Abstract
Minimally invasive percutaneous thermal ablation of osseous metastases has proved safe and effective in management of selected patients with bone metastatic disease. These procedures have become a part of the treatment algorithm for certain subgroup of patients with osseous metastases to achieve pain palliation and/or local tumor control. This review details the armamentarium available and the most recent advances in minimally invasive, percutaneous image-guided thermal ablation for management of osseous metastases.
Introduction
Bone is the third most common site of metastatic disease; up to 85% of patients with breast, prostate, kidney, and lung cancers are found to have osseous metastases at autopsy [Citation1]. Symptomatic patients most commonly present with pain, which is multifactorial owing to tumor-induced biochemical stimulation of periosteal and endosteal nociceptors, tumor mass effect on surrounding vital structures particularly nerves or spinal cord, or associated pathologic fracture [Citation1,Citation2]. In patients with painful osseous metastases, the goal of therapy is to achieve rapid and durable pain relief [Citation3]. Radiation therapy is the standard of care for pain palliation secondary to metastases, but it has important limitations. First, certain tumor histologies respond variably to radiation therapy, particularly renal cell carcinoma, melanoma, and soft tissue sarcoma [Citation4–6]. Second, radiation therapy may be limited by the cumulative radiation tolerance of nearby radiosensitive organs, such as the spinal cord or bowel. Third, some patients may not respond until 4 to 6 weeks following radiation therapy, which is suboptimal for patients with short life expectancies [Citation7]. Finally, radiation therapy may exclude patients from certain clinical trials. Additionally, painful bone metastases are often refractory to systemic therapies such as chemotherapy, hormonal therapy, radiopharmaceuticals, and bisphosphonates [Citation8]. Surgical intervention, including resection, cementation and internal fixation, and stabilization, is often of limited benefit in patients with osseous metastases because of its morbidity, patients’ often poor functional status, and the short expected life span. These procedures are generally reserved for patients with neurologic compromise or osseous instability. Pain palliation with systemic analgesics remains the only alternative for many patients [Citation9]. Over the past few years, investigators have exploited the use of minimally invasive percutaneous thermal ablation technologies for management of osseous metastases including radiofrequency ablation (RFA), cryoablation, microwave ablation, and high-intensity focused ultrasound treatment [Citation10–32]. There is typically rapid recovery and no hindrance or compromise of adjuvant radiation or chemotherapy. Percutaneous thermal ablation for osseous metastases is performed to achieve pain palliation, local tumor control, or both (often with cementation for pathologic fracture stabilization or prevention). In cases of osseous oligometastatic disease (less than 5 lesions), ablation may be performed with curative intent [Citation14–16,Citation27,Citation28]. The most critical factor determining effective local tumor control following thermal ablation is probably small tumor size (less than 2 cm) along with favorable patient criteria, particularly oligometastatic status [Citation27,Citation28].
This review details the armamentarium available and the most recent advances in minimally invasive, percutaneous image-guided thermal ablation for management of osseous metastases.
Thermal ablation technologies
Radiofrequency ablation
In RFA, a generator delivers high-frequency, alternating current (375–600 kHz) to the target tissue through the exposed active tip of an electrode resulting in oscillation of charged tissue molecules within the ablation zone producing frictional heat. The thermal effect depends upon the electrical-conducting properties of the target tissue and the characteristics of the electrode [Citation33]. When local tissue temperature between 60–100 °C is reached, protein denaturation and immediate coagulative necrosis occur [Citation33]. Several RFA electrode design technologies have been developed to achieve an improved ablation zone [Citation33]. Unipolar systems utilize dispersing grounding pads placed on patient skin near the ablation site to serve as the receiving limb of the electrical circuit to prevent potential skin burn. Bipolar systems use built-in transmitting and receiving electrical elements within the electrode which eliminate the need for grounding pads and possibility of skin burn [Citation21,Citation23]. Internally cooled electrodes decrease tissue char (carbonization) by maintaining lower temperature surrounding the active electrode tip [Citation33].
Recently, navigational bipolar RFA electrodes with built-in thermocouples have become available which allow real-time intra-procedural monitoring of ablation zone size by measuring the temperatures along the periphery of the ablation zone based on pre-determined manufacturer data [Citation21,Citation23] (). The navigating tip of the electrode can be articulated in different orientations through the same entry site which is beneficial for accessing lesions in challenging locations, particularly along the posterior vertebral body, and achieving larger ablation zones [Citation21,Citation23].
Figure 1. 59-year-old man with metastatic lung cancer and painful T7 pathologic fracture. Sagittal T1-weighted fat-saturated contrast-enhanced MR image (A) shows enhancing bone marrow replacing lesion within T7 vertebral body with pathologic compression fracture (A, arrow). Anterior-posterior fluoroscopic image during simultaneous bipedicular radiofrequency ablation (B) shows medial articulation of electrode tips which are 5–10 mm apart (width of the spinous process as landmark). Radiofrequency ablation was performed for a total of 16 min, and immediately followed by vertebral augmentation (not shown). Sagittal STIR MR image obtained 6 months following treatment (C) shows local tumor control with no evidence of recurrence (C, arrow). Note hypointense cement within vertebral body.
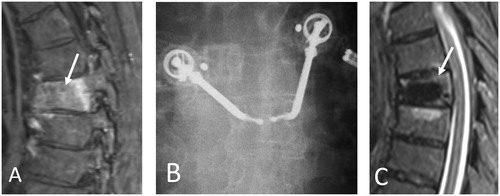
Simultaneous bipedicular vertebral RFA is a novel technique that efficiently generates two confluent, coalescent, and overlapping ablation zones in close proximity that minimize the convective cooling effect (heat sink), and subsequently decreases the power required to conduct heat through tissue decreasing the risk of thermal injury and minimizing charring and impedance related issues [Citation23].This technique may result in a more thorough ablation of the vertebral body and pedicles, and support the stereotactic spine radiosurgery paradigm to treat the entire vertebral body volume and pedicles for improved local tumor control rates and more durable pain palliation [Citation23,Citation34].
RFA is typically used for treatment of osseous lesions with no or small extra-osseous components, as well as osseous tumor which are primarily osteolytic as the higher intrinsic impedance of osteoblastic lesions prevents the RF circuit from generating sufficiently high temperatures to ensure cell death and renders RFA ineffective [Citation35]. Specifically, navigational bipolar RFA electrodes are preferred for treatment of posterior vertebral body metastases which are not readily accessible with straight electrodes [Citation21,Citation23]. Built-in thermocouples along the navigational bipolar RFA electrodes allow real-time precise monitoring of ablation zone size and geometry beyond which tissues are safe from potential thermal injury [Citation21,Citation23]. Intact bone cortex is considered a boundary for undesired RF energy propagation [Citation36].
Over the past several years, investigators have demonstrated safety and efficacy of RFA for management of patients with painful osseous metastatic disease to achieve pain palliation and/or local tumor control often combined with cementoplasty for pathological fracture stabilization or prevention [Citation10,Citation11,Citation21–23,Citation25]. In a multicenter single-arm prospective American College of Radiology Imaging Network trial, 55 patients underwent RFA for management of single painful osseous metastasis, and substantial pain relief and improved patient mood was achieved at 1-month and 3-month post-procedural time points [Citation10]. Grade 3 complications occurred in 5% of patients. In a multi-center clinical trial [Citation11], 43 patients with painful osteolytic osseous metastases were treated with RFA and 95% of patients had clinically significant improved pain with decreased opioid usage following the procedure. There were 3 complications including one skin burn at the grounding pad site, one acetabular fracture, and one transient bowel/bladder incontinence following a sacral RFA. In a retrospective multi-center study, investigators treated 128 vertebral metastases in 96 patients (vertebral augmentation in 92 patients) using bipolar navigational RFA electrodes implementing thermo-protective techniques and achieved pain palliation (improved visual analog scores and decreased opioid usage in majority of patients) in 1-week, 1-month, and 6-month intervals following procedures with no major complication [Citation21]. In a multicenter prospective study, Bagla et al treated 50 patients with spinal metastases using RFA (69 ablations) and vertebral augmentation (96% of patients) and achieved significant reduction in pain and disability along with improvement in functional status and quality of life with no complications [Citation22].
In a retrospective study, combination of RFA (bipolar navigational electrodes) and vertebral augmentation was used to treat 55 patients with vertebral metastases. Local tumor control was achieved in 89%, 74%, and 70% of cases at 3-months, 6-months, and 1-year post-procedure time points (median follow up of 34 weeks) with no major complications and no clinical evidence of metastatic spinal cord compression at treated levels [Citation25].
There has been recent paradigm shift in stereotactic spine radiosurgery for management of vertebral metastases with specific consensus recommendations by the international spine radiosurgery consortium for definition of clinical target volume (CTV) versus gross tumor volume (GTV) to account for microscopic tumor spread and marginal radiation therapy failures [Citation34]. The consensus recommendation defines CTV (to be treated by stereotactic spine radiosurgery) to include GTV plus surrounding abnormal bone marrow signal intensity on magnetic resonance imaging (MRI) to account for microscopic tumor invasion and adjacent normal osseous expansion to account for subclinical tumor spread in marrow space [Citation34]. In support of this paradigm shift, Tomasian et al. [Citation23] treated 33 tumors (27 patients) using simultaneous bipedicular RFA combined with vertebral augmentation and achieved local tumor control in 96% of cases with no complications and no clinical evidence of metastatic spinal cord compression at the treated levels (mean follow-up, 24.2 weeks). In addition, simultaneous bipedicular RFA using cooled bipolar electrode system has been utilized for management of vertebral metastases [Citation30,Citation31].
Limitations of RFA include non-visualization of ablation zone boundary with CT, procedure-related pain, and, frequently, increased pain during the immediate post-ablation period.
Cryoablation
In closed percutaneous cryoablation systems, liquid argon is typically utilized to rapidly drop the temperature at the cryoprobe tip using the Joule-Thomson effect (pressurized gas, when allowed to expand, results in temperature drop).The rapid cooling is exchanged with adjacent tissues forming an enlarging ice-ball with time followed by a ‘thawing’ phase, resulting in osmotic gradient across the cell membrane [Citation33,Citation37]. Formation of extracellular ice results in relative imbalance of intra- and extracellular electrolytes, and subsequently extracellular water shift by osmosis and cellular dehydration. The increase in intracellular electrolyte concentration results in damage to both the cellular enzymes and membrane [Citation33,Citation37]. Formation of intracellular ice crystals damages the cellular organelles. Both freezing and thawing lead to osmotic gradient and consequent cell membrane injury [Citation33,Citation37]. A temperature of −40 °C or lower is necessary to ensure complete cell death [Citation38].
Currently, the cryoprobes of two major manufacturers are utilized in the musculoskeletal system with diameters ranging from 1.2 mm to 2.4 mm, generating predictable ablation zones, according to pre-determined manufacturer data, utilizing at least 1 freeze/active thaw/freeze cycle (typically 10 min/5 min/10 min) [Citation12,Citation13,Citation16] (). Cryoablation is typically used for osseous metastases with large soft tissue components, or large lesions involving the posterior vertebral elements, and is preferred to RFA for management of osteoblastic lesions.
Figure 2. 60-year-old woman with metastatic peripheral nerve sheath tumor previously managed by surgical resection and adjuvant chemoradiation therapy presenting with recurrent tumor involving upper lumbar vertebral posterior elements and paraspinal soft tissues. Axial FDG-PET-CT image (A) shows hypermetabolic soft tissue mass within posterior upper lumbar paraspinal soft tissues (A, arrow). Prone axial (B) CT image during cryoablation shows placement of a cryoprobe (a total of 3 cryoprobes were used) within the lesion with hypoattenuating ice ball encompassing the tumor volume (B, arrows). Axial FDG-PET-CT image obtained 3 months following cryoablation (C) shows local tumor control with no evidence of residual or recurrent tumor (C, arrow).
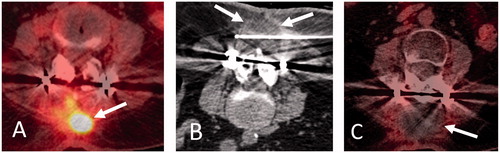
Advantages of cryoablation include formation of a hypoattenuating ice ball in the soft tissues which is readily identified by CT, decreased intra-procedural and post-procedural pain compared with RFA or microwave ablation, and ability to use multiple cryoprobes in various orientations to sculpt and achieve additive overlapping ablation zones. The intra-osseous ice ball may not be visible on CT particularly with osteoblastic lesions. An inter-cryoprobe distance of 1–1.5 cm is typically recommended with cryoprobe tips at bone-tumor or soft tissue-tumor interfaces [Citation13,Citation16]. The ice-ball peripheral margin corresponds to 0 °C, which is typically not sufficient for permanent tumor destruction. Reliable cell death is achieved at 3 mm margin from the peripheral edge, and therefore the ice-ball should extend beyond tumor margins [Citation39]. MRI-compatible cryoprobes offer an alternative approach, which may permit safe ablation of spinal lesions given the proximity of neural elements [Citation40].Advantages of MRI guidance include high tissue contrast resolution, lack of ionizing radiation, improved visualization of intra-osseous ice ball particularly in treatment of osteoblastic lesions, and possibility of real time temperature mapping.
Callstrom et al. [Citation18] reported successful treatment of 14 patients with osseous metastases refractory to chemoradiation therapy with cryoablation achieving substantial pain relief and decreased opioid use at 4-week time point after the procedure with no major complications. This was followed up with a multi-center clinical trial in 61 patients (69 treated lesions) which demonstrated substantial improvement in pain and quality of life as evaluated by Brief Pain Inventory Scale at 1, 4, 8, and 24 weeks after the procedure [Citation13]. Prologo et al. reported safety and effectiveness of percutaneous cryoablation for pain palliation in musculoskeletal metastases in a single-center retrospective study in 50 patients (54 tumors) with substantially decreased narcotics use and improved pain at 3-month follow up [Citation15]. Major complications included 2 proximal femur fractures and 2 peri-ablation neuropathies. Wallace et al. [Citation16] cryoablated 92 musculoskeletal metastases in 56 patients for pain palliation and/or local tumor control and concurrent cementoplasty was performed in 28% of patients. Radiographic local tumor control rates were 90% (37/41) at 3 months, 84% (27/32) at 6 months, and 73% (19/26) at 12 months after treatment. Improved median pain scores were reported up to 3 months following the procedure [Citation16]. McMenomy et al. performed percutaneous cryoablation on 43 patients (52 treated tumors) with musculoskeletal oligometastatic disease (five or fewer metastases) with aim of local tumor control and disease remission [Citation14]. Local tumor control was achieved in 87% (45 of 52 lesions) at median follow up of 21 months. One- and 2-year disease-free survival was 22% and 7%, respectively and median disease-free survival was 7 months [Citation14].
In a single-center retrospective study, Tomasian et al treated 31 vertebral metastases in 14 patients with cryoablation implementing thermo-protective techniques and achieved 96.7% local tumor control and statistically significant pain palliation and decreased analgesics usage at 1-week, 1-month, and 3-month post-procedural time points with no major complications [Citation12].
Microwave ablation
Microwave ablation utilizes antennae to deliver electromagnetic microwaves (approximately 900 and 2450 MHz) to target tissue which result in agitation of ionic molecules, frictional heat, and tissue coagulative necrosis. It is postulated that microwave ablation is less influenced by variable tissue impedance and perfusion-mediated tissue cooling, potentially resulting in higher intra-tumoral temperatures, larger and more uniform ablation zone and more efficient ablation using a single antenna, offering efficacy in management of osteoblastic lesions and obviating the need for grounding pads and thus diminished risk of skin burn [Citation17,Citation24,Citation26] (). Antenna shaft cooling system implemented in latest generations of microwave ablation equipment eliminates the risk of back-heating phenomena.
Figure 3. 55-year-old woman with metastatic lung adenocarcinoma and progressively enlarging left shoulder girdle metastatic lesion and intractable pain minimally relieved by oral and intravenous pain medications, admitted for pain palliation. Axial contrast-enhanced CT image (A) shows metastatic lesion involving the proximal humerus and scapular glenoid with glenohumeral intra-articular extension and surrounding shoulder girdle musculature involvement (A, arrows). Note metallic streak artifact from implants transfixing a proximal humerus pathologic fracture (not shown). Axial CT image during microwave ablation (B) shows placement of two microwave antennae within the soft tissue component of the metastatic lesion. Microwave ablation was performed at 100 Watts for a total of 10 min achieving overlapping 4 × 4 cm ablation zones. Antennae were repositioned caudally to ablate the inferior component of the lesion with identical ablation parameters. Note intra-tumoral gas from tissue vaporization (B, arrow). Axial CT image immediately following microwave ablation (C) shows largely CT-occult ablation zone with intra-tumoral gas from tissue vaporization (C, arrow). The patient endorsed substantial pain improvement.
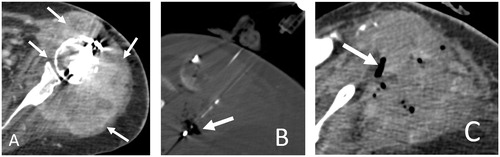
Rapid delivery of high amounts of power (up to 100 Watts) to large ablation zones might be a disadvantage when applied to spinal lesions as surrounding overheating could potentially lead to neural injuries Although hypo-attenuating ablated tissue is often identified on CT, margins of ablation zone are not well defined which is a disadvantage of spinal microwave ablation.
Pusceddu et al. [Citation26] utilized combination microwave ablation and cementoplasty to treat 35 patients (37 osseous metastatic lesions) and reported mean reduction in the VAS score of 84%, 90%, and 90% at 1-week, 1-month, and 6-month post-procedural time points, respectively. Improved walking ability was achieved in 100% and 98% of cases at 1- and 6-month post-treatment time points [Citation26].
In a retrospective single-center study, Khan et al. [Citation24] successfully treated 102 vertebral lesions in 69 patients (multiple myeloma in 10 patients and metastases in 59 patients) and achieved statistically significant pain palliation at 2–4 week and 20–24 week post-procedural time points as well as local tumor control on post-treatment imaging at 20–24 week interval. The procedures were performed under general anesthesia with no thermal protection techniques and the investigators reported complications in 2 patients (S1 nerve thermal injury and skin burn).
In a retrospective study, Kastler et al. [Citation17] successfully treated 20 spinal metastases (17 patients) with microwave ablation (adjunct cementation in 9 cases) implementing thermo-protection in 4 cases. The authors reported pain relief in 16 of 17 patients with significant pain reduction at 1-day, 1-week, 1-month, 3-month, and 6-month post-ablation time points with no major complications.
Magnetic resonance imaging-guided high intensity focused ultrasound (HIFU) ablation
MRI–guided high intensity focused ultrasound (HIFU) combines the use of focused ultrasound energy to thermally ablate tissue with continuous MR imaging and thermal feedback [Citation19]. Focused ultrasound systems produce acoustic energy by using a piezoelectric transducer which operates at frequencies of 200 kHz–4 MHz, producing an intensity in the focal volume on the order of 100–10 000 W/cm2 [Citation19]. At these energy levels, the interaction between the focused ultrasound beams and biologic tissue results in a rise of cell temperature over the treated volume of tissue, leading to coagulative necrosis at a thermal range of 65–85 °C, depending on the tissue absorption coefficient [Citation19,Citation20]. Sonications are limited in duration to only a few seconds, thereby reducing the potentially detrimental effects of perfusion and blood flow on energy distribution. Consequently, the ablation of large regions requires tiling of multiple sonications to cover a larger volume and to create homogeneous thermal damage and coagulative necrosis of the target lesion [Citation19,Citation20]. For pain palliation, the concentration of acoustic energy on the intact surface of cortical bone rapidly creates a temperature increase that determines critical thermal damage to the adjacent periosteum; since the periosteum is the highest innervated component of mature bone, its ablation is an effective approach for pain management [Citation19,Citation20]. The major advantages of MR imaging–guided focused ultrasound include MR-guided three-dimensional visualization for precise treatment planning, real-time monitoring of thermal damage in the target zone with MR thermometry, continuous temperature mapping of treated tissue, immediate assessment of therapy, as well as the totally noninvasive nature of this technology [Citation19,Citation20].
In 2013, Napoli et al. [Citation19] reported successful management of 18 osseous metastases lesions with MR-guided HIFU ablation. There were no complications and statistically significant improvement in pain and BPI-Quality of Life scores [Citation19]. In a multi-center single-blind randomized phase III clinical trial [Citation20], Hurwitz et al randomly assigned 112 patients with osseous metastases to receive MR-guided HIFU ablation and 35 patients to the placebo group (MR-guided HIFU with sonication power off). MR-guided HIFU was superior to placebo in regards to improved Numerical Rating Scale for pain as well as improved quality of life based on the BPI-Quality of Life scoring system at the 3-month time point. Major complications included pathologic fractures in 2 patients, third-degree skin burn in 1 patient, and neuropathy in 1 patient [Citation20].
Patient selection algorithms
Treatment plans for patients with osseous metastases should be determined by a multidisciplinary team of radiation oncologists, medical oncologists, interventional radiologists, and oncologic surgeons.
Several organizations have published guidelines for the treatment of metastatic bone disease; however, to date, only the National Comprehensive Cancer Network (NCCN) has incorporated percutaneous ablation. The most recent NCCN guidelines for adult cancer pain (version 1.2019) state that ablation may be considered for palliation of metastatic bone pain without an oncologic emergency (e.g. pathologic fracture or epidural tumor) when pharmacologic therapy is inadequate and radiation therapy is contraindicated or not desired by the patient [Citation41].
The metastatic spine disease multi-disciplinary working group [Citation3], declared in their algorithms that percutaneous spinal thermal ablation is considered a valid option for the following patient subgroups: (1) Asymptomatic spinal metastases in patients with life expectancy more than 6 months, good performance status, and few visceral metastases, (2) Uncomplicated (lack of pathologic vertebral compression fracture and MESCC) painful spinal metastases, (3) Stable pathologic vertebral compression fracture in patients with life expectancy more than 6 months, good performance status, and few visceral metastases.
Osseous metastases are ablated for pain palliation and/or local tumor control when there is persistent pain or imaging tumor progression despite maximum radiation therapy, radiation therapy is contraindicated, or there is inadequate response to systemic therapies and analgesics, Combination of radiation therapy and thermal ablation is also considered for radiation-insensitive tumors [Citation3]. Cementation may be performed following thermal ablation for added pain relief or pathologic fracture prevention/stabilization [Citation12,Citation16,Citation21,Citation23–26]. It should be noted that thermal ablation is synergetic with bone consolidation and radiation therapy at least for pain palliation (and probably for local tumor control), and combination of radiation therapy, thermal ablation and bone consolidation results in more effective pain palliation [Citation29]. Cementation and osteosynthesis are the two available techniques to provide bone consolidation following thermal ablation, and the choice between these two techniques depends upon the biomechanics of the target bone [Citation42–44].
Patient performance status is typically assessed by widely used and validated Karnofsky performance status [Citation3]. Spinal instability is a relative contraindication for percutaneous thermal ablation depending upon severity. Spinal instability is determined using the spinal instability neoplastic score (SINS) [Citation45]. Scores range from 0 to 18, and higher scores indicate greater instability. Although there is no score cutoff to prompt surgery, surgical evaluation for potential tumor resection and/or stabilization is recommended for scores of 7 or higher [Citation46]. At our institutions, we treat patients with spinal instability who are not surgical candidates with vertebral augmentation, which does not entirely restore stability but relieves pain related to motion at fracture site. Surgery is the treatment of choice for spinal metastases complicated by central canal stenosis [Citation47]; however, in the absence of spinal cord compression, thermal ablation may be considered as an alternative for patients who are not surgical candidates. CT and MR imaging are used to determine if central canal stenosis is due to tumor alone or in combination with retropulsion of fracture fragments as ablation may arrest or cause retraction of epidural tumor but will not alleviate symptoms related to osseous central canal stenosis. Such patients are alternatively managed by epidural corticosteroid and long-acting anesthetic injections [Citation48].
Thermal ablation planning: general considerations, pre-ablation imaging and procedure guidelines
After multidisciplinary consensus is reached for a patient to undergo percutaneous minimally invasive therapy, pretreatment consultation with the patient is recommended and should include explanation of benefits, risks, potential complications, and alternative treatment options. Pretreatment consultation should include history and focused physical examination to re-confirm focal pain/tenderness at the tumor site and neurologic examination to assess for potential focal neurologic deficit. Laboratory tests are utilized to evaluate the coagulation status (platelet count more than 50 000 per microliter and INR less than 1.5 are generally acceptable) and at times, possibility of systemic infection (CBC, ESR, CRP, and cultures, if clinically indicated). Thermal ablation of bone and soft-tissue tumors is painful and requires moderate sedation or general anesthesia, particularly with technically challenging procedures in close proximity to critical structures, to minimize patient movement and potential subsequent complications. When ablating vertebral lesions, general anesthesia may be considered when motor/somatosensory evoked potential monitoring or electrostimulation is utilized to minimize potential neural thermal injury.
Percutaneous thermal ablation and cementation may be performed with fluoroscopic or CT guidance. Cone-beam volume CT images may be acquired with some biplane flat-panel detector fluoroscopy units, utilizing the strengths of both modalities.
Spinal RFA and vertebral augmentation are most commonly performed under fluoroscopic guidance which is readily available, provides near real-time guidance and affords efficient needle placement and immediate recognition of cement leakage. The main disadvantage of fluoroscopy is the often lack of visualization of the target lesion. Therefore, fluoroscopic images must be correlated with pre-procedural cross-sectional imaging to confirm that the target lesion is ablated. Furthermore, pre- and intra-procedural vertebral segment counting is critical to account for potential anatomic variations, such as supernumerary ribs and transitional lumbosacral anatomy. The main advantage of CT guidance is detailed cross-sectional visualization of relevant anatomy which affords accurate needle placement in the target lesion, as well as assessment for potential thermal protection. When ablating tumor along the posterior vertebral body with bipolar navigational RFA electrodes, it is often easier to confirm with CT that the electrode is no closer than 10 mm from the posterior vertebral body cortex, the maximum short-axis radius of RFA volume [Citation21].
Cryoablation is typically performed under CT guidance as the ablation zone is visualized as a hypo-attenuating ice ball in soft tissues, and at times within bone, confirming that the entire tumor is included in the cryoablated volume [Citation12,Citation13,Citation16]. Although microwave ablation zone is largely CT occult, this procedure is generally performed under CT guidance since microwave antennae typically generate larger ablation zones which mandates accurate placement within the target lesion [Citation17,Citation24,Citation26]. Conventional or cone-beam volume CT provides immediate cross-sectional localization of epidural or neuroforaminal cement leakage during vertebral augmentation. MRI is the guidance modality of choice for HIFU.
Tumor location, size and composition (osteolytic, osteoblastic, or mixed) are used to determine the choice of ablation modality. RFA is typically used for treatment of geometric osteolytic lesions (with small or no soft tissue component) whereas osteoblastic lesions are better treated with cryoablation and microwave ablation as RFA is rendered ineffective due to high impedance of sclerotic bone [Citation35]. Cryoablation and microwave ablation are appropriate choices for larger tumors with complex geometries, as multiple cryoprobes or antennae can be strategically arranged to produce a contiguous ablation zone with a tailored configuration [Citation13–16,Citation18,Citation26]. Anterior and posterolateral vertebral body lesions can be accessed using a transpedicular approach with traditional straight RF electrodes, cryoprobes, and microwave antennae whereas central posterior vertebral body tumors may only be accessed from a transpedicular approach with navigational RF electrodes [Citation21,Citation23]. Cryoablation is also preferred for treatment of spinal tumors with an extraosseous soft-tissue component as the cryoablated volume is identified on CT. MR-guided HIFU is not typically recommended for treatment of vertebral lesions due to increased risk of thermal injury compared to other ablation modalities.
Pre-procedure imaging includes dedicated weight bearing spine radiographs, CT, PET-CT, and MRI. Radiographs are used to determine the presence and extent of vertebral compression fractures, spinal alignment, integrity of osseous central canal, and suitability of fluoroscopy as imaging guidance. CT accurately localizes the target tumor and provides information regarding the anatomy of the bone and surrounding soft tissues such as nerves, spinal canal and torso vital organs for treatment planning. For example, CT evaluates the vertebral pedicles to ensure that the outer diameter of the introducing cannula can be accommodated. Otherwise, a partial lateral parapedicular approach may be considered which is often necessary when accessing thoracic vertebrae, the pedicles of which are typically tall and narrow [Citation49]. The main disadvantage of a parapedicular approach is the potential injury to the radicular arteries or the artery of Adamkiewicz which may arise from either side of any vertebral level between T8 and L3 [Citation50]. Cortical discontinuities caused by tumor or pathologic fracture clefts are readily identified with CT and may be a source of cement leakage during post-ablation cementation. MRI is most accurate to determine tumor extent and provides guidance for procedure planning. MRI is important for delineating the extent of the tumor, including the presence of marrow replacement and extra-osseous tumor extension that may be difficult to assess on CT, contributing to the patient’s pain, particularly in the spine and pelvis where pain can be difficult to localize.
In our practice, we strive to ablate the entire volume of MR hyperintensity on fluid sensitive sequences and enhancement, as well as an additional 3-mm margin to account for microscopic tumor spread [Citation51]. In cases of RFA, we routinely perform simultaneous bipedicular ablations to include the entire CTV in alignment with the consensus recommendations by the international spine radiosurgery consortium resulting in more thorough ablation of the vertebral body and pedicles, for improved local tumor control rates and more durable pain palliation [Citation23,Citation34].
The size and configuration of ablation zone for each specific applicator for a selected ablation modality is available based on validated manufacturer’s data and is utilized to determine the number of applicators and ablation cycles required to achieve optimal treatment [Citation10–32].
Thermal protection and thermal monitoring
When performing bone thermal ablation, the major inherent risks lie in potential injury to adjacent structures and soft tissues including spinal cord, nerves, articular cartilage, vessels, skin, and torso vital organs such as bowel.
Several parameters influence the extent and severity of potential neural thermal injury including absolute temperature, duration of thermal effect, distance from margins of ablation zone, presence or absence of intact osseous cortex, and type of nerve fiber [Citation40].
Multiple passive thermo-protective techniques are available and well described. Use of conscious sedation allows the benefit of biofeedback from the patient during the procedure, particularly for heat-based ablation. Complaints of sensory-neural deficits along an adjacent nerve distribution should prompt termination of the ablation and use of active thermo-protective techniques (e.g. CO2 insufflation and/or cooling/warming with fluid) to allow for continuation of the ablation.
Current practice to minimize potential neural and vital soft tissue thermal injury includes the use of thermal insulation, as well as temperature and neurophysiologic monitoring [Citation12,Citation25,Citation52,Citation53]. Thermal insulation may be achieved by hydro-dissection and instillation of warm or cool liquid which result in displacement of the structure at risk and modification of the temperature surrounding the structure at risk, respectively. Hydro-dissection during RFA should be performed using nonionic solutions such as 5% dextrose-in-water. Saline solutions should be avoided during RFA as the electric conductivity may result in expansion of ablation zone and creation of a plasma field [Citation25,Citation40]. Carbon dioxide injection of the perineural space, epidural space and/or neuroforamina may be used to dissect and actively insulate the neural structures [Citation12,Citation40]. In addition to insulation, continuous real-time and precise temperature monitoring (passive thermal protection) may be undertaken by placing thermocouples close to the threatened structures [Citation52,Citation53]. In clinical practice, active thermo-protection is initiated once the temperature reaches 45 °C (heat) and 10 °C (cold) [Citation52,Citation53].
Neurophysiologic monitoring and nerve electro-stimulation during thermal ablation have been performed using estimations of motor- and somatosensory-evoked potential amplitudes [Citation12,Citation52–54]. Substantial reduction in amplitude and/or latency of evoked potential amplitudes affords early detection of impending neurologic injury which should prompt active thermo-protection or modification during ablation.
Intra-articular tumors and lesions close to the articular surface/subchondral bone, should be approached with caution to minimize the possibility of inadvertent injury to the subchondral bone plate and overlying articular cartilage and potential articular surface collapse [Citation33,Citation55]. Thermal protection may be achieved by intra-articular injection of dextrose 5% in water or carbon dioxide [Citation33,Citation55].
Skin injury is also a potential complication of percutaneous thermal ablation. Accurate assessment of ablation zone boundaries minimizes the risk of skin injury. Active skin thermo-protection such as surface application of warm saline in a glove or soaked gauze during cryoablation should be implemented to minimize skin injury. Furthermore, attention should be paid to prevent retraction of the outer cannula in cryoablation and unipolar RFA systems. Using a bipolar RFA system inherently obviates the risk of skin burn, and utilization of wider and more grounding pads with unipolar RFA systems decreases the risk of skin injury.
Post-treatment imaging
Contrast-enhanced MRI is the modality of choice for post-treatment imaging of osseous metastases. In our practice, we perform a baseline study 6–8 weeks following treatment to allow inflammation to subside, unless requested earlier by another physician. Subsequent imaging is performed when patients report new or increasing pain. Ablation typically generates a cavity of coagulation necrosis surrounded by an inner rim of hemorrhagic congestion and outer rim of granulation tissue [Citation56,Citation57]. Over time, the rim of granulation tissue evolves into vascular fibrosis [Citation58,Citation59]. On MRI, the ablated volume is identified by the lack of enhancement. The signal intensity of the ablated volume varies depending upon relative combination of sclerotic bone, residual vascular and yellow marrow, and hemorrhagic products. The inner rim of hemorrhagic congestion contains hemosiderin and is T1 and T2 hypointense [Citation56]. The outer rim of granulation tissue or vascular fibrosis is T1 hypointense and T2 hyperintense enhancing tissue [Citation56,Citation58,Citation59]. The marrow outside the ablated volume in vertebral lesions typically demonstrates no enhancement as ablation frequently results in thrombosis of the vertebral vascular pedicle, particularly during ablation of posterior vertebral body lesions. Following vertebral augmentation, the cement produces hypointensity on all pulse sequences.
Widely used methods for evaluating treatment response, such as the Response Evaluation Criteria in Solid Tumors [Citation60] and World Health Organization Handbook for Reporting Results of Cancer Treatment [Citation61], are not applicable to ablations as these methods are based on maximum dimensional measurements without considering tumor necrosis. Therefore, adequacy of treatment is determined by comparing the volume of non-enhancing ablation cavity with tumor volume on pretreatment imaging. Residual or recurrent tumor is typically hyperintense tissue on fluid sensitive MR sequences with nodular enhancement along the margin of the ablation cavity. Granulation tissue or vascular fibrosis may demonstrate identical MRI characteristics, and vascular fibrosis may evolve for years following ablation, mimicking tumor progression [Citation58,Citation59]. In such cases, PET/CT or PET/MRI may provide information in differentiating hypermetabolic tumor from fibrosis, which is not typically metabolically hyperactive. Ultimately, when clinical evaluations and imaging findings of tumor progression are discordant, tissue sampling should be performed before initiation of further radiation therapy, surgery, or change in systemic chemotherapy.
Complications
Percutaneous thermal ablation has been shown to be safe for management of osseous metastases [Citation10–32]. The rate of major complications following bone cryoablation has been reported at 2.5% [Citation32]. Neural thermal injury to the spinal cord and nerves is the most important potential complication of percutaneous bone thermal ablation which may result in myelopathic and radicular symptoms, as well as motor deficits. The vast majority of neural thermal injury are transient and may be managed by perineural, trans-foraminal or epidural injection of steroids and long-acting anesthetics. There remains risk of injury to torso vital organs such as bowel which may be minimized by the use of thermal protection techniques. Following thermal ablation, there is potential bone weakening, and particularly in ablation of tumors with large size or anterior vertebral body or pedicle lesions, there is a potential risk of ablation-related fracture which may be minimized with cement augmentation. Cement leakage into the central canal/epidural space or neuroforamina may worsen pain related to central canal or neuroforaminal stenosis, or even result in spinal cord compression. Thermal spinal cord injury may also be caused by exothermic polymerization of extruded cement. Additionally, cement pulmonary embolism caused by leakage into the caval or azygous venous systems should be recognized. There also remains a risk of thermal skin injury.
Summary
Minimally invasive percutaneous thermal ablation of osseous metastases has proved safe and effective in management of selected patients with bone metastatic disease. These procedures have become a part of the treatment algorithm for certain subgroup of patients with osseous metastases to achieve pain palliation and/or local tumor control. Special attention to the details of procedure techniques including choice of ablation modality, thermal protection, adequacy of treatment, and post-ablation imaging will result in improved patient outcomes.
Ethical statement
This review article was approved by the Mallinckrodt Institute of Radiology IRB. Informed consent was not obtained (waived) for this retrospective review article in accordance with the IRB guidelines.
Disclosure statement
Jack W. Jennings MD PhD: Consulting Fee or Honorarium: Medtronic and Galil Medical. Anderanik Tomasian MD: No conflict of interest.
References
- Mundy GR. Metastasis to bone: causes, consequences and therapeutic opportunities. Nat Rev Cancer. 2002;2:584–593.
- Urch C. The pathophysiology of cancer-induced bone pain: current understanding. Palliat Med. 2004;18:267–274.
- Wallace AN, Robinson CG, Meyer J, et al. The metastatic spine disease multidisciplinary working group algorithms. Oncologist. 2015;20:1205–1215.
- Deschavanne PJ, Fertil B. A review of human cell radiosensitivity in vitro. Int J Radiat Oncol Biol Phys. 1996;34:251–266.
- Rofstad EK. Radiation biology of malignant melanoma. Acta Radiol Oncol. 1986;25:1–10.
- Strander H, Turesson I, Cavallin-Ståhl E. A systematic overview of radiation therapy effects in soft tissue sarcomas. Acta Oncol. 2003;42:516–531.
- van der Linden YM, Steenland E, van Houwelingen HC, et al. Patients with a favourable prognosis are equally palliated with single and multiple fraction radiotherapy: results on survival in the Dutch bone metastasis study. Radiother Oncol. 2006;78:245–253.
- Rosenthal D, Callstrom MR. Critical review and state of the art in interventional oncology: benign and metastatic disease involving bone. Radiology. 2012;262:765–780.
- Hara S. Opioids for metastatic bone pain. Oncology. 2008;74:52–54.
- Dupuy DE, Liu D, Hartfeil D, et al. Percutaneous radiofrequency ablation of painful osseous metastases: a multicenter American College of Radiology Imaging Network trial. Cancer. 2010;116:989–997.
- Goetz MP, Callstrom MR, Charboneau JW, et al. Percutaneous image-guided radiofrequency ablation of painful metastases involving bone: a multicenter study. J Clin Oncol. 2004;22:300–306.
- Tomasian A, Wallace A, Northrup B, et al. Spine cryoablation: pain palliation and local tumor control for vertebral metastases. AJNR Am J Neuroradiol. 2016;37:189–195.
- Callstrom MR, Dupuy DE, Solomon SB, et al. Percutaneous image-guided cryoablation of painful metastases involving bone: multicenter trial. Cancer. 2013;119:1033–1041.
- McMenomy BP, Kurup AN, Johnson GB, et al. Percutaneous cryoablation of musculoskeletal oligometastatic disease for complete remission. J Vasc Interv Radiol. 2013;24:207–213.
- Prologo JD, Passalacqua M, Patel I, et al. Image-guided cryoablation for the treatment of painful musculoskeletal metastatic disease: a single center experience. Skeletal Radiol. 2014;43:1551–1559.
- Wallace AN, McWilliams SR, Connolly SE, et al. Percutaneous image-guided cryoablation of musculoskeletal metastases: pain palliation and local tumor control. J Vasc Interv Radiol. 2016;27:1788–1796.
- Kastler A, Alnassan H, Aubry S, et al. Microwave thermal ablation of spinal metastatic bone tumors. J Vasc Interv Radiol. 2014;25:1470–1475.
- Callstrom MR, Atwell TD, Charboneau JW, et al. Painful metastases involving bone: percutaneous image-guided cryoablation-prospective trial interim analysis. Radiology. 2006;241:572–580.
- Napoli A, Anzidei M, Marincola BC, et al. Primary pain palliation and local tumor control in bone metastases treated with magnetic resonance-guided focused ultrasound. Invest Radiol. 2013;48:351–358.
- Hurwitz MD, Ghanouni P, Kanaev SV, et al. Magnetic resonance-guided focused ultrasound for patients with painful bone metastases: phase III trial results. J Natl Cancer Inst. 2014;23:106.
- Anchala PR, Irving WD, Hillen TJ, et al. Treatment of metastatic spinal lesions with a navigational bipolar radiofrequency ablation device: a multicenter retrospective study. Pain Phys. 2014;17:317–327.
- Bagla S, Sayed D, Smirniotopoulos J, et al. Multicenter prospective clinical series evaluating radiofrequency ablation in the treatment of painful spine metastases. Cardiovasc Intervent Radiol. 2016;39:1289–1297.
- Tomasian A, Hillen TJ, Chang RO, et al. Simultaneous bipedicular radiofrequency ablation combined with vertebral augmentation for local tumor control of spinal metastases. AJNR Am J Neuroradiol. 2018;39:1768–1773.
- Khan MA, Deib G, Deldar B, et al. Efficacy and safety of percutaneous microwave ablation and cementoplasty in the treatment of painful spinal metastases and myeloma. AJNR Am J Neuroradiol. 2018;39:1376–1383.
- Wallace AN, Tomasian A, Vaswani D, et al. Radiographic local control of spinal metastases with percutaneous radiofrequency ablation and vertebral augmentation. AJNR Am J Neuroradiol. 2016;37:759–765.
- Pusceddu C, Sotgia B, Fele RM, et al. Combined microwave ablation and cementoplasty in patients with painful bone metastases at high risk of fracture. Cardiovasc Intervent Radiol. 2016;39:74–80.
- Deschamps F, Farouil G, Ternes N, et al. Thermal ablation techniques: a curative treatment of bone metastases in selected patients? Eur Radiol. 2014;24:1971–1980.
- Luigi Cazzato R, Auloge P, De Marini P, et al. Percutaneous image-guided ablation of bone metastases: local tumor control in oligometastatic patients. Int J Hyperthermia. 2018;35:493–499.
- Cazzato RL, Garnon J, Caudrelier J, et al. Percutaneous radiofrequency ablation of painful spinal metastasis: a systematic literature assessment of analgesia and safety. Int J Hyperthermia. 2018;34:1272–1281.
- David E, Kaduri S, Yee A, et al. Initial single center experience: radiofrequency ablation assisted vertebroplasty and osteoplasty using a bipolar device in the palliation of bone metastases. Ann Palliat Med. 2017;6:118–124.
- Cazzato RL, Garnon J, Caudrelier J, et al. Low-power bipolar radiofrequency ablation and vertebral augmentation for the palliative treatment of spinal malignancies. Int J Hyperthermia. 2018;34:1282–1288.
- Auloge P, Cazzato RL, Rousseau C, et al. Complications of percutaneous bone tumor cryoablation: a 10-year experience. Radiology. 2019;291:521–528.
- Rybak L. Fire and ice: thermal ablation of musculoskeletal tumors. Radiol Clin North Am. 2009;47:455–469.
- Cox BW, Spratt DE, Lovelock M, et al. International Spine Radiosurgery Consortium consensus guidelines for target volume definition in spinal stereotactic radiosurgery. Int J Radiat Oncol Biol Phys. 2012;83:e597–605.
- Singh S, Saha S. Electrical properties of bone. A review. Clin Orthop Relat Res. 1984;186:249–271.
- Dupuy DE, Hong R, Oliver B, et al. Radiofrequency ablation of spinal tumors: temperature distribution in the central canal. Am J Roentgenol. 2000;175:1263–1266.
- Gage AA, Baust JG. Cryosurgery for tumors. J Am Coll Surg. 2007;205:342–356.
- Weld KJ, Landman J. Comparison of cryoablation, radiofrequency ablation and high- intensity focused ultrasound for treating small renal cell tumors. BJU Int. 2005;96:1224–1229.
- Campbell SC, Krishnamurthi V, Chow G, et al. Renal cryosurgery: experimental evaluation of treatment parameters. Urology. 1998;52:29–33.
- Tsoumakidou G, Koch G, Caudrelier J, et al. Image-guided spinal ablation: a review. Cardiovasc Intervent Radiol. 2016;39:1229–1238.
- National Comprehensive Cancer Network. Adult Cancer Pain (Version 1.2019); 2019 [cited 2019 Feb 22].
- Cazzato RL, Arrigoni F, Boatta E, et al. Percutaneous management of bone metastases: state of the art, interventional strategies and joint position statement of the Italian College of MSK Radiology (ICoMSKR) and the Italian College of Interventional Radiology (ICIR). Radiol Med. 2019;124:34–49.
- Cazzato RL, Garnon J, Shaygi B, et al. Percutaneous consolidation of bone metastases: strategies and techniques. Insights Imag. 2019;10:14–16.
- Cazzato RL, Koch G, Buy X, et al. Percutaneous image-guided screw fixation of bone lesions in cancer patients: double-centre analysis of outcomes including local evolution of the treated focus. Cardiovasc Intervent Radiol. 2016;39:1455–1463.
- Fisher CG, DiPaola CP, Ryken TC, et al. A novel classification system for spinal instability in neoplastic disease: an evidence-based approach and expert consensus from the Spine Oncology Study Group. Spine. 2010; 35:E1221–E1229.
- Fourney DR, Frangou EM, Ryken TC, et al. Spinal instability neoplastic score: an analysis of reliability and validity from the spine oncology study group. J Clin Oncol. 2011;29:3072–3077.
- Patchell RA, Tibbs PA, Regine WF, et al. Direct decompressive surgical resection in the treatment of spinal cord compression caused by metastatic cancer: a randomised trial. Lancet. 2005;366:643–648.
- Wallace AN, Greenwood TJ, Jennings JW. Use of imaging in the management of metastatic spine disease with percutaneous ablation and vertebral augmentation. Am J Roentgenol. 2015;205:434–441.
- Mathis JM, Golovac S. Image-guided spine interventions,2nd ed. New York, NY: Springer; 2010. pp. 2–5.
- Charles YP, Barbe B, Beaujeux R, et al. Relevance of the anatomical location of the Adamkiewicz artery in spine surgery. Surg Radiol Anat. 2011;33:3–9.
- Lee YK, Bedford JL, McNair HA, et al. Comparison of deliverable IMRT and VMAT for spine metastases using a simultaneous integrated boost. Br J Radiol. 2013;86:20120466.
- Buy X, Tok C-H, Szwarc D, et al. Thermal protection during percutaneous thermal ablation procedures: interest of carbon dioxide dissection and temperature monitoring. Cardiovasc Intervent Radiol. 2009;32:529–534.
- Tsoumakidou G, Garnon J, Ramamurthy N, et al. Interest of electrostimulation of peripheral motor nerves during percutaneous thermal ablation. Cardiovasc Intervent Radiol. 2013;36:1624–1628.
- Kurup AN, Morris JM, Boon AJ, et al. Motor evoked potential monitoring during cryoablation of musculoskeletal tumors. J Vasc Interv Radiol. 2014;25:1657–1664.
- Friedman MV, Hillen TJ, Wessell DE, et al. Hip chondrolysis and femoral head osteonecrosis: a complication of periacetabular cryoablation. J Vasc Interv Radiol. 2014;25:1580–1588.
- Lee JM, Choi SH, Park HS, et al. Radiofrequency thermal ablation in canine femur: evaluation of coagulation necrosis reproducibility and MRI-histopathologic correlation. Am J Roentgenol. 2005;185:661–667.
- Yoshimoto Y, Azuma K, Miya A, et al. A fundamental study of cryoablation on normal bone: diagnostic imaging and histopathology. Cryobiology. 2014;69:229–235.
- Gervais DA, Arellano RS. Percutaneous tumor ablation for hepatocellular carcinoma. Am J Roentgenol. 2011;197:789–794.
- Sainani NI, Gervais DA, Mueller PR, et al. Imaging after percutaneous radiofrequency ablation of hepatic tumors.Part 1.Normal findings. Am J Roentgenol. 2013;200:184–193.
- Eisenhauer EA, Therasse P, Bogaerts J, et al. New response evaluation criteria in solid tumours: revised RECIST guideline (version 1.1). Eur J Cancer. 2009;45:228–247.
- World Health Organization. WHO handbook for reporting results of cancer treatment. WHO offset publication no. 48. Geneva, Switzerland: World Health Organization. 1979.