Abstract
Despite the significant improvement of knowledge and technologies in tumor treatments, pancreatic tumor remains a complex disease still characterized by a poor prognosis. The increasing role of minimally invasive techniques started to drive the effort of scientific medicine to evaluate the possibilities of application of these techniques to pancreatic cancer. The purpose of this paper is to present a brief summary of the different ablative techniques available and proposed for pancreatic tumor treatment considering invasive, noninvasive, thermal and non-thermal techniques.
Introduction
Pancreatic tumor remains a challenging issue in the medical field. Despite the improvements that are occurring in last years in all clinical fields, pancreatic cancer is still burdened by poor prognosis and the gold standard for treatment when possible is surgery [Citation1]. Unfortunately, less than 20% of patients is eligible for pancreatectomy at the diagnosis; the remaining patients are treated with systemic therapy that leads anyway to a 5-years survival less than 5% [Citation2]. These assumptions drove research efforts to find other approaches to support systemic therapy such as radiotherapy [Citation3] or other minimally invasive procedures [Citation4].
Image guided ablations are gaining increasing attention in the current clinical practice providing reduced invasiveness, good outcomes and low morbidity in the treatment of several different tumors (e.g., liver, kidney, lung) [Citation5–10]. Particularly, improvements in imaging-guidance methods and ablative technologies are contributing to the large diffusion of ablative techniques, and for expanding their possible applications in more and more difficult and complex scenarios [Citation11–14]. Dealing with pancreatic cancer, the main indication for locoregional treatments is pain palliation in advanced stages.
The purpose of this paper is to present the different ablative techniques available and proposed for pancreatic tumor treatment that can be divided into:
invasive – thermal techniques;
invasive – non-thermal techniques;
noninvasive thermal techniques.
Invasive – thermal techniques
These techniques are mostly represented by radiofrequency ablation and microwave ablation (RFA and MWA), but other techniques as laser ablation and cryoablation were developed in last years. They are considered minimally invasive techniques because of the requirement of needle positioning in the tumor, that can be performed percutaneously or during laparoscopic o laparotomic surgery.
RFA
Radiofrequency ablation induces coagulative necrosis within the tumor mass through the production of high temperatures, induced by the application of high-frequency alternating current. RFA represents the oldest thermal technique available and several papers were published on its application on different tumors and organs [Citation15–17]. The current technology allows ablation areas up to 5 cm of diameter requiring long ablation time, the application of a large area contact plate on the patient's skin (which could cause skin burns).
The temperature reached during the ablation seems to correlate with complication, for this reason some authors proposed to avoid more than 90 °C temperatures [Citation18–20]. While endoscopic ultrasound (EUS) is safer for lesions in the pancreatic head, the percutaneous approach can be adopted for lesions located in the body of the pancreas [Citation20].
Few papers deal with percutaneous approach, ultrasound (US) or computed tomography (CT) guided [Citation21,Citation22], while the larger amount of data available are on laparotomic surgery [Citation23] and no direct comparative study were performed. About 20% of patient presents a post-operative complication related to the RFA, the more frequent ones being pancreatic fistula, portal thrombosis and pancreatitis [Citation4]. Even data on mortality are widely different, Wu et al. report a mortality rate of 25% mostly due to massive post-operative hemorrhage [Citation24].
Data on follow-up are not constantly reported and have a wide range; OS ranges from 0% to 100% with observation lasting from 10 to 34 months [Citation21–23].
Survival benefits are suggested from a comparative study between patients receiving chemotherapy alone vs. chemotherapy plus RFA with mean overall survival rising to 20 months for combined approach from 13 months of the chemotherapy alone group [Citation25].
MWA
MWA is a relative newer technique compared to RFA and data on clinical application on pancreatic tumors are even less [Citation26,Citation27]. The MW unit consists of a microwave generator and a cooled shaft antenna. Erogated energy could be pulsed or continuous at 2450 MHz, at this frequency waves are mainly absorbed by water, causing heating over 100 °C.
Data available on pancreas tumors are presented by case reports or series reporting the experience with up to 20 cases [Citation28–30].
The main objective of these studies was to evaluate the feasibility and safety of percutaneous MWA. Complication rates reported are extremely low, with one major complication reported (one month delayed pseudoaneurysm of the gastroduodenal artery) and only few minor ones, such as pain, delayed pancreatitis (one month after the procedure) and pseudocyst. Even data on follow-up are poor but overall survival rate reported at 1 year reaches 80%. It has already been demonstrated that MWA offers several technical advantages over RFA in liver ablation, such as higher ablation volumes, shortened treatment duration and lower susceptibility to the heat-sink effect and we can suppose that these advantages will be reported even on pancreatic MWA.
Laser
Laser is coherent light beam that heats tissues interacting with them. Light penetrates the tissue and is absorbed and scattered in the tissue converting the energy in heat. The heating of the tissue by means of light depends on the absorption capability of the tissue itself [Citation31]. Due to the very small caliber of the applicators, laser has been used in the ablation of tumors in critical locations [Citation32–36].
Laser ablation technique provides the insertion of one or more needles in the lesion in order to insert an optical fiber which will deliver laser light. Needle positioning could be performed percutaneously or during endoscopy (EUS guided), anyhow few cases or series reporting endoscopic procedures are available in the literature [Citation4,Citation37,Citation38].
The largest series presents the data of nine patients with lesion size ranging from 21 mm to 45 mm. The patients were divided into three groups and treated with a total delivered energy of 800, 1000 and 1200 J, at a low-power setting (2, 3 and 4 W), respectively. All the procedures were performed under EUS guidance to avoid adjacent structures as vessels and to detect intraprocedural complication (none was reported). EUS was also used for real-time procedure monitoring. Additionally, in all cases, a contrast enhanced ultrasonography was performed before and after LA. In all cases, the EUS revealed a hyperechoic area. Regarding the clinical short-term outcomes, no major events were recorded. Three patients reported a post-procedural fluid collection, while a threefold increase in the amylase serum level was evidenced in other two patients. All of them were successfully treated conservatively. Follow up was performed at 24 h, 7 and 30 days after the ablation by means of CT scans. In all the follow-up periods, a clear margin ablation was evidenced with a gradual involution of the ablated areas. One patient died due to a not procedure related myocardial infarction before the 30-day CT scan reevaluation (mortality rate of 11%). Median OS was 7.4 months (range 29–662 days).
Cryoablation
Cryoablation freezes tissues to temperatures lower than −20 °C and determine cell membranes destruction due to intracellular and extracellular ice formation. Also, indirect cellular damage results from disruption of the vascular endothelium. This technique has been successfully applied in the treatment of several different kind of tumors [Citation8,Citation10,Citation14,Citation39]. To date, cryoablation has not been widely used in the treatment of pancreatic tumors primarily due to the small volume of the pancreatic gland, fragile pancreatic parenchyma and its proximity to structures such as the stomach, duodenum, colon, common bile duct and vessels. Few reports are available dealing with pancreatic cryoablation feasibility [Citation40,Citation41]. Complication rates were significant with about 10% of major complication and up to more than 50% of minor complication. A study involving 49 patients with locally advanced pancreatic cancer who received intraoperative cryosurgery or percutaneous cryosurgery in a single institution hospital between March 2001 and November 2007 showed that median survival was 16.2 months and overall survival rates at 6, 12, 24 and 36 months were 94.9%, 63.1%, 22.8% and 9.5%, respectively [Citation41].
An interesting application of cryoablation could be the combination with immune therapy; cryotherapy not only promotes tumor cell necrosis but also enhances the antitumor immune response. In 2013, Niu et al. [Citation42] retrospectively assessed the effect of immunotherapy combined to cryo vs. chemotherapy showing that the median overall survival times in the cryoimmunotherapy and cryotherapy groups (13 and 7 months, respectively) were significant longer than those in the chemotherapy group (3.5 months).
Invasive non-thermal techniques
Irreversible electroporation
Irreversible electroporation (IRE) is the newest and most promising invasive technique for pancreatic cancer ablation. IRE is based on the application of short high-voltage electric pulses, in order to produce multiple micropores on cell membranes causing an irreversible permeabilization, which leads to disruption of cellular homeostasis, activating apoptotic pathways in tumor cells [Citation43]. The main advantage of IRE compared with other ablative techniques is the ability to preserve the extracellular matrix, thus allowing ablation adjacent to critical structures as nerves, vessels and biliary ducts; IRE is therefore the safest ablative approach for tumors encasing major peripancreatic vessels [Citation43,Citation44].
A typical IRE procedure for a solid tumor, with a size of approximately 3 cm in diameter, uses 90 pulses with an ultra-short pulse length of 100 μs; for bigger lesions, more application can be overlapped to reach the desired volume with well-demarcated margins that are usually clearly identified by imaging. This relatively short ablation time for IRE may correlate with reduced anesthesia time, reduced post-ablation pain, decreased ablation-related complications, decreased overall cost of ablation treatment.
Irreversible electroporation has been proposed for palliation of unresectable tumors of the pancreas, as a bridge therapy before surgery, and also as a technique for intraoperative ‘margin augmentation’, in order to reach R0 resection in technically unresectable pancreatic tumors [Citation45]. Open, laparoscopic and percutaneous approaches have been evaluated for IRE. In most cases, percutaneous IRE was performed under CT guidance, with encouraging results in terms of feasibility, safety and effectiveness [Citation46,Citation47]. Preliminary studies [Citation48,Citation49] reported successful percutaneous US-guided IRE of pancreatic cancer, without significant procedure-related complications. Månsson et al. [Citation50] reported a median survival of 7 months after percutaneous US guided IRE of pancreatic cancer; the median time from IRE was 6.1 months to local progression and 2.7 months to observation of metastases. With larger studies, data on safety and overall survival after percutaneous US-guided IRE could be obtained to confirm its long-term efficacy within a multidisciplinary approach to unresectable pancreatic cancer.
Non-invasive non thermal techniques
High intensity focalized ultrasound
Ultrasound is a form of mechanical energy, represented by a wave of compression and rarefaction alternating each other, that propagates through a liquid or solid medium (e.g., tissues) yielding energy during its path. The difference between diagnostic US and HIFU is the amplitude and transmission of ultrasound waves. In HIFU, the US transducer is spherically curved, allowing ultrasound waves to be focused at the center of curvature. This can result in amplification of the pressure amplitude by a factor of 100 at the focus. The lesion targeting and monitoring during HIFU treatment, could be US-guided (USgHIFU) or MR-guided (MRgHIFU). The mechanism of tissue damage with high-energy ultrasound is mediated both by a thermal and a mechanical effect (). Thermal damage results from a temperature increase between 55 °C and 85 °C 12 in the treated volume, which causes cell coagulative necrosis. The mechanical effects produced by HIFU are cavitation, microstreaming and radiation force [Citation51–54].
Figure 1. Case of a patient with symptomatic pancreatic adenocarcinoma treated with high intensity focused ultrasound. (a) Magnetic resonance Imaging demonstrating pathologic pancreatic mass. (b) PET/CT scan demonstrating high FDG uptake of the pancreatic tumor. (c) Treatment with US-guided high-intensity focused ultrasound. (d) PET/CT scan after treatment demonstrating lack of FDG uptake in the ablated area.
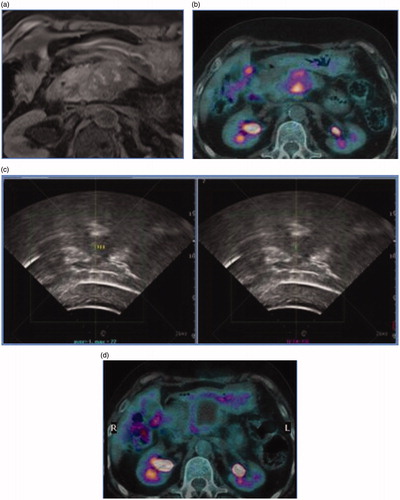
There are few preliminary studies reporting the results of HIFU impact on pancreatic cancer patients survival rates [Citation55–58]. The median overall survival and median time to progression, range from 6 to 11 months and from 5 to 8.4 months, respectively [Citation57,Citation58]. One-year survival rates after HIFU can reach 30.8–42% [Citation59,Citation60] and seems can prolong patients survival (median survival of 13 months) [Citation61].
Side effects reported after HIFU are mild to severe abdominal pain (in 5.9% of cases), edema (4.1%) and skin burns (1.7%) [Citation60,Citation62]. The most dangerous complication is bowel perforation, that can occur when the intestinal loops are near to the ultrasound beam path and the targeted area.
HIFU procedure, as a pain-relief technique, is completely noninvasive, since there is no transcutaneous approach or needle insertion, differently from other minimally invasive interventional procedures.
HIFU has been shown to be effective in the treatment of pancreatic cancer-related pain, with a range of 75–100% of patients experiencing significant pain relief [Citation54,Citation55,Citation60,Citation63]. The majority of treated patients refer an immediate and significant antalgic effect that can last for months; the long-term palliative effect is difficult to be evaluated since life expectancy of patients with advanced stage disease is poor.
The main limitations of HIFU, are mainly related to the imaging guidance: acoustic shadowing, reverberation, refraction and other acoustic artifacts are specific for the US imaging. However, HIFU itself is affected by the same acoustic artifacts, which may affect both the efficacy and the safety of the treatment. In order to decrease acoustic shadowing artifacts, an adequate bowel preparation for reducing meteorism, is usually required together with a specific slag-free diet. By applying a slight to moderate pressure on the abdominal surface, above the targeted area, bowel loops may also be compressed or displaced, in order to achieve a better and clearer acoustic window, for energy transmission to the target. Very high procedural costs and the treatment length are the main weaknesses for the HIFU, along with the complexity in safely tracking the pancreatic target due to the respiratory artifacts, but technological research and development could lead to cheaper and faster instruments.
Conclusions
Limited data are available on minimally invasive techniques in pancreatic primary tumor ablation. The reported literature highlights the necessity for larger studies analyzing not only the single technique efficacy but also comparing the different techniques available. None of the techniques reported showed significant better results compared to the other ones (). Current data suggest the application of minimally invasive ablation techniques only in advanced pancreatic tumor setting with palliative intent. The decision of which ablating technique apply should be decided based on center expertise.
Table 1. Pros and cons of different image-guided ablative modalities for the treatment of pancreatic cancer.
Disclosure statement
No potential conflict of interest was reported by the authors.
References
- Varadhachary GR, Tamm EP, Abbruzzese JL, et al. Borderline resectable pancreatic cancer: definitions, management, and role of preoperative therapy. Ann Surg Oncol. 2006;13:1035–1046.
- Sheahan AV, Biankin AV, Parish CR, et al. Targeted therapies in the management of locally advanced and metastatic pancreatic cancer: a systematic review. Oncotarget. 2018;21613:21627.
- Hammel P, Huguet F, Van Laethem JL, et al. Effect of chemoradiotherapy vs chemotherapy on survival in patients with locally advanced pancreatic cancer controlled after 4 months of gemcitabine with or without erlotinib the LAP07 randomized clinical trial. JAMA. 2016;315:1844–1853.
- Saccomandi P, Lapergola A, Longo F, et al. Thermal ablation of pancreatic cancer: a systematic literature review of clinical practice and pre-clinical studies. Int J Hyperthermia. 2018;35:398–418.
- Dai Y, Covarrubias D, Uppot R, et al. Image-guided percutaneous radiofrequency ablation of central renal cell carcinoma: assessment of clinical efficacy and safety in 31 tumors. J Vasc Interv Radiol. 2017. 28:1643–1650.
- Mauri G, Gennaro N, De Beni S, et al. Real-time US-18FDG-PET/CT image fusion for guidance of thermal ablation of 18FDG-PET-positive liver metastases: the added value of contrast enhancement. Cardiovasc Intervent Radiol. 2019;42:60–68.
- Zampino MG, Magni E, Ravenda PS, et al. Treatments for colorectal liver metastases: a new focus on a familiar concept. Crit Rev Oncol Hematol. 2016;108:154–163.
- Shyn PB, Mauri G, Alencar RO, et al. Percutaneous imaging-guided cryoablation of liver tumors: predicting local progression on 24-hour MRI. Am J Roentgenol. 2014;203:W181–W191.
- Mauri G, Nicosia L, Varano GM, et al. Tips and tricks for a safe and effective image-guided percutaneous renal tumour ablation. Insights Imaging. 2017;8:357–363.
- Mauri G, Sconfienza LM, Pescatori LC, et al. Technical success, technique efficacy and complications of minimally-invasive imaging-guided percutaneous ablation procedures of breast cancer: a systematic review and meta-analysis. Eur Radiol. 2017;27:3199.
- Mauri G, Beni S, De Forzoni L, et al. Virtual navigator automatic registration technology in abdominal application. Annual International Conference of the IEEE Engineering in Medicine and Biology Society; 2014 Aug 26–30; Chicago, Illinois: IEEE; 2014.
- Mauri G. Expanding role of virtual navigation and fusion imaging in percutaneous biopsies and ablation. Abdom Imaging. 2015;40:3238–3239.
- Mauri G, Cova L, De Beni S, et al. Real-time US-CT/MRI image fusion for guidance of thermal ablation of liver tumors undetectable with US: results in 295 cases. Cardiovasc Intervent Radiol. 2015;38:143–151.
- Mauri G, Nicosia L, Verano GM, et al. Unusual tumour ablations: report of difficult and interesting cases. Ecancermedicalscience. 2017;11:733.
- Mauri G, Porazzi E, Cova L, et al. Intraprocedural contrast-enhanced ultrasound (CEUS) in liver percutaneous radiofrequency ablation: clinical impact and health technology assessment. Insights Imaging. 2014;5:209–216.
- Solbiati L, Ahmed M, Cova L, et al. Small liver colorectal metastases treated with percutaneous radiofrequency ablation: local response rate and long-term survival with up to 10-year follow-up. Radiology. 2012;265:958–968.
- Papini E, Pacella CM, Solbiati LA, et al. Minimally-invasive treatments for benign thyroid nodules: a Delphi-based consensus statement from the Italian minimally-invasive treatments of the thyroid (MITT) group. Int J Hyperth. 2019;36:376–382.
- Girelli R, Frigerio I, Giardino A, et al. Results of 100 pancreatic radiofrequency ablations in the context of a multimodal strategy for stage III ductal adenocarcinoma. Langenbeck’s Arch Surg. 2013;398:63–69.
- D’Onofrio M, Barbi E, Girelli R, et al. Radiofrequency ablation of locally advanced pancreatic adenocarcinoma: an overview. World J Gastroenterol. 2010;16:3478–3483.
- D’Onofrio M, Ciaravino V, De Robertis R, et al. Percutaneous ablation of pancreatic cancer. World J Gastroenterol. 2016;22:9661.
- D’Onofrio M, Crosara S, De Robertis R, et al. Percutaneous radiofrequency ablation of unresectable locally advanced pancreatic cancer: preliminary results. Technol Cancer Res Treat. 2017;16:285–294.
- Rossi S, Viera FT, Ghittoni G, et al. Radiofrequency ablation of pancreatic neuroendocrine tumors: a pilot study of feasibility, efficacy, and safety. Pancreas. 2014;43:938.
- Giardino A, Girelli R, Frigerio I, et al. Triple approach strategy for patients with locally advanced pancreatic carcinoma. HPB. 2013;15:623–627.
- Wu Y, Tang Z, Fang H, et al. High operative risk of cool-tip radiofrequency ablation for unresectable pancreatic head cancer 1. J Surg Oncol. 2006;94:392–395.
- Spiliotis JD, Datsis AC, Michalopoulos NV, et al. Radiofrequency ablation combined with palliative surgery may prolong survival of patients with advanced cancer of the pancreas. Langenbeck’s Arch Surg. 2007;392:55–60.
- D’Amico F, Finotti M, Di Renzo C, et al. Microwave thermal ablation in an unusual case of malignant and locally advanced rare tumor of pancreas in ASA IV old male patient and literature review. Case Rep Gastrointest Med. 2018;2018:1–7.
- Lygidakis NJ, Sharma SK, Papastratis P, et al. Microwave ablation in locally advanced pancreatic carcinoma – a new look. Hepatogastroenterology. 2007;54:1305–1310.
- Vogl TJ, Panahi B, Albrecht MH, et al. Microwave ablation of pancreatic tumors. Minim Invasive Ther Allied Technol. 2018;27:33–40.
- Carrafiello G, Ierardi AM, Fontana F, et al. Microwave ablation of pancreatic head cancer: safety and efficacy. J Vasc Interv Radiol. 2013;24:1513–1520.
- Ierardi AM, Biondetti P, Coppola A, et al. Percutaneous microwave thermosphere ablation of pancreatic tumours. Gland Surg. 2018;7:59.
- Welch AJ, Van Gemert MJC. Optical-thermal response of laser-irradiated tissue. Berlin: Springer; 2011.
- Mainini AP, Monaco C, Pescatori LC, et al. Image-guided thermal ablation of benign thyroid nodules. J Ultrasound. 2017;20:11–22.
- Mauri G, Cova L, Ierace T, et al. Treatment of metastatic lymph nodes in the neck from papillary thyroid carcinoma with percutaneous laser ablation. Cardiovasc Intervent Radiol. 2016;39:1023–1030.
- Mauri G, Nicosia L, Della Vigna P, et al. Percutaneous laser ablation for benign and malignant thyroid diseases. Ultrasonography. 2019;38:25–36.
- Patelli G, Ranieri A, Paganelli A, et al. Transperineal laser ablation for percutaneous treatment of benign prostatic hyperplasia: a feasibility study. Cardiovasc Intervent Radiol. 2017;40:1440.
- Sartori S, Mauri G, Tombesi P, et al. Ultrasound-guided percutaneous laser ablation is safe and effective in the treatment of small renal tumors in patients at increased bleeding risk. Int J Hyperth. 2018;1:7.
- Di Matteo FM, Saccomandi P, Martino M, et al. Feasibility of EUS-guided Nd:YAG laser ablation of unresectable pancreatic adenocarcinoma. Gastrointest Endosc. 2018;88:168–174.e1.
- Yoon WJ, Seo DW. Endoscopic ultrasound-guided local therapy of pancreatic tumors. Korean J Gastroenterol. 2015;66:154.
- Cazzato RL, Garnon J, Ramamurthy N, et al. Percutaneous image-guided cryoablation: current applications and results in the oncologic field. Med Oncol. 2016;33:1–16.
- Xu K-C, Niu L-Z, Hu Y-Z, et al. A pilot study on combination of cryosurgery and 125iodine seed implantation for treatment of locally advanced pancreatic cancer. World J Gastroenterol. 2008;14:1603–1611.
- Niu LZ, Li HB, Wen WF, et al. Feasibility and safety of percutaneous cryoablation for locally advanced pancreatic cancer. Zhonghua Yixianbing Zazhi. 2011;11:1–4.
- Niu L, Chen J, He L, et al. Combination treatment with comprehensive cryoablation and immunotherapy in metastatic pancreatic cancer. Pancreas. 2013;42:1143.
- Paiella S, Salvia R, Girelli R, et al. Role of local ablative techniques (radiofrequency ablation and irreversible electroporation) in the treatment of pancreatic cancer. Updates Surg. 2016;68:307.
- Venkat S, Hosein PJ, Narayanan G. Percutaneous approach to irreversible electroporation of the pancreas: Miami Protocol. Tech Vasc Interv Radiol. 2015;18:153–158.
- Tasu JP, Vesselle G, Herpe G, et al. Irreversible electroporation for locally advanced pancreatic cancer: where do we stand in 2017? Pancreas. 2017;46:283.
- Leen E, Picard J, Stebbing J, et al. Percutaneous irreversible electroporation with systemic treatment for locally advanced pancreatic adenocarcinoma. J Gastrointest Oncol. 2018;9:275–281.
- Narayanan G, Hosein PJ, Beulaygue IC, et al. Percutaneous image-guided irreversible electroporation for the treatment of unresectable, locally advanced pancreatic adenocarcinoma. J Vasc Interv Radiol. 2017;28:342–348.
- Månsson C, Bergenfeldt M, Brahmstaedt R, et al. Safety and preliminary efficacy of ultrasound-guided percutaneous irreversible electroporation for treatment of localized pancreatic cancer. Anticancer Res. 2014;34:289–293.
- Zhang Y, Shi J, Zeng J, et al. Percutaneous irreversible electroporation for ablation of locally advanced pancreatic cancer experience from a Chinese institution. Pancreas. 2017;46:e12.
- Månsson C, Brahmstaedt R, Nilsson A, et al. Percutaneous irreversible electroporation for treatment of locally advanced pancreatic cancer following chemotherapy or radiochemotherapy. Eur J Surg Oncol. 2016;42:1401–1406.
- Luo J, Ren X, Yu T. Efficacy of extracorporeal ultrasound-guided high intensity focused ultrasound: an evaluation based on controlled trials in China. Int J Radiat Biol. 2015;91:480–485.
- Orsi F, Mauri G. HIFU and radio frequency as alternatives to surgery In: Veronesi U, Goldhrisch A, editors. Breast cancer: innovations in research and management. Switzerland: Springer International Publishing; 2017. p. 849–854.
- Mauri G, Nicosia L, Xu Z, et al. Focused ultrasound: tumour ablation and its potential to enhance immunological therapy to cancer. BJR. 2018;91:20170641.
- Zhou Y-F. High intensity focused ultrasound in clinical tumor ablation. World J Clin Oncol. 2010;2:8–27.
- Orgera G, Monfardini L, Della Vigna P, et al. High-intensity focused ultrasound (HIFU) in patients with solid malignancies: evaluation of feasibility, local tumour response and clinical results. Radiol Med. 2011;116:734–748.
- Orsi F, Orgera G. Does it have a role in breast cancer? Cardiovasc Intervent Radiol. 2010. https://doi.org/10.1007/s00270-010-9954-3
- Wang K, Chen Z, Meng Z, et al. Analgesic effect of high intensity focused ultrasound therapy for unresectable pancreatic cancer. Int J Hyperth. 2011;27:101–107.
- Gao HF, Wang K, Meng ZQ, et al. High intensity focused ultrasound treatment for patients with local advanced pancreatic cancer. Hepatogastroenterology. 2013;60. https://doi.org/10.5754/hge13498
- Melodelima D, Chapelon JY, Theillère Y, et al. Combination of thermal and cavitation effects to generate deep lesions with an endocavitary applicator using a plane transducer: ex vivo studies. Ultrasound Med Biol. 2004;30:103–111.
- Dababou S, Marrocchio C, Scipione R, et al. High-intensity focused ultrasound for pain management in patients with cancer. RadioGraphics. 2018;38:603.
- Vidal-Jove J, Perich E, Del Castillo MA. Ultrasound guided high intensity focused ultrasound for malignant tumors: the Spanish experience of survival advantage in stage III and IV pancreatic cancer. Ultrason Sonochem. 2015;27:703–706.
- Marinova M, Rauch MM, Mücke M, et al. High-intensity focused ultrasound (HIFU) for pancreatic carcinoma: evaluation of feasibility, reduction of tumour volume and pain intensity. Eur Radiol. 2016;26:4047–4056.
- Wu F. High intensity focused ultrasound: a noninvasive therapy for locally advanced pancreatic cancer. World J. Gastroenterol. 2014;20:16480–16488.