Abstract
Purpose: To evaluate whether local tumor progression (LTP) would be further reduced when contrast-enhanced ultrasound (CEUS)-CT/MR fusion imaging was used as intraprocedural assessment method in hepatocellular carcinoma (HCC) thermal ablation compared with routine CEUS.
Materials and methods: This prospective non-randomized study was conducted from December 2010 to July 2012. CEUS-CT/MR fusion imaging and routine CEUS were used for treatment response assessment in the ablation procedure of 146 HCCs and 122 HCCs, respectively. Supplementary ablations were performed immediately if necessary. The primary technique efficacy rate, LTP rate and overall survival (OS) rate were calculated.
Results: For CEUS-CT/MR fusion imaging and routine CEUS, the technical success rate, technique efficacy rate and supplementary ablation rate were 86.3% (126/146) and 98.4% (120/122) (p = .000), 99.2% (125/126) and 94.2% (113/120) (p = .032), and 14.3% (18/126) and 4.2% (5/120) (p = .006), respectively. The cumulative LTP rate and OS rate were not significantly different between fusion imaging group and routine CEUS group. However, for lesions that were larger than 3 cm or close to major vessels (41 lesions in fusion imaging group and 44 lesions in routine CEUS group, who received transcatheter arterial chemoembolization before ablation), the cumulative LTP rate was significantly lower in fusion imaging group than in routine CEUS group (p = .032).
Conclusion: Although intraprocedural CEUS-CT/MR fusion imaging has certain limitations in application, it might provide a potential more efficient method compared with routine CEUS in reducing LTP in HCC thermal ablation, especially for difficult ablation lesions.
Introduction
For hepatocellular carcinoma (HCC), thermal ablation is increasingly used as a first-choice treatment [Citation1–3]. Intraprocedural imaging assessment is critical for thermal ablation as it helps to improve the treatment effect by assessing treatment response and guiding necessary supplementary ablations timely in the treatment procedure.
Contrast-enhanced ultrasound (CEUS) is now commonly used for intraprocedural assessment during the thermal ablation of HCC as it is real-time, accessible, required no radiation and no renal toxicity. However, it has some shortcomings. First, CEUS tends to be less accurate in intraprocedural residual tumor detection [Citation4–10]. The hyper-enhanced area frequently observed around the ablation zone in the arterial phase of CEUS, caused by inflammatory reaction and tissue hyperemia after thermal ablation, might obscure results of residual tumors. What’s more, it is difficult to assess ablative margin (AM) using routine CEUS as both the ablated index tumor and the ablated liver tissue appear as perfusion defects on CEUS images. According to the previous reports, there is a 3.2%–24.6% probability that local tumor progression (LTP) will occur even after complete ablation of the lesion [Citation11–14], and failure to establish a sufficient AM was believed an important independent risk factor for LTP [Citation15–19]. Thus, to reduce the incidence of LTP, an intraprocedural imaging assessment method with the ability to quickly and accurately evaluate AM is necessary.
Ultrasound (US)-CT/MR fusion imaging can possibly resolve these issues [Citation20–22]. It integrates US images and corresponding CT/MR planar images together in real-time on one monitor and has been widely used for puncture guidance [Citation23–25]. We combined US-CT/MR fusion imaging with CEUS and projected the CT/MR images of the index tumor onto the perfusion defect on CEUS after the ablation procedure. By observing such spatial relationships, the success of complete tumor ablation could be determined intuitively. Moreover, the AM’s size could also be determined by comparing the boundaries of the index tumor and the ablation zone.
At our department, model experiments and preliminary clinical studies on CEUS-CT/MR fusion imaging have been performed, and the feasibility and accuracy of fusion imaging in treatment response assessment for HCC thermal ablation were initially verified [Citation26–28]. There were also several reports on CEUS-CT/MR fusion imaging for treatment response assessment for HCC ablation [Citation29–31]; nevertheless, none of the reports have compared CEUS-CT/MR fusion imaging with routine CEUS in intraprocedural assessment for HCC thermal ablation. To evaluate whether local efficacy would be further improved when CEUS-CT/MR fusion imaging was used as intraprocedural assessment method in HCC thermal ablation compared with routine CEUS, we conducted this study.
Materials and methods
Patients and tumors
Our study was performed with the approval of our institutional review board. It was conducted in accordance with the Declaration of Helsinki with written informed consent sought from each patient. From December 2010 to July 2012, patients diagnosed with HCC who intended to receive US-guided thermal ablation at our department were enrolled. The diagnosis of HCC was established based on AASLD guidance [Citation1]. The inclusion criteria were as follows: (I) 18–80 years old; (II) eligible for curative radiofrequency ablation (RFA) or microwave ablation (MWA) (≤5 nodules with a maximum diameter ≤7 cm, nodular-type lesion without tumor thrombosis in the portal vein or hepatic vein branches, no adjacent organ invasion or distal metastasis); and (III) well-preserved liver function (Child-Pugh A or B class, platelets count >50 × 109/L, PT prolonged <3 s). The exclusion criteria were as follows: (I) variceal bleeding within a month; (II) uncontrolled ascites; (III) cachexia; (IV) active infection, particularly of the biliary system; and (V) contraindications of CEUS agent; (VI) no contrast-enhanced CT/MR (CECT/MR) images taken ≤2 months before ablation.
Grouping
CEUS-CT/MR fusion imaging and routine CEUS were used for intraprocedural assessment alternately every week. According to the different intraprocedural assessment method, patients were separated into fusion imaging group and routine CEUS group.
Treatment procedures
Operators
Thermal ablations of patients in the two groups were randomly performed by three operators having >5 years’ experience in HCC ablation; all operators were proficient in operating the CEUS-CT/MR fusion imaging and routine CEUS assessments.
Equipment and agent
The cool-tip radiofrequency system (Covidien, Mansfield, MA, USA) with a single electrode (3 cm long exposed tip); KY-2000 microwave generator of 2450 MHz (Kangyou Corp., Nanjing, China) with an internally cooled microwave antenna; and the MyLab 90/Twice (Esaote, Genoa, Italy) ultrasound unit with convex array transducer CA431/CA541 (1–8 MHz) were used. The ultrasound unit MyLab Twice was equipped with the Virtual Navigator program, and both ultrasound units were equipped with real-time contrast-tuned imaging technique (CnTI, mechanical index <0.05). Moreover, the GE LightSpeed QX/i CT scanner with slice thicknesses of 5 mm was used with iopromide or iopamidol acting as the contrast agent. We also used the GE SIGNA TWINSPEED1.5 MR scanner with slice thicknesses of 2.5 mm and gadopentetate dimeglumine as the contrast agent. SonoVue (Bracco, Milan, Italy) was used as the contrast agent for CEUS (antecubital vein administration, 2.4 ml rapid bolus injection followed by 5 ml saline solution).
Ablation method
Based on tumor size, location and coagulation function, either RFA or MWA was selected. Small tumor size, location adjacent to critical structures (such as diaphragm, gastrointestinal tract, gall bladder or main biliary duct brunch) and a well-preserved coagulation function prompted our selection of RFA. When the tumor was large, no critical structures located adjacent to it were observed, or the coagulation function of the patient was poor, we preferred using MWA. If the tumor was ≥3 cm in diameter or was close to major vessels, transcatheter arterial chemoembolization (TACE) was performed 1–2 weeks before ablation. To spare adjacent critical structures, hydrodissection (creation of artificial ascites or pleural effusion) was performed.
Ablation process
All thermal ablation therapies were performed under general anesthesia. First, the index tumor was ablated as planned. Some effort was made to ablate the whole tumor and at least 5 mm of AM. Then, intraprocedural CEUS-CT/MR fusion imaging or routine CEUS assessment was performed. According to the assessment result, supplementary ablation was performed immediately when necessary. The same kind of imaging assessment was then performed again. This process was repeated until the ablation zone was deemed satisfactory.
Intraprocedural treatment response assessment and supplementary ablation
CEUS-CT/MR fusion imaging group
In previous studies, detailed procedures of CEUS-CT/MR fusion imaging assessment have been described [Citation27,Citation28]. Before the treatment procedure, selection of CT/MR series, data transfer and tumor depiction were performed. A 5 mm AM was depicted automatically by the system around the tumor in a different color. Co-registration was performed 5–10 min after ablation (after vaporization had weakened). The time spent on co-registration was recorded. Then, CnTI mode was started (mechanical index <0.05) and the contrast agent was administered. The real-time CEUS image was displayed in the overlap mode with the CT/MR image. To aid in co-registration and observation, the breathing of the patient, which was controlled by the breathing machine, was stopped at end expiration for 2 min at the most. The assessment results were divided into three categories based on the positional relationship between the overlaid tumor with a 5 mm AM and the perfusion defect on CEUS: (I) complete ablation of tumor and adequate AM (minimal AM ≥ 5 mm); (II) complete ablation of tumor but inadequate AM (minimal AM <5 mm); (III) incomplete ablation of the tumor. Tumors of category II or III immediately had supplementary ablations under CEUS-CT/MR fusion imaging’s guidance until they could be defined as category I (). For some tumors in category II, supplementary ablations were canceled because of insufficient liver function reserves or if the supplementary ablation was very risky.
Figure 1. A case using CEUS-MR fusion imaging for intraprocedural treatment response assessment. (a) shows the co-registration process: a1 shows the overlapped US and MR images; the left branch of the portal vein is chosen as an anatomical landmark; a2 shows the corresponding MR image. (b) shows the first CEUS-MR fusion imaging assessment: b1 shows the overlapped CEUS and MR images after the administration of the contrast agent; AM is inadequate (arrow); b2 shows the corresponding MR image with the tumor outlined in blue and the AM in purple. (c) shows the process of supplementary ablation: c1 shows that the electrode is inserted to the site of inadequate AM (arrow) under the guidance of US-MR fusion imaging; c2 shows the corresponding MR image. (d) shows the second CEUS-MR fusion imaging assessment: d1 shows the overlapped CEUS and MR images; adequate AM is obtained (arrow) after the supplementary ablation; d2 shows the corresponding MR image.
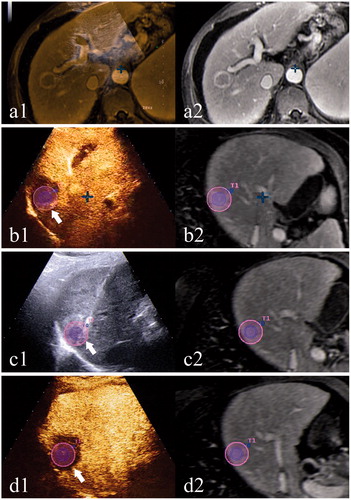
Routine CEUS group
CEUS was performed 5–10 min after ablation. The contrast agent was administered and ultrasound probe was moved across the ablation zone during arterial, portal venous and late phase for observation. The assessment results were divided into two categories based on whether there was characteristic tumor enhancement (usually hyperenhancement in arterial phase and hypoenhancement in portal venous or late phase [Citation32]) in or adjacent to the coagulated area: (I) complete ablation of tumor (without characteristic tumor enhancement) and (II) incomplete ablation of tumor (with characteristic tumor enhancement). Tumors of category II underwent immediate supplementary ablations under the guidance of routine CEUS until they could be defined as category I. AM was not evaluated in routine CEUS group because routine CEUS cannot accurately compare the position and range of the index tumor and the ablation zone.
Treatment evaluation by CECT/MR after ablation
CECT/MR was performed for all patients to evaluate the treatment effect about 1 month after ablation. By the consensus of two radiologists, who had >10 years of experience and were blinded to the patient’s grouping, an agreement on diagnosis was reached. ‘Primary technique efficacy’ was achieved when the macroscopic tumor was completely ablated according to 1-month CECT/MR. Patients with residual tumors were made to receive further treatment. Major complications related to ablation were recorded.
Follow-up
For the follow-up, CEUS examination and serum alpha-fetoprotein (AFP) test were performed every 3 months, and CECT/MR images were obtained every 6 months. If there was suspected tumor recurrence on CEUS, CECT/MR was performed. The imaging criterion for LTP was the characteristic enhancement pattern’s presence at the edge of the ablation zone (hypervascularization in arterial phase and wash-out pattern in portal and delayed phase) on CECT/MR.
Statistical analysis
Statistical analysis was performed using SPSS for Microsoft Windows (version 22.0, SPSS, Chicago, IL, USA). The cumulative LTP rate and overall survival (OS) rate were estimated using the Kaplan–Meier method with the comparison of two groups being analyzed by the log-rank test. Measurement data were described as means ± SD and analyzed using the t-test. The categorical variable was analyzed by chi-square test. A p value of <.05 was considered to be statistically significant.
Results
From December 2010 to July 2012, 207 patients with a total of 268 HCC tumors were treated with thermal ablation at our apartment according to the inclusion and exclusion criteria. 114 patients with 146 tumors were designated to use CEUS-CT/MR fusion imaging for intraprocedural assessment and 93 patients with 122 tumors were designated to use routine CEUS.
The implementation of intraprocedural CEUS-CT/MR fusion imaging and routine CEUS assessment
Sixteen patients who had 20 tumors failed in intraprocedural CEUS-CT/MR fusion imaging assessment and were evaluated using routine CEUS instead. The technical success rate was 86.3% (126/146). summarizes the reasons for failure in CEUS-CT/MR fusion imaging assessment. One patient with two tumors failed in intraprocedural routine CEUS assessment because of poor CEUS image quality and the technical success rate was 98.4% (120/122). The difference in the technical success rates of the two methods was significant (p = .000).
Table 1. Reasons for failure in CEUS-CT/MR fusion imaging assessment.
Baseline characteristics of two groups
After excluding patients with failed procedures, 190 patients with 246 tumors were finally enrolled in this prospective study, of which 98 patients with 126 tumors were in the CEUS-CT/MR fusion imaging group, and 92 patients with 120 tumors were in the routine CEUS group. There was no significant difference in the baseline characteristics between the two groups ().
Table 2. Baseline characteristics of study population.
Intraprocedural treatment response assessment and supplementary ablation
A total of 14.3% (18/126) and 4.2% (5/120) of the lesions underwent supplementary ablations in the thermal ablation procedure in the fusion imaging group and routine CEUS group, respectively (p = .006). After the final intraprocedural assessments, complete ablation was believed to have been achieved for all lesions in both groups, and for lesions in the fusion imaging group, 65.9% (83/126) got adequate AM (minimal AM ≥ 5 mm) while 34.1% (43/126) did not (minimal AM <5 mm). Reasons for inadequate AM were summarized ().
Table 3. Reasons for inadequate AM in CEUS-CT/MR fusion imaging group.
The time spent for co-registration was 4.9 ± 2.0 min (mean ± SD) in a range of 3–13 min. The total time spent for intraprocedural CEUS-CT/MR fusion imaging assessment including the time for gas dissipating, co-registration, contrast agent injection and observation was about 20 min.
Treatment evaluation by CECT/MR after ablation and major complications
According to CECT/MR after ablation, one tumor in fusion imaging group and seven tumors in routine CEUS group got incompletely ablated and the primary efficacy rate was 99.2% (125/126) and 94.2% (113/120), respectively (p = .032).
Subsequent treatments of residual tumors: The only one residual tumor in the fusion imaging group was treated with TACE. For the seven residual tumors in the routine CEUS group, four were treated with RFA, one with TACE, one with TACE and MWA, and one with TACE and percutaneous ethanol injection (PEI). All of these residual tumors were successfully treated in the second treatment session.
The major complication rate of fusion imaging group and routine CEUS group was 2.0% (2/98, 1 hemothorax and 1 massive ascites) and 3.3% (3/92, 1 hemothorax and 2 upper digestive tract hemorrhages), respectively. The difference in major complication rate was not significant (p = .675).
Follow-up
Follow-up was conducted until March 2017, and the median follow-up time was 44 months (range 1–75 months). During follow-up, a total of 11 and 13 LTPs occurred in the fusion imaging group and routine CEUS group, respectively. In the fusion imaging group, of the lesions ablated with adequate AM 6 LTPs occurred, and of the lesions with inadequate AM 5 LTPs occurred.
In the fusion imaging group, the cumulative LTP rate was significantly lower in lesions with adequate AM than in lesions with inadequate AM in the first 21 months (p = .028); the difference became insignificant after that period (, ). However, the cumulative LTP rate curves were found with no significant difference between fusion imaging group and routine CEUS group (p = .316, , ).
Figure 2. Cumulative local tumor progression rate curves. (a) Cumulative local tumor progression rate curves of lesions with adequate and inadequate AM in fusion imaging group. The difference was significant by the first 21 months (p = .028) but was not significant as a whole (p = .447). (b) Cumulative local tumor progression rate curves of two groups. The difference was not significant as a whole (p = .316). (c) Cumulative local tumor progression rate curves of lesions of two groups that didn’t receive TACE therapy 1–2 weeks before ablation. The difference was not significant as a whole (p = .549). (d) Cumulative local tumor progression rate curves of lesions of two groups that received TACE therapy 1–2 weeks before ablation. The difference was significant as a whole (p = .032).
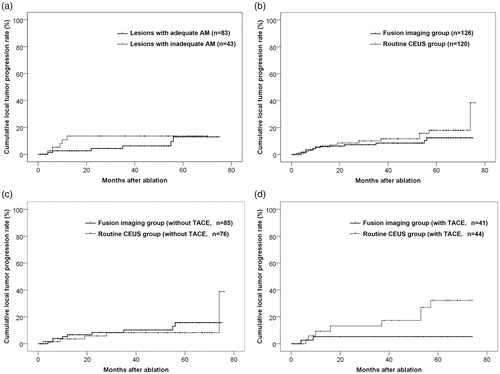
Table 4. Cumulative local tumor progression rate of each year.
For tumors of the two groups that didn’t receive TACE therapy before ablation (68 patients with 85 lesions in fusion imaging group and 62 patients with 76 lesions in routine CEUS group), the difference in cumulative LTP rate was insignificant (p = .549, ). For tumors that received TACE therapy before ablation (30 patients with 41 lesions in fusion imaging group and 30 patients with 44 lesions in routine CEUS group), the cumulative LTP rate was significantly lower in the fusion imaging group than in the routine CEUS group (p = .032, ).
The OS rate curves of two groups and OS rate of each year were shown (, ). The difference in OS rate between the two groups was insignificant (p = .892).
Figure 3. Overall survival rate curves. (a) Overall survival rate curves of two groups. The difference was not significant as a whole (p = .892). (b) Overall survival rate curves of patients of two groups that received TACE therapy 1–2 weeks before ablation. The difference was not significant as a whole (p = .901).
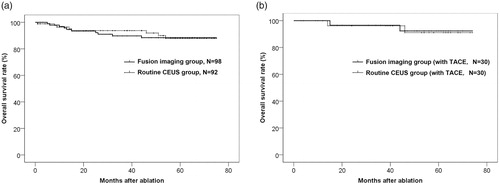
Table 5. Overall survival rate of each year.
The OS rate curves of patients of two groups that received TACE therapy 1–2 weeks before ablation were shown (). The difference was insignificant (p = .901).
Discussion
Compared with routine CEUS, the advantage of CEUS-CT/MR fusion imaging for treatment response assessment in thermal ablation therapy is the ability to virtually show the original tumor. Thus, the spatial relationship between the original tumor and ablation zone can be visualized and AM can be evaluated. This makes CEUS-CT/MR fusion imaging potentially more objective and accurate compared to routine CEUS in assessing treatment response in HCC thermal ablation.
Indeed, the complete ablation rate was further improved in the fusion imaging group compared to the routine CEUS group. This could be attributed to the more effective decision-making for supplementary ablation for residual tumors according to the CEUS-CT/MR fusion imaging assessment. As the spatial relationship between the original tumor and the ablation zone cannot be displayed on routine CEUS, the diagnosis of tumor residue by routine CEUS is mainly based on the appearance of characteristic tumor enhancement, which depends on the experience of an individual surgeon a lot and is prone to be less accurate.
In the fusion imaging group, the cumulative LTP rate was significantly lower in lesions with adequate AM than in lesions with inadequate AM by the first 21 months. This result is consistent with previous studies and confirms indirectly the accuracy of intraoperative CEUS-CT/MR fusion imaging in AM evaluation [Citation27,Citation28]. It also suggests us that LTP after HCC thermal ablation might be reduced if AM could be further improved under the guidance of CEUS-CT/MR fusion imaging. The reason that the difference in cumulative LTP rate became insignificant after 21 months might be that most LTPs tend to develop in the first 2 years and some of the ‘LTPs’ diagnosed later might be just intrahepatic recurrences that inadvertently occurred close to the lesion [Citation33–35].
As CEUS-CT/MR fusion imaging was used to improve AM, we expected a lower cumulative LTP rate in the fusion imaging group than in the routine CEUS group. However, we didn’t observe a significant difference in LTP rate, for which there are two plausible explanations. First, inadequate AM is not the only independent risk factor for LTP. According to the literature, there were several factors that might influence LTP rate apart from AM, such as tumor size, tumor location (e.g., close to large vessels or not), pathological stage and single/multiple nodules [Citation11,Citation14,Citation17–19,Citation36–39]. As a non-randomized controlled research, there was possibility that these factors might bias the results. Second, although supplementary ablations were conducted for inadequate AM under fusion imaging guidance in fusion imaging group, there were still 34.1% of the lesions ended up with inadequate AM for multiple reasons. As AM could not be evaluated in routine CEUS group, the percentage of inadequate AM in routine CEUS group was unknown. Therefore, there was possibility that the difference in AM between two groups might be not significant enough to make a difference in LTP rate. According to the study, the existence of large vessels or critical structures adjacent to the tumor was the primary reason for inadequate AM as further expansion of the ablation zone was limited for safety. To further improve the AM, a combination of thermal ablation and other treatment modalities (such as ethanol ablation) might be tried in future studies.
We calculated the cumulative LTP rates of tumors that received and didn’t receive TACE therapy before ablation separately and found that: for the former, the cumulative LTP rate was significantly lower in fusion imaging group than in routine CEUS group; for the latter, the difference between two groups was not significant. This result suggests that intraprocedural CEUS-CT/MR fusion imaging assessment might be more valuable for tumors that required complicated ablation. Tumors that received TACE therapy (≥3 cm in diameter or close to major vessels) are difficult ones to ablate as either multiple needle insertions are needed or expansion of the ablation zone is limited. For these tumors, the control of the ablation range is more difficult and the role of intraprocedural assessment becomes more critical.
According to the previous studies, initial treatment response (complete ablation, no LTP) is essential to improve the survival rate of HCC ablation [Citation40,Citation41]. In this study, the OS rate was not significantly increased in fusion imaging group, suggesting that the role of intraprocedural fusion imaging assessment on improving the efficacy of HCC thermal ablation might be limited. To further explore the impact of intraprocedural fusion imaging evaluation on survival, rigorous randomized controlled trials with larger sample size are needed.
According to our study, intraprocedural CEUS-CT/MR fusion imaging assessment is feasible in operation. The time spent for image registration was about 5 min and the total time required for one CEUS-CT/MR fusion imaging assessment, including the time for gas dissipating, co-registration, contrast agent injection and observation, was about 20 min, which is acceptable in practice. Furthermore, there was no significant increase in major complications, although additional supplementary ablations were conducted for inadequate AM in the fusion imaging group. However, we also noticed that there are some shortcomings in CEUS-CT/MR fusion imaging assessment. First, surgical procedures, changes in posture and the application of hydrodissection (artificial ascites/pleural effusion) could cause deformation of the liver and lead to failure in co-registration. This accounted for the majority of cases that failed in CEUS-CT/MR fusion imaging assessment. In such situations, 3DUS-CEUS fusion imaging could be helpful as data of index tumors could be acquired just before needle insertion and the influence of the liver deformation could be avoided [Citation42,Citation43]. Development of a new non-rigid registration technique might also be helpful. Second, location of the tumor could affect the accuracy of co-registration. In our study, one lesion in the fusion imaging group was diagnosed to be completely ablated with adequate AM according to the intraprocedural CEUS-CT/MR fusion imaging assessment. However, it turned out to be incompletely ablated 1 month after the procedure. Note that this lesion was located just beneath the diaphragm where few intrahepatic landmarks were available for registration. Third, the magnetic field used for positioning was unstable. The interference might be from the relatively long distance between the magnetic generator and sensor, the iron bed, or other magnetic fields. Thus, improvement of the fusion imaging system is needed.
Also, there were some limitations in our study. First, it was a single center, non-randomized study. The sample size was relatively small but this was because of the low incidence of LTP. Second, the HCCs included in our study were relatively small. As previously discussed, the advantage of CEUS-CT/MR fusion imaging assessment might be more significant for difficult ablation tumors. Thus, additional studies on larger HCCs or HCCs that were close to major vessels are necessary. Third, as CEUS-CT/MR fusion imaging was less experience-dependent compared with routine CEUS in treatment response assessment, its efficiency when performed by inexperienced young operators remains unknown and needs further study.
Conclusion
In conclusion, although intraprocedural CEUS-CT/MR fusion imaging has certain limitations in application, it might provide a potential more efficient method compared with routine CEUS in reducing LTP in HCC thermal ablation, especially for difficult ablation lesions.
Disclosure statement
The authors report no conflicts of interest.
Additional information
Funding
References
- Bruix J, Sherman M. Management of hepatocellular carcinoma: an update. Hepatology. 2011;53:1020–1022.
- Weinstein JL, Ahmed M. Percutaneous ablation for hepatocellular carcinoma. Am J Roentgenol. 2018;210:1368–1375.
- Ng KKC, Chok KSH, Chan ACY, et al. Randomized clinical trial of hepatic resection versus radiofrequency ablation for early-stage hepatocellular carcinoma. Br J Surg. 2017;104:1775–1784.
- Dill-Macky MJ, Asch M, Burns P, et al. Radiofrequency ablation of hepatocellular carcinoma: predicting success using contrast-enhanced sonography. Am J Roentgenol. 2006;186:S287–S295.
- Lu MD, Yu XL, Li AH, et al. Comparison of contrast enhanced ultrasound and contrast enhanced CT or MRI in monitoring percutaneous thermal ablation procedure in patients with hepatocellular carcinoma: a multi-center study in China. Ultrasound Med Biol. 2007;33:1736–1749.
- Meloni MF, Andreano A, Franza E, et al. Contrast enhanced ultrasound: should it play a role in immediate evaluation of liver tumors following thermal ablation? Eur J Radiol. 2012;81:e897–902.
- Morimoto M, Nozawa A, Numata K, et al. Evaluation using contrast-enhanced harmonic gray scale sonography after radiofrequency ablation of small hepatocellular carcinoma: sonographic-histopathologic correlation. J Ultrasound Med. 2005;24:273–283.
- Ricci P, Cantisani V, Drudi F, et al. Is contrast-enhanced US alternative to spiral CT in the assessment of treatment outcome of radiofrequency ablation in hepatocellular carcinoma? Ultraschall Med. 2009;30:252–258.
- Vilana R, Bianchi L, Varela M, et al. Is microbubble-enhanced ultrasonography sufficient for assessment of response to percutaneous treatment in patients with early hepatocellular carcinoma? Eur Radiol. 2006;16:2454–2462.
- Minami Y, Nishida N, Kudo M. Therapeutic response assessment of RFA for HCC: contrast-enhanced US, CT and MRI. World J Gastroenterol. 2014;20:4160.
- Choi D, Lim HK, Rhim H, et al. Percutaneous radiofrequency ablation for early-stage hepatocellular carcinoma as a first-line treatment: long-term results and prognostic factors in a large single-institution series. Eur Radiol. 2007;17:684–692.
- Lencioni R, Cioni D, Crocetti L, et al. Early-stage hepatocellular carcinoma in patients with cirrhosis: long-term results of percutaneous image-guided radiofrequency ablation. Radiology. 2005;234:961–967.
- Shiina S, Tateishi R, Arano T, et al. Radiofrequency ablation for hepatocellular carcinoma: 10-year outcome and prognostic factors. Am J Gastroenterol. 2012;107:569–577.
- Hao Y, Numata K, Ishii T, et al. Rate of local tumor progression following radiofrequency ablation of pathologically early hepatocellular carcinoma. World J Gastroenterol. 2017;23:3111–3121.
- Kei SK, Rhim H, Choi D, et al. Local tumor progression after radiofrequency ablation of liver tumors: analysis of morphologic pattern and site of recurrence. Am J Roentgenol. 2008;190:1544–1551.
- Kim YS, Lee WJ, Rhim H, et al. The minimal ablative margin of radiofrequency ablation of hepatocellular carcinoma (>2 and <5 cm) needed to prevent local tumor progression: 3D quantitative assessment using CT image fusion. Am J Roentgenol. 2010;195:758–765.
- Koda M, Tokunaga S, Miyoshi K, et al. Ablative margin states by magnetic resonance imaging with ferucarbotran in radiofrequency ablation for hepatocellular carcinoma can predict local tumor progression. J Gastroenterol. 2013;48:1283–1292.
- Liu CH, Arellano RS, Uppot RN, et al. Radiofrequency ablation of hepatic tumours: effect of post-ablation margin on local tumour progression. Eur Radiol. 2010;20:877–885.
- Nakazawa T, Kokubu S, Shibuya A, et al. Radiofrequency ablation of hepatocellular carcinoma: correlation between local tumor progression after ablation and ablative margin. Am J Roentgenol. 2007;188:480–488.
- Makino Y, Imai Y, Igura T, et al. Usefulness of the multimodality fusion imaging for the diagnosis and treatment of hepatocellular carcinoma. Dig Dis. 2012;30:580–587.
- Minami Y, Kudo M. Ultrasound fusion imaging of hepatocellular carcinoma: a review of current evidence. Dig Dis. 2014;32:690–695.
- Toshikuni N, Tsutsumi M, Takuma Y, et al. Real-time image fusion for successful percutaneous radiofrequency ablation of hepatocellular carcinoma. J Ultrasound Med. 2014;33:2005–2010.
- Hirooka M, Iuchi H, Kumagi T, et al. Virtual sonographic radiofrequency ablation of hepatocellular carcinoma visualized on CT but not on conventional sonography. Am J Roentgenol. 2006;186:S255–S260.
- Mauri G, Cova L, De Beni S, et al. Real-time US-CT/MRI image fusion for guidance of thermal ablation of liver tumors undetectable with US: results in 295 cases. Cardiovasc Intervent Radiol. 2015;38:143–151.
- Minami Y, Kudo M, Chung H, et al. Percutaneous radiofrequency ablation of sonographically unidentifiable liver tumors. Feasibility and usefulness of a novel guiding technique with an integrated system of computed tomography and sonographic images. Oncology. 2007;72:111–116.
- Li K, Su Z, Xu E, et al. Evaluation of the ablation margin of hepatocellular carcinoma using CEUS-CT/MR image fusion in a phantom model and in patients. BMC Cancer. 2017;17:61.
- Li K, Su ZZ, Xu EJ, et al. Improvement of ablative margins by the intraoperative use of CEUS-CT/MR image fusion in hepatocellular carcinoma. BMC Cancer. 2016;16:277.
- Zhong-Zhen S, Kai L, Rong-Qin Z, et al. A feasibility study for determining ablative margin with 3D-CEUS-CT/MR image fusion after radiofrequency ablation of hepatocellular carcinoma. Ultraschall Med. 2012;33:E250–E255.
- Bo XW, Xu HX, Guo LH, et al. Ablative safety margin depicted by fusion imaging with post-treatment contrast-enhanced ultrasound and pre-treatment CECT/CEMRI after radiofrequency ablation for liver cancers. Br J Radiol. 2017;90:20170063.
- Makino Y, Imai Y, Igura T, et al. Feasibility of extracted-overlay fusion imaging for intraoperative treatment evaluation of radiofrequency ablation for hepatocellular carcinoma. Liver Cancer. 2016;5:269–279.
- Numata K, Fukuda H, Morimoto M, et al. Use of fusion imaging combining contrast-enhanced ultrasonography with a perflubutane-based contrast agent and contrast-enhanced computed tomography for the evaluation of percutaneous radiofrequency ablation of hypervascular hepatocellular carcinoma. Eur J Radiol. 2012;81:2746–2753.
- Claudon M, Dietrich CF, Choi BI, et al. Guidelines and good clinical practice recommendations for Contrast Enhanced Ultrasound (CEUS) in the liver – update 2012: a WFUMB-EFSUMB initiative in cooperation with representatives of AFSUMB, AIUM, ASUM, FLAUS and ICUS. Ultrasound Med Biol. 2013;39:187–210.
- Makino Y, Imai Y, Igura T, et al. Utility of computed tomography fusion imaging for the evaluation of the ablative margin of radiofrequency ablation for hepatocellular carcinoma and the correlation to local tumor progression. Hepatol Res. 2013;43:950–958.
- Okusaka T, Okada S, Ueno H, et al. Satellite lesions in patients with small hepatocellular carcinoma with reference to clinicopathologic features. Cancer. 2002;95:1931–1937.
- Goldberg SN, Grassi CJ, Cardella JF, et al. Image-guided tumor ablation: standardization of terminology and reporting criteria. Radiology. 2005;235:728–739.
- Komatsu S, Murakami M, Fukumoto T, et al. Risk factors for survival and local recurrence after particle radiotherapy for single small hepatocellular carcinoma. Br J Surg. 2011;98:558–564.
- Kono M, Inoue T, Kudo M, et al. Radiofrequency ablation for hepatocellular carcinoma measuring 2 cm or smaller: results and risk factors for local recurrence. Dig Dis. 2014;32:670–677.
- Tateishi R, Shiina S, Teratani T, et al. Percutaneous radiofrequency ablation for hepatocellular carcinoma. An analysis of 1000 cases. Cancer. 2005;103:1201–1209.
- Zytoon AA, Ishii H, Murakami K, et al. Recurrence-free survival after radiofrequency ablation of hepatocellular carcinoma. A registry report of the impact of risk factors on outcome. Jpn J Clin Oncol. 2007;37:658–672.
- Sala M, Llovet JM, Vilana R, et al. Initial response to percutaneous ablation predicts survival in patients with hepatocellular carcinoma. Hepatology. 2004;40:1352–1360.
- Takahashi S, Kudo M, Chung H, et al. Initial treatment response is essential to improve survival in patients with hepatocellular carcinoma who underwent curative radiofrequency ablation therapy. Oncology. 2007;72:98–103.
- Xu EJ, Lv SM, Li K, et al. Immediate evaluation and guidance of liver cancer thermal ablation by three-dimensional ultrasound/contrast-enhanced ultrasound fusion imaging. Int J Hyperthermia. 2018;34:870–876.
- Toshikuni N, Shiroeda H, Ozaki K, et al. Advanced ultrasonography technologies to assess the effects of radiofrequency ablation on hepatocellular carcinoma. Radiol Oncol. 2013;47:224–229.