling and heating-preliminary results in an animal model. In: Olaf Dössel, Wolfgang C. Schlegel, editors. World congress on medical physics and biomedical engineering. Vol. 25. Switzerland: Springer Nature; 2009. p. 337–340.
- Liu P, Ren X, Xu LX. Alternate cooling and heating thermal physical treatment: an effective strategy against MDSCs in 4T1 mouse mammary carcinoma. ASME. 2012;45:937–938.
- Cai Z, Song M, Zhang A, et al. Numerical simulation of a new probe for the alternate cooling and heating of a subcutaneous mouse tumor model. Numer Heat Trans A Appl. 2013;63:534–548.
- Zhu J, Zhang Y, Zhang A, et al. Cryo-thermal therapy elicits potent anti-tumor immunity by inducing extracellular Hsp70-dependent MDSC differentiation. Sci Rep. 2016;6:27136.
- Xue T, Ping L, Yong Z, et al. Interleukin-6 induced “acute” phenotypic microenvironment promotes th1 anti-tumor immunity in cryo-thermal therapy revealed by shotgun and parallel reaction monitoring proteomics. Theranostics. 2016;6:773–794.
- Liu K, He K, Xue T, et al. The cryo-thermal therapy-induced IL-6-rich acute pro-inflammatory response promoted DCs phenotypic maturation as the prerequisite to CD4+ T cell differentiation. Int J Hyperth. 2017;34:261–272.
- He K, Liu P, Xu LX. The cryo-thermal therapy eradicated melanoma in mice by eliciting CD4+ T-cell-mediated antitumor memory immune response. Cell Death Dis. 2017;8:e2703.
- Chen C, Zhang A, Cai Z, et al. Design of microprobe for accurate thermal treatment of tumor. Cryo Letters. 2011;32:275–286.
- Cai Z, Song M, Sun J, et al. Design of a new probe for tumor treatment in the alternate thermal system based on numerical simulation. Engineering in medicine and biology society, EMBC, 2011 Annual international conference of the IEEE; 2011; Boston, MA.
- Moore TC, Kumm PM, Brown DM, et al. Interferon response factor 3 is crucial to poly-I:C induced NK cell activity and control of B16 melanoma growth. Cancer Lett. 2014;346:122–128.
- Barreira dSR, Laird ME, Yatim N, et al. Dipeptidylpeptidase 4 inhibition enhances lymphocyte trafficking, improving both naturally occurring tumor immunity and immunotherapy. Nat Immunol. 2015;16:850–858.
- Fearon DT. Immune-suppressing cellular elements of the tumor microenvironment. Annu Rev Cancer Biol. 2017;1:241–255.
- Zhou J, Tang PC, Qin L, et al. CXCR3-dependent accumulation and activation of perivascular macrophages is necessary for homeostatic arterial remodeling to hemodynamic stresses. J Exp Med. 2010;207:1951–1966.
- Chomarat P, Banchereau J, Davoust J, et al. IL-6 switches the differentiation of monocytes from dendritic cells to macrophages. Nat Immunol. 2000;1:510–514.
- Groom JR, Luster AD. CXCR3 ligands: redundant, collaborative and antagonistic functions. Immunol Cell Biol. 2011;89:207–215.
- Liu M, Guo S, Hibbert JM, et al. CXCL10/IP-10 in infectious diseases pathogenesis and potential therapeutic implications. Cytokine Growth Factor Rev. 2011;22:121–130.
- Lai C, Yu X, Zhuo H, et al. Anti-tumor immune response of folate-conjugated chitosan nanoparticles containing the IP-10 gene in mice with hepatocellular carcinoma. J Biomed Nanotechnol. 2014;10:3576–3589.
- Gabrilovich DI, Ostrand-Rosenberg S, Bronte V. Coordinated regulation of myeloid cells by tumours. Nat Rev Immunol. 2012;12:253–268.
- Nelles ME, Moreau JM, Furlonger CL, et al. Murine splenic CD4(+) T cells, induced by innate immune cell interactions and secreted factors, develop antileukemia cytotoxicity. Cancer Immunol Res. 2014;2:1113–1124.
- Lin WW, Karin M. A cytokine-mediated link between innate immunity, inflammation, and cancer. J Clin Invest. 2007;117:1175–1183.
- Belardelli F, Ferrantini M. Cytokines as a link between innate and adaptive antitumor immunity. Trends Immunol. 2002;23:201–208.
- Striz I, Brabcova E, Kolesar L, et al. Cytokine networking of innate immunity cells: a potential target of therapy. Clin Sci. 2014;126:593–612.
- Dudek AM, Martin S, Garg AD, et al. Immature, semi-mature, and fully mature dendritic cells: toward a DC-cancer cells interface that augments anticancer immunity. Front Immunol. 2013;4:438.
- Tran Janco JM, Lamichhane P, Karyampudi L, et al. Tumor-infiltrating dendritic cells in cancer pathogenesis. J Immunol. 2015;194:2985–2991.
- Hope JC, Cumberbatch M, Fielding I, et al. Identification of dendritic cells as a major source of interleukin-6 in draining lymph nodes following skin sensitization of mice. Immunology. 1995;86:441–447.
- Schaefer E, Wu W, Mark C, et al. Intermittent hypoxia is a proinflammatory stimulus resulting in IL-6 expression and M1 macrophage polarization. Hepatol Commun. 2017;1:326.
- Kim HJ, Cantor H. CD4 T-cell subsets and tumor immunity: the helpful and the not-so-helpful. Cancer Immunol Res. 2014;2:91–98.
- Romagnani P, Maggi L, Mazzinghi B, et al. CXCR3-mediated opposite effects of CXCL10 and CXCL4 on TH1 or TH2 cytokine production. J Allergy Clin Immunol. 2005;116:1372–1379.
- Kammertoens T, Qin Z, Briesemeister D, et al. B-cells and IL-4 promote methylcholanthrene-induced carcinogenesis but there is no evidence for a role of T/NKT-cells and their effector molecules (Fas-ligand, TNF-α, perforin)). Int J Cancer. 2012;131:1499–1508.
- Facciabene A, Motz GT, Coukos G. T-regulatory cells: key players in tumor immune escape and angiogenesis. Cancer Res. 2012;72:2162–2171.
- Asadzadeh Z, Mohammadi H, Safarzadeh E, et al. The paradox of Th17 cell functions in tumor immunity. Cell Immunol. 2017;322:15–25.
- Mojic M, Takeda K, Hayakawa Y. The dark side of IFN-gamma: its role in promoting cancer immunoevasion. Int J Mol Sci. 2017;19:89.
- Pearce EL, Mullen AC, Martins GA, et al. Control of effector CD8+ T cell function by the transcription factor eomesodermin. Science. 2003;302:1041–1043.
- Fujita M, Kohanbash G, Fellows-Mayle W, et al. COX-2 blockade suppresses gliomagenesis by inhibiting myeloid-derived suppressor cells. Cancer Res. 2011;71:2664.