Abstract
Purpose: To review the available options of percutaneous ablation of lung metastasis.
Methods: General indications, prognostic factors, and image guidance of percutaneous lung ablations were reviewed. Specificities, technical aspects, advantages and limitations of each technic were highlighted. Complications and follow up where also reviewed.
Results: Image-guided, percutaneous ablation is of interest for patients with a limit number (<3–5) small metastases (<2–3 cm). Other predictive factors have been reported such as the disease-free interval, the primary tumor, or the proximity with large vessels or bronchus. Radiofrequency ablation (RFA) is the most reported technic, with local control rate >90% for small tumors, and a very low complication rate. Microwave (MWA) and cryoablation are alternative technics developed in the last 15 years to overcome RFA limitations, with encouraging results. Larger ablations zones and less heat sink effect have been described with MWA. On the other hand, cryoablation allows painless treatments under conscious sedation and/or local anesthesia, high accessibility of difficult locations and promising results on prospective multicenter series. Although irreversible electroporation (IRE) could be used for lesions close to main blood vessels as it is not limited by the heat sink effect and does not have significant effects on connective tissue, allowing to treat lesions near to vital organs, preliminary results for lung metastasis are disappointing.
Conclusion: Percutaneous ablation of lung metastases, whatever technic is used, is feasible, with high local control rate, and acceptable complication rate. Although indications seem clear enough, validation through controlled trials is mandatory.
Introduction
Lung is one of the most common sites of metastatic spread [Citation1] with incidence varying from 20%, for patients with metastatic lesions [Citation2] limited to the lung, to 55% for patients with multi-organ involvement [Citation2,Citation3].
Nowadays, diagnosis is made easier and faster thank to better accuracy of modern imaging modalities, specifically CT scan and PET-CT ().
Figure 1. (A, B and C) Lung metastases presentation on CT-scan. Patient with three metachronous, bilateral metastases (arrows) from a rectal cancer. All metastases are sub-pleural, in the lung bases, with well-defined margins. In image B, there is a vessel going into the tumor, this is called ‘the Feeding-Vessel’ sign (arrowhead).
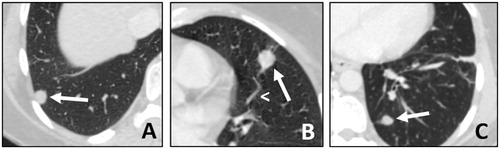
Lesions mainly responsible for lung metastasis are epithelial carcinomas (i.e. colorectal, kidney, ENT and breast cancers), accounting for about 75% of all lung metastases, followed by sarcomas (15%) melanomas (5%) and germ cell tumors (2–3%) [Citation4].
As the main pathways of dissemination are haematogenic and lymphatic, lung bases and subpleural space are the commonest metastatic areas, where the lymphovascular network is more developed [Citation5].
In selected cases of oligometastatic disease (i.e. with metastases confined to one or few sites), local treatments may prolong survival and more rarely used for symptom palliation [Citation6].
Local treatments include surgery, percutaneous ablations and stereotactic body radiation therapy [Citation7]. Thus, in order to choose the best option, cases have to be discussed in multidisciplinary tumor boards involving at least oncologist, organ specialist, radiologists, radiotherapist and surgeons.
Nowadays, there is no consensus regarding the maximum number of lesions that may be ablated, even though patients with <5 lesions, or even <3, are considered better candidates for ablation.
The present review will focus on the different percutaneous techniques to treat lung metastasis, considering indications, contraindications, outcome, follow-up and perspectives.
General indications and contraindications
Nowadays, the three main indications for local treatments are: curative intent, chemo-vacations and, more recently, to treat metastases that present a dissociated or disproportional response to chemotherapy or new generation treatments (i.e. targeted biotherapies, immunotherapies, antiangiogenics) to preserve further lines.
Moreover, prognosis is strongly related to the nature of the primary tumor [Citation8]. Thus, based on surgical and histological series, patients most likely to respond to local treatments are those with [Citation9]:
Long disease-free interval (>36 months) between the treatment of the primary tumor and the appearance of metastases.
Single or oligometastatic disease (i.e. <3–5 metastases).
Disease involving only the lung (or small number of extra-thoracic locations).
Patients in which local treatment are likely to achieve complete ablation/resection (‘R0’).
Small size (up to 2–3 cm of larger diameter).
Contraindications are those common to all interventional procedures, such as anticoagulation therapy or clopidogrel that cannot be withheld, platelets <50.000/µL, prothrombin activity <50% or INR >1.5 [Citation10,Citation11].
There are no established limits regarding functional respiratory parameters, and percutaneous ablation is feasible in single lung patients [Citation12] or patients having undergone previous surgical or percutaneous procedures. Nevertheless, areas already treated with radiation therapies seem more prone to complications and studies are ongoing on this precise topic [Citation13].
Finally, pacemaker- or defibrillator-carriers should not undergo radiofrequency, as the electric wave can interfere with the function of the device. So, microwave or cryoablation should be preferred in these cases.
Ablation techniques
Radiofrequency ablation (RFA)
It is the first percutaneous technique used in this setting [Citation14]. It consists in creating an electric field oscillating with a frequency ranging between 375 and 500 MHz, with the progressive generation of heat that is then dispersed through the tissue resulting in coagulative necrosis [Citation15].
The vast majority of electrodes in use for lung ablation operate in a monopolar mode, i.e. with a grounding pad placed on the skin surface allowing electric diffusion.
Length and characteristics of the needle allow modulating the energetic deposit as well as the size and shape of ablation, as an example deployable needles can increase the surface of energy deliverance producing spherical ablation zones.
Currently, generators allow for gradual rise of energy deliverance using well-defined protocols, to reduce the carbonization phenomena, appearing for a temperature over 100 °C.
Size of the ablation is determined by technical parameters including amount and duration of the stimulus, as well as the characteristics of the tissue, such as density and proximity to bronchovascular structures. In fact, vascular structures near a target lesion could limit the effectiveness of RFA and of all thermal-based ablation modalities due to the so called ‘heat sink effect’, causing thermal energy dissipation by flowing blood [Citation6].
Microwaves ablation (MWA)
MWA, like RFA, is a thermal ablation technique based on the use of electromagnetic energy (). It works with a frequency spectrum between 915 MHz and 2.45 GHz [Citation16], and allows to use multiple probes at the same time, with no need for a grounding pad because of a shorter radiofrequency wave length when compared to RFA [Citation17].
Figure 2. A. 67-y.o. patient with a single metastasis from rectal cancer, 28 mm in diameter, (black asterisk) treated with MWA (white arrow). B. A post treatment pneumothorax was found (white asterisk). C. It required thoracic drain insertion (black arrow). The drain was withdrawn at day 1 and the patient dismissed.
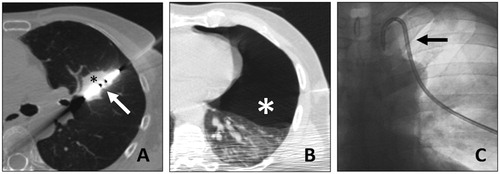
Its efficacy is not modified by the impedance of the surrounding tissue. Thus, the ablation can be carried out at higher temperatures (up to 180°), reducing the heat-sink effect and resulting in more extensive necrosis is a shorter time, even for lesions close to large vessels [Citation18–20].
For a successful ablation, the temperature should be homogeneously kept at 50 – 60 °C for at least 5 min [Citation16,Citation21], allowing ablations up to 5 cm in a single session [Citation22,Citation23].
Cryoablation (CA)
Cryotherapy kills tumor cells through a complex combination of different mechanisms produced by tissue freezing and thawing (). The principle is based on the Joule–Thomson effect. The ablation process consists of successive freezing-thawing cycles and induces cell death by protein denaturation, membrane disruption, and microvascular thrombosis [Citation24–27].
Figure 3. Cryoablation of a posterior right basal lung metastasis with four needles. A. Lung metastasis before treatment (black asterisk). B. Final positioning of the four needles (arrowheads) in the lung lesion (asterisk) in MIP (B(0).1) and VR (B(0).2) reconstructions. C. Lung lesion at the end of the treatment (asterisk) with minimal peripheral intra alveolar hemorrhage (white arrows). D. Control of cryoablation scares (asterisks) at 1 month (D(0).1), 3 months (D(0).2) and 6 months (D(0).3).
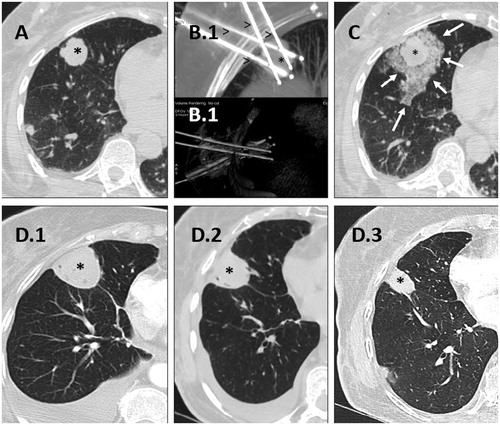
It is also hypothesized that the necrosis induced by freezing does not damage the cellular content that is consequently presented to the immune system, favoring a specific antitumoral response. This tumor-specific immune reaction may prime or enhance the efficacy of antitumoral immunotherapies [Citation28].
Moreover, cryoablation better preserves the collagenous architecture inside the ablated area which may be advantageous in treating lesions adjacent to the bronchi [Citation29].
Furthermore, as cryoablation is based on hypothermic damage, it is characterized by low incidence of per-procedural pain. Thus, it can be performed under conscious sedation and/or local anesthesia, even when the target lesion abuts the chest wall [Citation30]. As for MWA, several cryoprobes can be activated at the same time to obtain a larger ablation area.
As the predominant air component of the alveolar structure can interfere with the freezing process, the treatment protocol has been modified in order to increase the water component and to favorize the ice-ball formation.
A first short (3 min) freezing and thawing cycle is carried out, leading to fluid and hemorrhage filling of airspaces, hence, resulting in an estimated 20-fold increase of thermal conductivity [Citation31].
The process leads to the formation of a frozen area, called ‘ice ball’ which is slightly larger than the ablation zone (0 °C isotherm) and it can be monitored per-procedurally using computed tomography [Citation32].
Irreversible electroporation (IRE)
IRE is a new ablation modality that uses pulsed electric fields to induce cell death. The technology behind IRE has been in use since the 1960s [Citation33,Citation34].
This modality induces an opening of the pores of the cell membrane, that can be reversible or irreversible (depending on the electric potential), and induces cell death. In 2005, Davalos et al. [Citation35] first demonstrated the usefulness of IRE as an ablation modality, describing significant ablation of liver tissue with no or few thermal effects. The device can deliver up to 3,000 V and 50 A through either unipolar or bipolar needle electrodes and an indefinite number of electrodes can be used.
When using unipolar electrodes, the probes have to be as parallel as possible to achieve a predictable ablation zone. Ablation size can be influenced by length of the active tip (0.5–4 cm), pulse number (typically 70–90), duration of pulses (typically 90–100 µs), distance between probes, and voltage applied.
The procedure is performed under general anesthesia with complete muscle blockade. The patient’s electrocardiogram should be synchronized to the IRE device, to prevent dysrhythmias during the intervention.
The nonthermal nature of IRE has some advantages compared with thermal ablation [Citation36] as it seems not limited by the heat sink effect [Citation37] and it has no significant effect on connective tissue, allowing ablation of tumors close to vulnerable structures such as nerves [Citation38] or bile ducts [Citation37].
Comparison according to the ablation modality
Cryoablation does not result in substantial collagen damage and appears to be a better option for patients with extensive emphysema [Citation39] and for lesions that are close to the pleura or diaphragm, where other modalities may cause pain or diaphragmatic rupture [Citation40]. Moreover, rapid involution of ice-ball facilitates identification of local treatment failure, with most local tumor progression, or incomplete ablation, visualized by 6-month CT () [Citation30].
Table 1. Main differences according to ablation technic for lung metastases treatment.
Nevertheless, this technique is more expensive and takes more time.
MWA creates larger ablation zone than RFA and is less affected by heat sink effect, which makes it a better fit for patients who have larger tumors or tumors that are close to larger vessels to provide complete ablations [Citation19]. The diffusion of energy lays on the permittivity of each tissue [Citation41]. The organs the more favorable at this treatment are those with a stronger difference of permittivity, i.e. the lung with the air and the breast with the fat.
Radiofrequency has some advantages as well, such as a lower cost and a relatively spherical ablation zone, which may be effective for medium-sized tumors (up to 2.5 cm) that can be covered with a single electrode.
Electroporation is a non-thermic ablation technique, so it does not seem to have a significant effect on connective tissue, allowing ablation of tumors adjacent to sensitive structures such as nerves [Citation38] and bile ducts [Citation37] Then, the possibility to have areas of reversible and IRE creates the possibility of associating intra-tumoral drug delivery to ablation [Citation42].
All these techniques have been already widely used in several settings for percutaneous ablation.
A vast literature has been produced on radiofrequency and microwave, firstly used in liver ablation [Citation43] and then to treat tumors of others locations, such as kidney, bone) and thyroid [Citation44,Citation45] among the others.
Cryoablation found its best success and most common indication in treating renal lesions [Citation46], but some series validated its’ use on desmoid or bone tumors [Citation47].
IRE, still in its earlier phase of development, seems promising in treating lesion near or within noble structures, such as lesion within the pancreas [Citation48], close to the bile duct or ureteral ducts [Citation49].
Anesthesiologic management
Procedures are generally performed under general anesthesia (GA) as hyperthermal percutaneous ablations can be painful. Insufficient pain control may result in operator hurrying or the need to stop the thermoablation procedures, which may subsequently result in an incomplete ablation, especially in case of lesion close to the pleural surface or near the hilum, more prone to provoke pain [Citation50].
During GA a preventive paravertebral block (TPVB) can be performed to prevent parietal pain [Citation51]. In addition, general anesthesia allows a better control of respiratory movements, such as the use of high frequency jet ventilation, and an early detection of possible gaseous embolisms [Citation52].
Cryoablation, should be preferred when GA is contraindicated, for tumors near the pleura, as it seems less painful than the others techniques [Citation53].
Image guidance
All these interventions are performed under image-guidance. CT scan is the main modality but, since the development of the CT-fluoroscopy and Cone-beam CT, procedures can be performed using either of this guidance systems [Citation54]. Nevertheless, in case of difficult accesses to the lesion or when noble organs are close to it, a iatrogenic pneumothorax could be necessary to perform a safer procedure [Citation52]. Cryoablation enables interventionalists to stick the probe in the tumor after a few seconds of freezing, giving the possibility to move the tumor away from vulnerable structures in the vicinity or, conversely, to avoid displacement during treatment. The same possibility is given by the RF expandable needles that, in fact, are often used in lung ablations (). In these cases, a CT guidance seems more appropriate as an almost real time, high resolution imaging technology [Citation54]. Furthermore, Positron Emission tomography has been recently developed in additional tool for guiding percutaneous treatment.
Figure 4. A. Left para cardiac nodule (circle) before treatement with RFA. B. Use of deployable needle (arrowhead) with multiple tips (white arrows) to catch the nodule (black arrow) after performing an artificial pneumothorax (asterisk). C & D. Once the needle tips (white arrows) are deployed in the nodule (asterisk), the probe (arrowhead) is retracted, in order to create a space between the heart bordert and the lesion, to reduce the heat sink effect and avoid major damages to the surrounding structures.
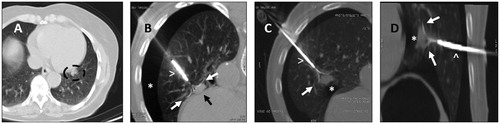
Specifically, the opportunity to detect the hypermetabolic part of lung lesion can rise the accuracy of the needle positioning and the subsequent ablation. Moreover, recently several navigation systems have been developed, allowing the operator to reach lesion located in a difficult anatomical spaces such as apexes, hilar or cardio-phrenic angle [Citation55–58].
Post-procedural management and follow-up
Post-procedural management and follow-up depend on the protocols and habits of each institution, although there are some cornerstones that have to be verified.
Post-procedural management
Depending on whether the intervention is done under general anesthesia or conscious sedation, the occurrence of complications, the number of lesions to treat and the general status and co-morbidities of each patient, percutaneous ablation of lung metastases can be performed as an ‘outpatient procedure’, or may require a short hospital stay (24–48 h in most of the cases).
Immediate post-procedural imaging needs to be done within a few minutes after the ablation, to confirm the technical success of the procedure and depict possible complications requiring a specific management [Citation59].
Post-ablation aspect, for all ablation technologies, often demonstrates an area of central dense opacity enclosed by an area of ground-glass opacity. At the borders of this zone there is a denser halo marking with a sharp limit with the surrounding lung parenchyma. The ablation zone (cell death induced by the ablation) is slightly smaller than the ground-glass opacity area. Thus, local tumor recurrence can occur within 3 mm of this margin in all three dimensions [Citation60].
An ablation zone covering a margin of 5–10 mm beyond the visible tumor border is suggested [Citation61]. The actual ablation zone may be estimated by the extent of ground glass opacity surrounding the lesion during RFA. A 5-mm ground glass rim covering the lesion in the immediate post-procedural CT images suggests a good chance of complete treatment [Citation60]. For large tumors, multiple overlapping ablations are used to achieve an adequate ablation zone.
Complications
RFA is the most reported percutaneous ablation technique for lung tumors. As the other percutaneous ablation technics, It has a very good safety profile with very low mortality (<0.5%) and no alteration of the respiratory function. Peri-procedural morbidity ranges from 15.5% to 55.6%, with 8–12% being major complications [Citation62].
Pneumothorax [Citation63] is the most common adverse event, occurring in up to 67% of patients (). About 20% do not requiring any specific treatment; 20% can be managed with aspiration only; the rest of the patients will need a chest tube insertion; and very rare proportion will need surgical pleurodesis [Citation63].
Infectious complications need to be treated carefully and very early to avoid abscess, or later complications.
Mild adverse events such as post-procedural pain (treated by oral analgesic), aseptic pleural effusions not requiring drainage or rib fracture have been reported [Citation63] for RFA and MWA.
Hemorrhagic complications occur in up to 15% of patients, and are dominated by self-limiting alveolar hemorrhage, hemoptysis or haemothorax. Less than 1% of the cases require blood transfusion and coil embolization of the intercostal, bronchial or pulmonary arteries (false aneurysm) [Citation63].
Other extremely rare complications include nerve or other adjacent organs injuries, broncho-pleural fistula, seeding or skin burns (ground pads positioning or prosthetic material, or ablation reaching the chest wall) [Citation22].
Follow-up
Long-term follow-up is mandatory for patients after percutaneous ablation of lung metastases, as recurrence can be detected even 2–3 years after ablation. It can be done every 3 months by the interventional oncologist and/or the medical oncologist in charge of the patient for the first year, then every 6 months [Citation64].
The baseline post-ablation imaging is performed from 24 h to 4 weeks after ablation. After this time, any increase in size of the ablation zone must be considered as local tumor progression.
During follow-up, there will be a slow involution of the ablation zone. For RFA the reported decrease in size is about 11.4% in 6 months, and 40% at 1 year [Citation65]. Cryoablation produces a faster involution of the size of the ablated area that facilitates identification of local treatment failure. Most local tumor progression, or incomplete ablation can be visualized within 6 months [Citation32].
The most common involution patterns after percutaneous ablations are [Citation66] ‘nodular’: the ablation zone remains spherical and slowly decreases in size (over 18 months); ‘fibrotic’: the ablation becomes elongated and linear; ‘cavitation’ could be incomplete and only in one part of the ablated volume.
The fibrotic process is more common after MWA and is associated with a lower rate of local recurrence. It is also more common when the area of ablation is in contact with a segmental bronchus.
Atelectasis or disappearance of the ablated area can be noticed at long-term follow-up of all ablation technics and, in case of IRE, can be appreciated also in the early follow-up (within 0–6 months).
Half of local progressions are depicted during the first year of follow-up, and in a series on 1,037 treated metastases reported by de Baere et al. [Citation8], local tumor progression was 5.9%, 8.5%, 10.2% and 11% at 1, 2, 3 and 4 years, respectively. Although some groups use enhanced (multiple phase) CT scans, most of the reports of lung thermal ablation rely on morphological changes (depicted on non-enhanced CT) for evaluation of treatment efficacy.
Finally, to overcome limitations of morphological imaging, several studies have evaluated the role of PET-CT [Citation67], most of them reporting promising results and encouraging the use of PET-CT in follow-up strategies. Particular attention should be paid to exams in the first six months after ablations, as there is a risk of false positive exams due to early inflammation processes mimicking an active tumor, or false negative results due to early inflammation masking active residual tumor.
Nowadays, because of its cost and the drawbacks mentioned previously, PET-CT is performed 6 months post ablation in case of non-univocal CT scan results [Citation68].
A study by Jin et al. reported that the ablation zone is commonly enlarged in the immediate follow-up, and tends to decrease in size at 3, 6, 9 and 12 months by 6%, 11%, 14%, and 40%, respectively [Citation65]. So, it is better to judge the response at 3 months than at 1 month.
When performing enhanced CT, focal nodular enhancements of more than 15 Hounsfield units (HU) have to be considered as a local tumor progression [Citation69].
Outcome
For lung metastases, survival rates are comparable to those obtained after surgery. A 4-year local efficacy of 89% has been reported by both Lencioni et al. [Citation70] and de Baere et al. [Citation8] in two multicenter prospective trials enrolling 61 and 566 patients, respectively. This high local rate of control, due to strict patient selection, leads to global control of the disease. The largest prospective report of lung RFA for metastases on 1,037 lung metastases (52% being colorectal metastases) shows a median OS of 62 months and 1-, 2-, 3-, 4- and 5-years OS rates of 92.4%, 79.4%, 67.7%, 58.9% and 51.5%, respectively. In comparison, OS rates are between 27% and 68% at 5 years in a meta-analysis pooling 2,925 lung colorectal metastases [Citation25] treated by surgery and of 53.5% in a multicenter registry [Citation4,Citation8].
Furthermore, the challenge of disease control for lung metastatic patients is related to the repeatability of these treatments, as progression-free-survival (PFS) is low, and most patients will progress over time in a site distant from that initially treated but still in the lung. De Baere et al. [Citation8] reports 24% of re-treatments (up to 4) of the initially treated patients producing a 4-year control rate of lung metastatic disease of 44.1%.
So, patient selection must be done very carefully, as several factors have been reported to influence the rate of ablation success; i.e. tumors between 2 and 3 cm; proximity/contact with vessels (>3 mm of diameter) is a predictive factor for incomplete local treatment, the entire covering of the tumor by the ground-glass opacity induced by the ablation, and the margins (a ratio <4 in between the area of RFA-induced ground-glass opacity and the tumor is a risk factor for recurrence [Citation71]).
In a recent publication, Nour-Eldin et al. [Citation72] report an advantage of MWA over RFA in terms of local recurrence and PFS in a series comparing chronologically LITT (year 2000–2003), RFA (2004–2008) and MWA (2008–2014) procedures in 109 patients. In comparison MWA was significantly more effective than RFA (p = 0.032), with a 4-year OS of 45.4% vs. 24.2% respectively, this last being lower reported in the literature but with less strict inclusion criteria. Finally, more recent technologies of MWA systems seem to improve the reproducibility and sphericity of the ablation zone making this technology easier to use in everyday practice.
In a phase 2 multicenter prospective study [Citation30] evaluating 40 patients with 60 metastases measuring 1.4 ± 0.7 cm [0.3–3.4 cm], cryoablation has been reported using a mean of 1.7 probes per patient with a promising 94.2% local tumor control at 12 months. Prolonged follow-up of the same study is ongoing, and recruitment for a larger trial of 130 patients have been completed recently.
IRE is a relatively recent technique, short-term oncological results are promising, but more complementary data on remote oncology follow-up are needed [Citation73]. On the periphery of the IRE ablation zone, there could be a reversible electroporation zone that would allow the association with drug treatments directly delivered in the lesion [Citation33,Citation73]. Nevertheless, early clinical results in lung ablation with IRE close to large vessels have been disappointing with 61% local recurrence in a multi-institutional study of 20 patients [Citation73]. This unexpectedly high recurrence rate was hypothesized to be due to high differences in electrical conductivity between the lung parenchyma and tumor tissue.
Limitations
Ablation techniques have some important limitations. First, because local tumor ablation can destroy only the targeted tumor and does not affect lymph nodes, pre-operative workup needs to be very careful (PET-CT) or combine with other modalities for lymph node metastases, such as radiation therapy. Second, location can be challenging, such as metastasis near the mediastinum and lateral aspect of the lung apex. Some technics have been described to overcome these limitations, such artificial pneumothorax, or tumor displacement using deployable RF needle, or the ‘stick mode’ with cryotherapy.
Conclusion
Percutaneous image-guided ablation of metastatic lung lesions provides high local control rates, with few complications, that may, in selected patients, confer a survival benefit over no treatment or systemic therapy alone. Risk factors for local progression following percutaneous ablation are important to identify and are the subject of numerous recent investigations. Prospective, randomized, trials are still needed to directly compare therapeutic options and further define the role of percutaneous image-guided ablation in the treatment of pulmonary metastatic disease.
Disclosure statement
No potential conflict of interest was reported by the authors.
References
- Herold CJ, Bankier AA, Fleischmann D. Lung metastases. Eur Radiol. 1996;6:596–606. http://www.ncbi.nlm.nih.gov/pubmed/8934121 (accessed May 26, 2019).
- Hess KR, Varadhachary GR, Taylor SH, et al. Metastatic patterns in adenocarcinoma. Cancer. 2006;106:1624–1633.
- Mohammed T-LH, Chowdhry A, Reddy GP, et al. Expert panel on thoracic imaging, ACR Appropriateness Criteria® screening for pulmonary metastases. J Thorac Imaging. 2011;26:W1–W3.
- Casiraghi M, De Pas T, Maisonneuve P, et al. A 10-year single-center experience on 708 lung metastasectomies: the evidence of the international registry of lung metastases. J Thorac Oncol. 2011;6:1373–1378.
- Sethi N, Kang Y. Unravelling the complexity of metastasis – molecular understanding and targeted therapies. Nat Rev Cancer. 2011;11:735–748.
- Quirk MT, Pomykala KL, Suh RD. Current readings: percutaneous ablation for pulmonary metastatic disease. Semin Thorac Cardiovasc Surg. 2014;26:239–248.
- de Baere T, Tselikas L, Gravel G, et al. Lung ablation: best practice/results/response assessment/role alongside other ablative therapies. Clin Radiol. 2017;72:657–664.
- de Baère T, Aupérin A, Deschamps F, et al. Radiofrequency ablation is a valid treatment option for lung metastases: experience in 566 patients with 1037 metastases. Ann Oncol Off J Eur Soc Med Oncol. 2015;26:987–991.
- Pastorino U, Buyse M, Friedel G, et al. International registry of lung metastases, long-term results of lung metastasectomy: prognostic analyses based on 5206 cases. J Thorac Cardiovasc Surg. 1997;113:37–49. http://www.ncbi.nlm.nih.gov/pubmed/9011700 (accessed May 26, 2019).
- Patel IJ, Davidson JC, Nikolic B, Standards of Practice Committee, with Cardiovascular and Interventional Radiological Society of Europe (CIRSE) Endorsement, et al. Consensus guidelines for periprocedural management of coagulation status and hemostasis risk in percutaneous image-guided interventions. J Vasc Interv Radiol. 2012;23:727–736.
- Pereira PL, Salvatore M, Salvatore M, Cardiovascular and Interventional Radiological Society of Europe (CIRSE). Standards of practice: guidelines for thermal ablation of primary and secondary lung tumors. Cardiovasc Intervent Radiol. 2012;35:247–254.
- Hess A, Palussière J, Goyers J-F, et al. Pulmonary radiofrequency ablation in patients with a single lung: feasibility, efficacy, and tolerance. Radiology. 2011;258:635–642.
- Grieco CA, Simon CJ, Mayo-Smith WW, et al. Percutaneous image-guided thermal ablation and radiation therapy: outcomes of combined treatment for 41 patients with inoperable stage I/II non-small-cell lung cancer. J Vasc Interv Radiol. 2006;17:1117–1124.
- Dupuy DE, Zagoria RJ, Akerley W, et al. Percutaneous radiofrequency ablation of malignancies in the lung. AJR Am J Roentgenol. 2000;174:57–59.
- Hiraki T, Mimura H, Gobara H, et al. Two cases of needle-tract seeding after percutaneous radiofrequency ablation for lung cancer. J Vasc Interv Radiol. 2009;20:415–418.
- Vogl TJ, Nour-Eldin N-EA, Hammerstingl RM, et al. Microwave ablation (MWA): basics, technique and results in primary and metastatic liver neoplasms - review article. Fortschr Röntgenstr. 2017;189:1055–1066.
- Wright AS, Lee FT, Mahvi DM. Hepatic microwave ablation with multiple antennae results in synergistically larger zones of coagulation necrosis. Ann Surg Oncol. 2003;10:275–283.
- Liang P, Wang Y, Yu X, et al. Malignant liver tumors: treatment with percutaneous microwave ablation – complications among cohort of 1136 patients. Radiology. 2009;251:933–940.
- Brace CL. Radiofrequency and microwave ablation of the liver, lung, kidney, and bone: what are the differences? Curr Probl Diagn Radiol. 2009;38:135–143.
- Wolf FJ, Grand DJ, Machan JT, et al. Microwave ablation of lung malignancies: effectiveness, CT findings, and safety in 50 patients. Radiology. 2008;247:871–879.
- Goldberg SN, Gazelle GS, Mueller PR. Thermal ablation therapy for focal malignancy: a unified approach to underlying principles, techniques, and diagnostic imaging guidance. AJR Am J Roentgenol. 2000;174:323–331.
- Yang D, Converse MC, Mahvi DM, et al. Measurement and analysis of tissue temperature during microwave liver ablation. IEEE Trans Biomed Eng. 2007;54:150–155.
- Brace CL. Microwave ablation technology: what every user should know. Curr Probl Diagn Radiol. 2009;38:61.
- Ye X, Fan W, Chen J, et al. Chinese expert consensus workshop report: Guidelines for thermal ablation of primary and metastatic lung tumors. Thorac Cancer. 2015;6:112–121.
- de Freitas RMC, Andrade CS, Caldas JGMP, et al. Image-guided cryoablation of the spine in a swine model: clinical, radiological, and pathological findings with light and electron microscopy. Cardiovasc Intervent Radiol. 2015;38:1261–1270.
- Gage AA, Baust J. Mechanisms of tissue injury in cryosurgery. Cryobiology. 1998;37:171–186.
- Guan L, Wang J, Gao K, et al. Percutaneous intraductal radiofrequency ablation combined with biliary stent placement for malignant biliary obstruction: a case report and review of the literature. J Cancer Res Ther. 2016;12:221–224.
- Waitz R, Solomon SB, Petre EN, et al. Potent induction of tumor immunity by combining tumor cryoablation with anti-CTLA-4 therapy. Cancer Res. 2012;72:430–439.
- Littrup PJ, Mody A, Sparschu R, et al. Prostatic cryotherapy: ultrasonographic and pathologic correlation in the canine model. Urology. 1994;44:175–183.
- de Baere T, Tselikas L, Woodrum D, et al. Evaluating cryoablation of metastatic lung tumors in patients-safety and efficacy: the ECLIPSE Trial-interim analysis at 1 year. J Thorac Oncol. 2015;10:1468–1474.
- Hinshaw JL, Littrup PJ, Durick N, et al. Optimizing the protocol for pulmonary cryoablation: a comparison of a dual- and triple-freeze protocol. Cardiovasc Intervent Radiol. 2010;33:1180–1185.
- Ito N, Nakatsuka S, Inoue M, et al. Computed tomographic appearance of lung tumors treated with percutaneous cryoablation. J Vasc Interv Radiol. 2012;23:1043–1052.
- Silk M, Tahour D, Srimathveeravalli G, et al. The state of irreversible electroporation in interventional oncology. Semin Intervent Radiol. 2014;31:111–117.
- Coster HGL. A quantitative analysis of the voltage-current relationships of fixed charge membranes and the associated property of ‘punch-through’. Biophys J. 1965;5:669–686.
- Davalos RV, Mir ILM, Rubinsky B. Tissue ablation with irreversible electroporation. Ann Biomed Eng. 2005;33:223–231.
- Breton M, Mir LM. Microsecond and nanosecond electric pulses in cancer treatments. Bioelectromagnetics. 2012;33:106–123.
- Charpentier KP, Wolf F, Noble L, et al. Irreversible electroporation of the liver and liver hilum in swine. HPB (Oxford). 2011;13:168–173.
- Schoellnast H, Monette S, Ezell PC, et al. Acute and subacute effects of irreversible electroporation on nerves: experimental study in a pig model. Radiology. 2011;260:421–427.
- Zemlyak A, Moore WH, Bilfinger TV. Comparison of survival after sublobar resections and ablative therapies for stage I non-small cell lung cancer. J Am Coll Surg. 2010;211:68–72.
- Chen C-K, Chou T-Y, Chen C-B, et al. Image-guided cryoablation for metastatic diaphragmatic thymoma. J Vasc Interv Radiol. 2012;23:1097–1099.
- Stuchly MA, Athey TW, Stuchly SS, et al. Dielectric properties of animal tissues in vivo at frequencies 10 MHz–1 GHz. Bioelectromagnetics. 1981;2:93–103.
- de Baere T. New techniques of tumor ablation (microwaves, electroporation)]. J Radiol. 2011;92:789–795.
- de Baere T, Tselikas L, Pearson E, et al. Interventional oncology for liver and lung metastases from colorectal cancer: the current state of the art. Diagn Interv Imaging. 2015;96:647–654.
- Pescatori LC, Torcia P, Nicosia L, et al. Which needle in the treatment of thyroid nodules? Gland Surg. 2018;7:111.
- De Filippo M, Russo U, Papapietro VR, et al. Radiofrequency ablation of osteoid osteoma. Acta Biomed. 2018;89:175–185.
- Higgins LJ, Hong K. Renal ablation techniques: state of the art. AJR Am J Roentgenol. 2015;205:735–741.
- Havez M, Lippa N, Al-Ammari S, et al. Percutaneous image-guided cryoablation in inoperable extra-abdominal desmoid tumors: a study of tolerability and efficacy. Cardiovasc Intervent Radiol. 2014;37:1500–1506.
- O’Brien TJ, Passeri M, Lorenzo MF, et al. Experimental high-frequency irreversible electroporation using a single-needle delivery approach for nonthermal pancreatic ablation in vivo. J Vasc Interv Radiol. 2019;30:854–862.e7.
- Vroomen LGPH, Petre EN, Cornelis FH, et al. Irreversible electroporation and thermal ablation of tumors in the liver, lung, kidney and bone: what are the differences? Diagn Interv Imaging. 2017;98:609–617.
- Kim HJ, Park BK, Chung IS. Comparison of general anesthesia and conscious sedation during computed tomography-guided radiofrequency ablation of T1a renal cell carcinoma. Can Assoc Radiol J. 2018;69:24–29.
- Gazzera C, Fonio P, Faletti R, et al. Role of paravertebral block anaesthesia during percutaneous transhepatic thermoablation. Radiol Med. 2014;119:549–557.
- Palussière J, Catena V, Gaubert J-Y, et al. Percutaneous lung thermo-ablation. Bull Cancer. 2017;104:417–422.
- Sharma A, Abtin F, Shepard J-AO. Image-guided ablative therapies for lung cancer. Radiol Clin North Am. 2012;50:975–999.
- Cazzato RL, Battistuzzi J-B, Catena V, et al. Cone-beam computed tomography (CBCT) versus CT in lung ablation procedure: which is faster? Cardiovasc Intervent Radiol. 2015;38:1231–1236.
- Mauri G, Gennaro N, De Beni S, et al. Real-time US-18FDG-PET/CT image fusion for guidance of thermal ablation of 18FDG-PET-positive liver metastases: the added value of contrast enhancement. Cardiovasc Intervent Radiol. 2019;42:60–68.
- Mauri G. Expanding role of virtual navigation and fusion imaging in percutaneous biopsies and ablation. Abdom Imaging. 2015;40:3238–3239.
- Mauri G, Solbiati L. Virtual navigation and fusion imaging in percutaneous ablations in the neck. Ultrasound Med Biol. 2015;41:898.
- Mauri G, Cova L, De Beni S, et al. Real-time US-CT/MRI image fusion for guidance of thermal ablation of liver tumors undetectable with US: results in 295 cases. Cardiovasc Intervent Radiol. 2015;38:143–151.
- de Baère T, Palussière J, Aupérin A, et al. Midterm local efficacy and survival after radiofrequency ablation of lung tumors with minimum follow-up of 1 year: prospective evaluation. Radiology. 2006;240:587–596.
- Lee JM, Jin GY, Goldberg SN, et al. Percutaneous radiofrequency ablation for inoperable non-small cell lung cancer and metastases: preliminary report. Radiology. 2004;230:125–134.
- Giraud P, Antoine M, Larrouy A, et al. Evaluation of microscopic tumor extension in non-small-cell lung cancer for three-dimensional conformal radiotherapy planning. Int J Radiat Oncol Biol Phys. 2000;48:1015–1024.
- Kashima M, Yamakado K, Takaki H, et al. Complications after 1000 lung radiofrequency ablation sessions in 420 patients: a single center’s experiences. AJR Am J Roentgenol. 2011;197:W576–W580.
- Hiraki T, Tajiri N, Mimura H, et al. Pneumothorax, pleural effusion, and chest tube placement after radiofrequency ablation of lung tumors: incidence and risk factors. Radiology. 2006;241:275–283.
- Abtin FG, Eradat J, Gutierrez AJ, et al. Radiofrequency ablation of lung tumors: imaging features of the postablation zone. Radiographics. 2012;32:947–969.
- Jin GY, Lee JM, Lee YC, et al. Primary and secondary lung malignancies treated with percutaneous radiofrequency ablation: evaluation with follow-up helical CT. AJR Am J Roentgenol. 2004;183:1013–1020.
- Palussière J, Marcet B, Descat E, et al. Lung tumors treated with percutaneous radiofrequency ablation: computed tomography imaging follow-up. Cardiovasc Intervent Radiol. 2011;34:989–997.
- Bonichon F, Palussière J, Godbert Y, et al. Diagnostic accuracy of 18F-FDG PET/CT for assessing response to radiofrequency ablation treatment in lung metastases: a multicentre prospective study. Eur J Nucl Med Mol Imaging. 2013;40:1817–1827.
- Deandreis D, Leboulleux S, Dromain C, et al. Role of FDG PET/CT and chest CT in the follow-up of lung lesions treated with radiofrequency ablation. Radiology. 2011;258:270–276.
- Hiraki T, Gobara H, Mimura H, et al. Percutaneous radiofrequency ablation of clinical stage I non-small cell lung cancer. J Thorac Cardiovasc Surg. 2011;142:24–30.
- Lencioni R, Crocetti L, Cioni R, et al. Response to radiofrequency ablation of pulmonary tumours: a prospective, intention-to-treat, multicentre clinical trial (the RAPTURE study). Lancet Oncol. 2008;9:621–628.
- de Baere T, Robinson JM, Rao P, et al. Radiofrequency ablation of lung metastases close to large vessels during vascular occlusion: preliminary experience. J Vasc Interv Radiol. 2011;22:749–754.
- Nour-Eldin N-EA, Exner S, Al-Subhi M, et al. Ablation therapy of non-colorectal cancer lung metastases: retrospective analysis of tumour response post-laser-induced interstitial thermotherapy (LITT), radiofrequency ablation (RFA) and microwave ablation (MWA). Int J Hyperthermia. 2017;33:820–829.
- Ricke J, Jürgens JHW, Deschamps F, et al. Irreversible electroporation (IRE) fails to demonstrate efficacy in a prospective multicenter phase II trial on lung malignancies: the ALICE trial. Cardiovasc Intervent Radiol. 2015;38:401–408.