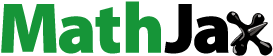
Abstract
Objective: Hyperthermia therapy (HT), heating tumors to 40–45 °C, is a known radiotherapy (RT) and chemotherapy sensitizer. The additional benefit of HT to RT for recurrent breast cancer has been proven in multiple randomized trials. However, published outcome after RT + HT varies widely. We performed a systematic review to investigate whether there is a relationship between achieved HT dose and clinical outcome and thermal toxicity for patients with recurrent breast cancer treated with RT + HT.
Method: Four databases, EMBASE, PubMed, Cochrane library and clinicaltrials.gov, were searched with the terms breast, radiotherapy, hyperthermia therapy and their synonyms. Final search was performed on 3 April 2019. Twenty-two articles were included in the systematic review, reporting on 2330 patients with breast cancer treated with RT + HT.
Results: Thirty-two HT parameters were tested for a relationship with clinical outcome. In studies reporting a relationship, the relationship was significant for complete response in 10/15 studies, in 10/13 studies for duration of local control, in 2/2 studies for overall survival and in 7/11 studies for thermal toxicity. Patients who received high thermal dose had on average 34% (range 27%–53%) more complete responses than patients who received low thermal dose. Patients who achieved higher HT parameters had increased odds/probability on improved clinical outcome and on thermal toxicity.
Conclusion: Temperature and thermal dose during HT had significant influence on complete response, duration of local control, overall survival and thermal toxicity of patients with recurrent breast cancer treated with RT + HT. Higher temperature and thermal dose improved outcome, while higher maximum temperature increased incidence of thermal toxicity.
Introduction
Hyperthermia therapy (HT), increasing the tumor temperature to 40–45 °C, is a known radiotherapy (RT) and chemotherapy sensitizer. The additional benefit of HT to RT and chemotherapy has been proven in randomized trials for melanoma, sarcoma, recurrent breast cancer, cervical cancer and other tumor types [Citation1–5]. A recent systematic review showed the additional value of HT to RT for patients with locoregional recurrent breast cancer [Citation6]. Complete response rate increased from 38.1% for patients treated with RT to 60.2% for patients treated with RT + HT, respectively. The complete response of RT + HT varied widely throughout the included studies; range 33.3%–95.0% [Citation7,Citation8]. An important factor contributing to this wide variation was hypothesized to be the variability in HT delivery, that is the HT technique, sequencing of RT and HT, duration of HT and temperature and thermal dose achieved during HT treatment [Citation6].
Techniques used in the past and in the present vary in effective field size and penetration depth depending on the design and used frequency of the technique [Citation9], resulting in variations in temperature and thermal dose achieved during HT treatment. Furthermore, the amount of radiosensitization by HT is strongly dependent on the sequence and time-interval between RT and HT, where the effect is largest when HT and RT are given simultaneously [Citation10,Citation11]. This maximum radiosensitization for simultaneous application is observed for both tumor and normal tissue.
A thermal dose–effect relationship for HT has been reported in the literature, both in pre-clinical and clinical studies. In vitro studies show that there is an increase in cell death when tumor cells are heated longer or to a higher temperature [Citation12–14]. Several clinical studies underline the importance of duration of HT and temperature and thermal dose achieved during HT treatment [Citation15,Citation16]. The effect of HT increases with a longer heating time, but during and after exposure to heating the onset of thermotolerance is induced, which results in a temporarily reduced sensitivity to heating [Citation17,Citation18]. This reduced effectiveness of HT is a transient effect and persists for several days [Citation18]. Therefore, clinical HT treatments are limited to 1–1.5 h, once or twice weekly. As a result of the observed thermal dose-effect relationship, HT treatments are usually quantified by calculating a ‘thermal isoeffective dose’, which captures both temperature and time. The most commonly used thermal isoeffective dose is the number of equivalent minutes at 43 °C (CEM43) [Citation19,Citation20]. Other parameters representing minimum or median tumor temperature levels achieved during HT, include T90, T50, and TRISE [Citation21–23].
We performed a systematic review to determine whether the large variation in outcome observed in clinical studies for patients with recurrent breast cancer treated with RT + HT can be explained by variation in achieved temperatures and thermal dose during HT treatment.
Methods
The systematic review was conducted following the PRISMA guidelines [Citation24]. Four databases were searched from inception to 3 April 2019: EMBASE, PubMed, Cochrane library and clinicaltrials.gov. An initial search was performed in PubMed, and relevant articles were found by citation tracking. The synonyms for breast, hyperthermia therapy and radiotherapy reported in those articles were used as entrance parameters in the final search. For each database an individual search was performed. In PubMed the following search was executed: (‘Breast’[Mesh] or breast[tw] or mamma[tw] or mammary[tw]) AND (‘Hyperthermia, Induced’[Mesh] or hyperthermia[tw] or heat[tw] or thermotherapy[tw] or thermoradiotherapy[tw]) AND (‘Combined Modality Therapy’[Mesh] or ‘Radiotherapy’[Mesh] or radio[tw] or radiation[tw] or irradiation[tw] or reirradiation[tw] or re-irradiation[tw] or radiotherapy[tw] or thermoradiotherapy[tw]). In clinicaltrials.gov studies for breast cancer and hyperthermia were searched, while a filter for the intervention type, that is not drugs, was applied. The search was not limited to any date, both articles and conference proceedings were allowed, a filter for English articles and human studies was applied. When warranted we contacted study authors to identify additional studies.
Inclusion criteria
Single-arm, double-arm, retrospective and prospective studies (randomized and non-randomized) fulfilling the following criteria were included: Patients with recurrent breast cancer treated with external beam RT and local HT.
Study selection
After exclusion of duplicates, articles were screened according to their titles and abstracts (). Articles were excluded when:
Figure 1. PRISMA flow diagram of the literature search [Citation24].
![Figure 1. PRISMA flow diagram of the literature search [Citation24].](/cms/asset/43ebd610-9895-4e6e-b3dd-79f75e3bf65d/ihyt_a_1665718_f0001_c.jpg)
Articles were not clinical studies.
Patients received concurrent chemotherapy or drugs.
The endpoint of the study was not outcome.
The relationship between thermal dose parameters and outcome was not analyzed.
Less than ten patients or lesions with breast cancer treated with RT + HT were reported.
Articles were updated in a later publication by the same author(s).
Mixed patient groups were reported and the outcomes of breast cancer patients were not analyzed separately.
Articles from the same authors describing the temperature analysis of the HT treatment in more detail were taken into account.
Data extraction and quality assessment
The primary endpoints of interest were the relationships between temperature and/or thermal dose during HT with clinical outcome: complete response (CR), duration of local control (LC), overall survival (OS) as well as thermal toxicity (i.e., acute blisters). Details of the (pre) treatment patient characteristics and present treatment parameters were investigated, as well as clinical outcome.
The relationships between thermal dose parameters and CR, LC, OS, and thermal toxicity were tested in univariate and multivariate analysis. A p value<.05 was considered significant, p between .05 and .1 a trend and for p ≥ .1 we concluded that there was no relationship. Furthermore, we registered whether a parameter was mentioned by the authors but not reported as related to outcome, which we interpreted as that the analysis was either not performed or not reported. This selective reporting within studies is a possible publication bias. An overview of the relationships of thermal dose parameters to CR, LC, OS, and thermal toxicity is presented in a graph. In this graph, the thermal dose parameters are grouped in categories as either high-end temperature (e.g., maximum temperature, T10, T20), mean temperature (e.g., mean and median temperature), and low-end temperature (e.g., T90 and minimum temperature) and as either high-end dose (e.g., maximum CEM43, time > 43 °C), mean dose (e.g., CEM43T50) and low-end dose (e.g., CEM43T90, minimum CEM43, time < 40 °C). The number of HT treatments is considered as a separate category. T10, T20, and T90 are the 90th, 80th, and 10th percentile of all temperature measurements, respectively. For each category, a study is taken into account once. We selected the parameter that had the strongest relationship with the outcome variables.
The odds ratio (OR), risk ratio (RR) and risk difference (RD) was calculated for studies which reported the clinical outcome measures for patients grouped based on the value of their thermal dose parameters during HT treatment. The OR and RR are expressed as the logarithmic average with the 95% confidence interval (CI). The RD is expressed as the average with the 95% CI. When one of the events was zero, for example, patients with a low thermal dose had no CR, we first added 0.5 to all categories and then calculated the OR, RR, or RD (Haldane-Anscombe correction) [Citation25]. The statistical analysis was performed in R (version 3.5.1) with package ‘metafor’ (version 2.0-0). No meta-analysis was performed due to the strong variation in definitions of thermal dose parameters.
The study selection and risk of bias assessment (QUIPS) [Citation26] were performed by author AB. Data extraction was done by authors JZ and AB.
Results
A total of 1249 articles were identified through the search and were screened (). Twenty-five studies described in 22 articles were included in the systematic review, reporting a total of 2330 patients with breast cancer. Two additional articles presenting a detailed temperature analysis of the studies presented by Vernon et al. [Citation4] were taken into account [Citation15,Citation27].
Patient and treatment characteristics
In total 1749 patients with macroscopic disease (77.0%) and 563 patients with microscopic disease (23.0%) were reported. Most patients were treated for recurrent disease (n = 2304, 98.9%), while 26 patients (1.1%) were treated for primary disease. Patients received RT + HT for the breast (n = 256), chest wall (n = 1861), and lymph nodes (n = 181) ().
Table 1. Salient features and results of the assessment on the risk of bias [Citation26] of the 22 articles, pertaining to 25 individual studies that have been included in the systematic review.
Most patients had previously received extensive treatment on the areas treated with RT + HT. RT was previously given with a median dose of 50.0 Gy (range 32–65) to 2007 patients (86.1%). At least 1773 patients (76.1%) underwent surgery in the currently treated area, 1319 patients (56.6%) received prior chemotherapy and 971 patients (41.7%) received prior hormonal therapy.
During RT + HT treatment, patients were treated with a median RT dose of 36 Gy (range 29–56) and either microwave HT (n = 2155), radiofrequency HT (n = 135), interstitial HT (n = 31), ultrasound HT (n = 26), capacitive HT (n = 24) or with a mix of HT devices with different frequencies (n = 8) (). HT treatments had an average duration of 52.8 ± 10.4 min (SD). HT was given mostly once or twice per week and in one study every 2 weeks. Patients received five HT treatments (median, range 2–10). In one study RT was given after HT (as soon as possible), while in 19 studies HT was given after RT. The time interval between RT and HT varied from <30 min (9 studies), <45 min (3 studies), <60 min (8 studies), 80 min (1 study) to >90 min (1 study). Three studies did not report the time interval between RT and HT ().
Temperature and thermal dose measurement
Temperature during HT was measured invasively in all 25 studies, but not in all patients. The type of thermometry equipment and the number of temperature sensors were poorly reported (). A total of 32 thermal dose parameters were tested for a relationship with outcome.
Thermal dose parameters were derived from superficial temperature sensors, invasive temperature sensors or from a combination. Thermal dose parameters measured superficially showed no relationship with CR, LC, and OS, but did show a relationship with thermal toxicity. Thermal dose parameters derived from invasive measurements correlated with all outcome measures, that is CR, LC, OS, and thermal toxicity (, supplementary materials).
Table 2. The location of temperature sensors (skin and/or invasively) used for calculation of a thermal dose parameter influences the relationship of the parameter with outcome.
Clinical outcome
The log OR, log RR, and RD are above zero for all clinical outcome measures in every study (). Thus, patients achieving a higher thermal dose parameter during HT treatment had an increased odds/probability of CR, longer duration of LC, longer OS and more thermal toxicity. An overview of all reported thermal dose parameters in the included studies with their respective statistical relationships to the outcome measures and their respective thermal dose category is available as supplementary materials.
Figure 2. Forest plots of the (A) odds ratio (OR), (B) risk ratio (RR), and (C) risk difference (RD) for complete response (CR) [Citation4,Citation15,Citation28–34], local control (LC) at 12 months [Citation15,Citation35–38] and * 6 months [Citation33], overall survival (OS) at 18 months [Citation15,Citation31], and thermal toxicity [Citation33,Citation39–41]. The values were calculated from subgroups of patients, which were grouped based on their variation in thermal dose parameters during hyperthermia treatment; thermal dose parameters above a certain cutoff value (high) versus thermal dose parameters below the cutoff value (low). The OR and RR are presented as the logarithmic mean with 95% confidence interval (CI). The RD is presented as the mean with 95% CI. CEM43: cumulative equivalent minutes at 43 °C [Citation19,Citation20], Tmax: maximum temperature; Tmin: minimum temperature; CR: complete response; PR: partial response; NR: no response; LC: local control; Tox: thermal toxicity.
Complete response
Eight studies reported a significant univariate relationship between CR and thermal dose [Citation28–31] or temperature [Citation32,Citation33,Citation39,Citation42] ( and ). Four studies [Citation29,Citation34,Citation35,Citation39] reported a trend (p < .1) between temperature and CR, while two studies did not find any relationship between CR and thermal dose parameters [Citation43,Citation44].
Figure 3. The number of studies where a high-end, mean, or low-end thermal parameter (T), thermal dose parameter (TD) or number of hyperthermia treatments (#HT tr), is reported in relation to complete response, duration of local control, overall survival and thermal toxicity. The univariate or multivariate relationship of the thermal dose parameters with one of the outcome measures is labeled as either a significant relationship (p < .05) a trend (p values between .05 and .1), no relationship (p ≥ .1) or as not done or not reported. The labels inside the bars represent the number of studies.
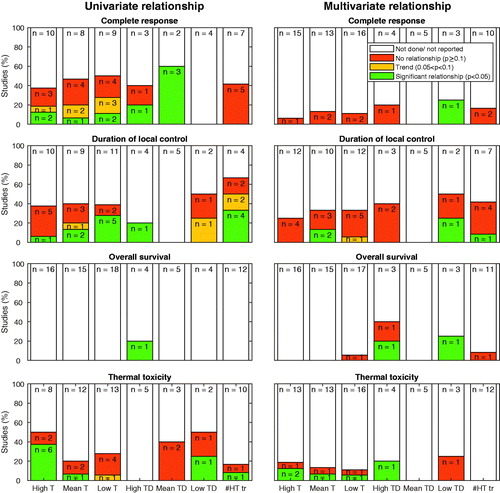
Multiple studies showed a significant relationship between the achieved thermal dose invasively (CEM42.5, CEM43, time > 42.5 °C, time > 43 °C) and CR (). Patients who received a high thermal dose had on average 34% (range 27%–53%) more CR than patients who received a low thermal dose [Citation4,Citation15,Citation28–34].
Figure 4. The relationship between tumor response and thermal dose below/above certain cutoff values (noted between the brackets) [Citation4,Citation15,Citation28–34].
![Figure 4. The relationship between tumor response and thermal dose below/above certain cutoff values (noted between the brackets) [Citation4,Citation15,Citation28–34].](/cms/asset/b353e6fc-c269-43bb-92fd-10a1d56caadb/ihyt_a_1665718_f0004_c.jpg)
Several studies investigated the relationship between temperature and CR. Gabriele et al. [Citation32] found that the CR rate was correlated with the average maximum (skin + invasive) temperature during HT treatment (≥42 °C 76.5% CR vs. <42 °C 30% CR), while there was no correlation with average temperature or minimum temperature (). Similarly, van der Zee et al. [Citation42] showed that the CR rate increased with a higher maximum temperature in normal tissue (p < .04), while neither the maximum temperature in tumor tissue (p = .29) nor the T90 for normal (p = .62) or tumor tissue (p = .30) showed an association with CR. Lindholm et al. [Citation39] found that none of the investigated thermal factors predicted CR. However, for the subgroup treated with 915 MHz (n = 55/59), the minimum temperature in the HT session with the highest temperatures, was predictive for CR (p = .02). Seegenschmiedt et al. [Citation33] also found a significant relationship between the mean minimum temperature, the minimum temperature of the first session and of the best session and CR. A minimum invasive temperature above 41 °C resulted in a significantly better CR. Sannazzari et al. [Citation34] also found that higher minimum temperatures increased the CR rate ().
Both studies that did an in-depth temperature analysis on the trials reported in Vernon et al. [Citation4], found statistically significant multivariate relationships with minimum thermal dose [Citation15,Citation27], after adjusting for maximum tumor depth and RT regimen (radical or palliative RT) [Citation27] and after adjusting for systemic disease at entry and tumor depth [Citation15].
Local control
In univariate analysis, duration of LC was significantly correlated with minimum temperature [Citation33,Citation35,Citation36], T90 [Citation35,Citation40,Citation42] and average temperature [Citation40]. Furthermore, Kapp et al. [Citation45] found that the average percentage of temperatures ≥40 °C was significantly correlated with duration of LC (p < .05; ). Van der Zee et al. [Citation42] also found that duration of LC was improved by a higher maximum normal tissue temperature (p = .02; ). Three studies found no relationship ().
Kapp et al. [Citation35] found that macroscopic tumor lesions that had an average minimum temperature ≥40.75 °C or with <20% temperatures <41 °C had longer LC. In patients with microscopic disease, Kapp et al. [Citation36], confirmed that patients achieving an average minimum temperature ≥39.7 °C or 96% temperatures ≥40 °C resulted in significantly longer LC. Seegenschmiedt et al. [Citation33] found a significant relationship between LC and the mean minimum temperature, the minimum temperature of the first session and of the best session. Where an invasive minimum temperature above 41 °C resulted in significantly better duration of LC [Citation33]. Lee et al. [Citation37] found that patients who achieved temperatures >43 °C for a longer duration showed improved duration of LC [Citation37] ().
Eight studies investigated the relationship between the number of HT treatments and LC. Four found a significant relationship [Citation36,Citation37,Citation41,Citation46] and two a trend [Citation38,Citation39]. Kapp et al., [Citation36] found that ≥2 HT treatments improved duration of LC compared to one treatment in total. Hehr et al. [Citation46] found that >7 HT sessions improved duration of LC. Lee et al. [Citation37] treated patients with a median of eight treatments, where more treatments were better, which remained significant in multivariate analysis. Arcangeli et al. [Citation38] treated one patient with 40 lesions, where a lesion was either treated with one or four HT treatments. When a lesion received four HT treatments there was less tumor regrowth and longer freedom from local progression ().
In multivariate analysis, several thermal dose parameters remained significant for a relationship with duration of LC:
The minimum thermal dose after adjusting for systemic disease at entry [Citation4,Citation15].
The average temperature after adjusting for lesion size and time interval between diagnosis primary tumor and present treatment [Citation39].
The percentage temperature ≥40 °C after adjusting for estrogen receptor status, initial T-stage, time interval between initial breast cancer to first failure, age at HT and concurrent RT [Citation36].
The number of HT treatments and ≥10 min >43° after adjusting for initial specific absorption rate (SAR), current RT dose, tumor volume, and tumor thickness [Citation47].
Overall survival
Both studies investigating the relationship between thermal dose parameters and OS found a positive relationship. Most relevant thermal dose parameters correlating with survival are:
The time >42.5 °C. Refaat et al. [Citation31] reported that when patients were treated with more than 200 min above 42.5 °C, patients had better OS. This remained significant in multivariate analysis after adjusting for microscopic disease, RT dose and mastectomy.
CEM43T100. The DHG, ESHO, and PMH trials [Citation4,Citation15] found in multivariate analyses that OS at 18 months after treatment was significantly better for patients who received more than 6 min CEM43T100 compared to patients who received less than 6 minutes CEM43T100, 57% versus 22%, respectively, after adjusting for systemic disease at entry, age, and tumor area ().
Thermal toxicity
Overall, seven studies report a relationship between toxicity and maximum temperature [Citation33,Citation36,Citation39–41,Citation48,Citation49], of which two studies also report a relationship with thermal dose [Citation41,Citation48]. Furthermore, Lindholm et al. [Citation39] found that two HT treatments per week gave more severe skin reactions than one treatment per week. Linthorst et al. [Citation41] found that the average temperature on the skin had a relationship with thermal toxicity. While four studies report no relationship ( and ) [Citation28,Citation30,Citation42,Citation44].
Several studies showed a relationship between the recorded maximum temperature on the skin and thermal toxicity (). Engin et al. [Citation49] found that patients without a skin reaction had a maximum temperature on the skin of 42.1 ± 0.5 °C (mean ± SD), while patients with thermal blisters had a maximum temperature of 43.7 ± 0.2 °C (p < .01). Seegenschmiedt et al. [Citation33] found that thermal adverse events (superficial blisters and deep burns) were significantly correlated with maximum temperatures of more than 45 °C (p < .01). Lindholm et al. [Citation39] found that one of the prognostic factors for blisters was the highest average maximum skin temperature during a given HT treatment (p = .03). The correlation between normal tissue damage and maximum skin temperature was even stronger (p = .01) when only tumors treated with 915 MHz were considered (n = 55/59). Linthorst et al. [Citation41] found that invasively measured thermal parameters had no influence on thermal toxicity, whereas the incidence of grade 2 and 3 burns clearly increased with a higher maximum skin temperature. Patients with a maximum skin temperature <43 °C had a grade 2 or 3 burn incidence of 15%, while incidence was 32% in patients with a maximum skin temperature >43 °C (p = .006). In 2015, Linthorst et al. [Citation40] again showed that the incidence of grade 2 and 3 skin burns increased with a higher maximum skin temperature; 13% incidence when the maximum skin temperature was <43 °C and 28% when >43 °C (p = .003), see . Likewise, Bakker et al. [Citation48] found in multivariate analysis a significant relationship between the maximum temperature on the skin and the occurrence of thermal skin damage adjusted for patient and tissue type, they derived probability models for skin toxicity for scar and skin tissue ().
Figure 5. The incidence of thermal toxicity (blisters) increases with a higher maximum skin temperature. Data was adapted from Lindholm et al. [Citation39], Linthorst et al. [Citation41], and Linthorst et al. [Citation40] combined with probability models for thermal toxicity for scar and skin tissue [Citation48].
![Figure 5. The incidence of thermal toxicity (blisters) increases with a higher maximum skin temperature. Data was adapted from Lindholm et al. [Citation39], Linthorst et al. [Citation41], and Linthorst et al. [Citation40] combined with probability models for thermal toxicity for scar and skin tissue [Citation48].](/cms/asset/f7692099-328c-43d2-9a59-660f2e15e3bf/ihyt_a_1665718_f0005_c.jpg)
Multivariate analysis by Kapp et al. [Citation36] revealed a higher maximum invasive temperature in fields developing adverse events (cutaneous and/or subcutaneous induration/fibrosis, normal tissue burns/ulceration, edema, pain) compared with fields free of adverse events (45.8 °C vs. 45.2 °C, respectively; p = .005), after adjusting for HT device, tumor stage and site. Furthermore they found a correlation for the percentage of invasive temperature ≥45 °C, after adjusting for HT device, tumor stage and site.
Three studies [Citation30,Citation42,Citation44] found no correlation between thermal parameters and adverse events. Although, van der Zee et al. [Citation42] did find a correlation between the technique used to deliver HT, for example, 434 MHz treatments caused much less acute damage than 2450 MHz treatments (p = .001). They reported that the acute damage for 2450 MHz systems was likely due to having no water bolus cooling. Indeed, when Lindholm et al. [Citation50] added water bolus cooling to a 2450 MHz system, toxicity decreased from 55% to 17%.
Discussion
This systematic review shows that for patients with locoregional recurrent breast cancer treated with RT + HT higher temperature and/or thermal dose during HT improves CR, LC and OS [Citation4,Citation15,Citation27–33,Citation35–37,Citation39,Citation40,Citation42], while increasing the incidence of thermal toxicity [Citation33,Citation36,Citation39–41,Citation48,Citation49]. It is intriguing to see that the CR of patients who received RT alone versus patients who received RT + low dose HT were comparable; 38.1% [Citation6] versus 36.2% (), respectively. Comparable benefit in CR was found when either RT + HT or RT + high dose HT was received; 60.2% [Citation6] versus 66.6% (), respectively. The achieved temperature and thermal dose during HT thus at least partly explains the reported variation in CR after RT + HT in patients with recurrent breast cancer [Citation6].
Optimizing the temperature and thermal dose of HT treatment in patients with locoregional recurrent breast cancer in reirradiated area is therefore of utmost importance. The included studies aimed for minimum temperatures between 41 °C and 43 °C, which were rarely achieved. Whereas the achieved maximum temperature was frequently higher than the beforehand specified allowed maximum temperature, which varied between 43 °C and 50 °C. This implies a very heterogeneous temperature distribution which led to the minimum goal temperatures and allowed maximum temperatures to vary widely between institutes. The recently published ESHO guidelines for superficial HT quality assurance might help to improve overall quality assurance practice and reduce variation between institutes. These guidelines advise to aim for a T90 > 40 °C and T50 > 41 °C to achieve local tumor control, with maximum temperatures of 43–45 °C [Citation51]. Furthermore, hyperthermia techniques have improved over the years and more uniform and adequate heating is presently feasible.
Temperature and thermal dose measurement
Although achieved temperatures and thermal dose of HT treatment determined treatment quality, these treatment parameters were often not reported. Six studies concerning HT and RT in patients with recurrent breast cancer were excluded from this review because no temperature data were reported. In the studies that were included, the reported thermal dose parameters were often not tested for a relationship with outcome (four studies) or the result of the tests were not reported ().
In total, the values of 27 different thermal dose parameters were presented and 32 parameters were tested for relationships. These parameters ranged from simple temperature statistics (minimum, average and maximum temperature, etc.) to more complex thermal dose parameters (CEM43, minutes >42.5 °C, % temperature >45 °C, etc.). Besides that, there is also an institutional variation in the calculation of thermal dose parameters. In the rare studies where thermal dose parameters were reported, the reported parameters varied widely, for example, low-end versus high-end temperatures. Although some correlation is expected because thermal dose parameters from the high-end, mean and low-end temperatures are correlated [Citation27, van der Zee, Personal communications 2019]; comparison of these parameters was nevertheless difficult.
The measured temperature during HT is vastly dependent on the type, extent and location of thermometry used; for example, the number of sensors, type of thermometry equipment [Citation52], location of sensors (e.g., invasive, skin, macroscopic tumor, scar, and healthy tissue) [Citation48], but also on the use of a (circulating) water bolus during HT treatment [Citation50]. For example, it is essential to have a sufficient number of sensors, because sampling the true temperature distribution during HT treatment with a small number of temperature sensors may introduce significant errors in the measured and calculated thermal dose variables compared to the true underlying temperature distribution [Citation53–57]. Several studies attribute their inability to find a dose-effect relationship to the low number of temperature sensors [Citation43,Citation49,Citation58]. Thus, adequate thermometry feedback during treatment and registration of thermal dose parameters are essential to ensure treatment quality. To allow comparison of the achieved temperature and thermal dose between different institutes, an uniform registration, calculation and reporting of thermal dose parameters is required.
Abovementioned arguments were a leading factor in scoring a moderate to high risk of bias in one of the domains of the QUIPS tool; i.e. the prognostic factor measurement. Only three out of the 22 included studies had a low risk of bias in this respective domain ().
Relationship of thermal dose parameters with outcome
An association between treatment outcome and thermal dose parameters was not found in all studies. This is not surprising, since most of the included studies were not designed to investigate the presence of a HT dose-response effect. Studies aimed at giving a uniform thermal dose which, if successful, will result in little variation in HT parameters, and thus a relationship of a HT parameter with outcome is more difficult to prove. Nevertheless, most included studies (22/25) showed enough variation in several of the thermal dose parameters to find significant positive relationships with outcome in both univariate and multivariate analysis. Three studies [Citation43,Citation44,Citation59] did not find any relationship between thermal dose parameters and CR, LC, and OS. Both studies of Oldenborg et al. [Citation44,Citation59] only investigated parameters derived from the skin, while Li et al. [Citation43] used a low number of invasive temperature sensors. Furthermore, univariate analysis does not take into account other patient-, tumor-, and treatment related parameters, which also contribute to the final outcome of RT + HT. In this systematic review, only ten studies have performed multivariate analysis to adjust for patient- tumor-, and treatment related parameters [Citation15,Citation27,Citation31,Citation35–37,Citation39–42,Citation48]. Of these studies, in seven of the 37 multivariate analyses performed for CR, LC and OS, the addition of a thermal dose parameter improved the model. To clarify the need for multivariate analysis, for example Hehr et al. [Citation46] found that more HT treatments yielded better duration of LC, but those patients probably received more RT treatments and thus a higher RT dose (range 30–68 Gy). The RT dose is known to influence treatment outcome in cancer patients. Patients with primary breast cancer treated with a high RT dose benefit less from the addition of HT than patients treated with a lower dose palliative RT scheme [Citation4,Citation16]. Performing a multivariate analysis to investigate the relationship of outcome with thermal dose parameters is thus essential to establish a reliable correlation. Not performing a multivariate analysis yields a high risk of bias in this situation.
Several studies find a relationship between the number of HT treatments and duration of LC. However, two randomized studies investigating the number of HT treatments found no difference between groups. In a prospective randomized study, comparing once versus twice weekly HT applications in 127 patients with chest wall recurrences of breast cancer, no difference was found in CR and duration of LC [Citation60] (patients also reported in [Citation33]). Similarly, Kapp et al. [Citation61] compared two versus six HT treatments in 70 patients with superficially located tumors and found no difference in CR and duration of LC. For both studies, the RT dose was similar between groups.
In general, the addition of HT to RT is well tolerated and adds no significant late toxicity in patients treated with RT + HT, although more blistering occurs during RT + HT compared to RT alone [Citation4]. This acute thermal toxicity is caused by higher maximum temperatures [Citation33,Citation36,Citation39–41,Citation48,Citation49] and thermal dose [Citation41,Citation48] on the skin during HT treatment. In untreated healthy skin, a higher thermal dose on the skin also increases blistering [Citation62,Citation63].
As shown in , thermal dose variables derived superficially were predictive for thermal toxicity, while thermal dose variables derived from invasive temperature sensors were predictive for both CR, LC, OS, and thermal toxicity. Surface temperature measurements during HT reflect an average of the water bolus and the true surface temperature. Nevertheless, the true surface temperature might indeed have a significant relationship with outcome. Therefore, it is important to measure both invasive and superficial temperatures during treatment and to report them separately.
Other HT parameters that are related to outcome are the SAR coverage [Citation37], type of HT device [Citation37,Citation42] and frequency [Citation36,Citation42]. These HT parameters influence the achieved temperature and thermal dose during HT.
Relationship between thermal dose parameters and outcome in other tumor types
Relationships between thermal dose parameters and clinical outcome have not only been found in recurrent breast cancer, but also in cervical carcinomas [Citation21,Citation22,Citation64], sarcomas [Citation56,Citation65], rectal cancer [Citation66], brain tumors [Citation67] and melanomas [Citation2,Citation68]. Thermal dose variables (maximum CEM43, CEM43T50, CEM43T90, CEM43T100, and TRISE) [Citation2,Citation21,Citation22,Citation56,Citation64,Citation65], minimum temperature variables (minimum temperature, T90) [Citation65,Citation67,Citation69] and maximum temperature variables [Citation2] were found to relate to outcome.
The first randomized controlled study showing a relationship between thermal dose achieved during HT and outcome was published in 1984 [Citation70]. Dewhirst and Sim randomized 236 dogs and cats with a variety of cancers to either receive RT alone or RT + HT. In the RT + HT group (n = 116) a relationship between CEM43T100 and both CR and duration of LC was found, while the maximum CEM43 was related to thermal injury. Animals achieving an average CEM43T100 ≤ 1 during all HT treatments had a CR comparable to the group treated with RT alone (n = 120). Furthermore, extensive temperature data from animal studies indicated that the coolest part of the tumor determined the response to RT + HT [Citation70–72]. Interestingly, in animals with tumors that showed very heterogeneous heating more skin toxicity was found while the response rate decreased [Citation72,Citation73]. It is likely that the maximum temperature limited the HT operator to increase the temperature in the coolest part of the tumor. These pioneering studies paved the way for subsequent human trials.
CEM43
The suitability of CEM43 to represent thermal dose is a widely debated subject, as excellently summarized by van Rhoon in 2016 [Citation17]. CEM43 is based on the direct cytotoxic effect of heat. The amount of cell death depends on the temperature and duration of heating and has been shown to be suitable for predicting the risk of adverse events of RT + HT in normal tissue [Citation48,Citation63]. CEM43 does not include all underlying synergistic mechanisms of HT to RT when used to predict treatment efficacy of combined RT + HT; for example, inhibiting DNA damage repair [Citation74], selective killing of radioresistant hypoxic tumor cells [Citation75] and increased radiosensitivity by enhanced tissue perfusion and reoxygenation [Citation76]. Each of these mechanisms display a different dose–effect relationship [Citation10]. Furthermore, instead of representing the direct effect of HT quality, the measured CEM43T90 might instead actually represent tumor characteristics (i.e., perfusion level), which are predictive of sensitivity to RT. The ability to realize a uniform and high temperature is likely to be associated with a homogeneously perfused and thus well-oxygenated tumor. Such a tumor would generally respond well to treatment, even with RT alone. Therefore, the development of a new dose parameter incorporating the combined RT + HT effect and/or employing thermoradiotherapy planning [Citation77] might more accurately represent the synergistic effect of RT and HT in the whole area treated with RT + HT.
Nevertheless, most underlying HT mechanisms display increasing HT effectiveness with higher temperatures. This might explain why multiple clinical studies included in this review did find a relationship between CR and CEM43 or other thermal dose parameters (). Consequently, CEM43T90 has been deployed in a prospective setting to investigate whether patients with superficial tumors treated with CEM43T90 ≤ 1 or CEM43T90 > 10 had different outcome. Indeed, superficial tumors treated with a thermal dose CEM43T90 > 10 had considerably longer duration of LC than patients with CEM43T90 ≤ 1 [Citation16]. So although the CEM43 concept has shortcomings, at present this parameter does show a relationship with outcome in many studies.
Conclusion
This systematic review shows that higher temperature and thermal dose during hyperthermia therapy significantly improve clinical outcome; complete response, local control, and overall survival; and increase thermal toxicity for patients with recurrent breast cancer treated with radiotherapy and hyperthermia therapy. A sufficiently high hyperthermia therapy dose is required to achieve the radiosensitizing effect of hyperthermia therapy. Thermal dose parameters derived from the surface have a relationship with thermal toxicity, whereas invasive thermal dose parameters have a relationship with response, local control and overall survival as well as with thermal toxicity. Achieving a clinically relevant effect of hyperthermia therapy when added to radiotherapy for patients with locoregional recurrent breast cancer in previously irradiated area, requires ensuring a high hyperthermia dose.
Supplemental Material
Download PDF (619.5 KB)Acknowledgments
This research did not receive any specific grant from funding agencies in the public, commercial, or not-for-profit sectors.
Disclosure statement
The authors report no conflict of interest.
References
- Franckena M, van der Zee J. Use of combined radiation and hyperthermia for gynecological cancer. Curr Opin Obstet Gynecol. 2010;22(1):9–14.
- Overgaard J, Gonzalez Gonzalez D, Hulshof MC, et al. Hyperthermia as an adjuvant to radiation therapy of recurrent or metastatic malignant melanoma. A multicentre randomized trial by the European Society for Hyperthermic Oncology. Int J Hyperth. 1996;12(1):3–20.
- van der Zee J, González González D, van Rhoon GC, et al. Comparison of radiotherapy alone with radiotherapy plus hyperthermia in locally advanced pelvic tumours: a prospective, randomised, multicentre trial. Dutch Deep Hyperthermia Group. Lancet. 2000;355(9210):1119–1125.
- Vernon CC, Hand JW, Field SB, et al. Radiotherapy with or without hyperthermia in the treatment of superficial localized breast cancer: Results from five randomized controlled trials. International Collaborative Hyperthermia Group. Int J Radiat Oncol Biol Phys. 1996;35:731–744.
- Issels RD, Lindner LH, Verweij J, et al. Neo-adjuvant chemotherapy alone or with regional hyperthermia for localised high-risk soft-tissue sarcoma: a randomised phase 3 multicentre study. Lancet Oncol. 2010;11(6):561–570.
- Datta NR, Puric E, Klingbiel D, et al. Hyperthermia and radiation therapy in locoregional recurrent breast cancers: a systematic review and meta-analysis. Int J Radiat Oncol Biol Phys. 2016;94(5):1073–1087.
- Perez CAA, Pajak T, Emami B, et al. Randomized phase III study comparing irradiation and hyperthermia with irradiation alone in superficial measurable tumors. Final report by the Radiation Therapy Oncology Group. Am J Clin Oncol. 1991;14(2):133–141.
- Engin K, Tupchong L, Waterman FM, et al. Multiple field hyperthermia combined with radiotherapy in advanced carcinoma of the breast. Int J Hyperth. 1994;10(5):587–603.
- Stauffer PR. Evolving technology for thermal therapy of cancer. Int J Hyperth. 2005;21(8):731–744.
- Crezee H, Van Leeuwen CM, Oei AL, et al. Thermoradiotherapy planning: integration in routine clinical practice. Int J Hyperth. 2016;32(1):41–49.
- Overgaard J. Simultaneous and sequential hyperthermia and radiation treatment of an experimental tumor and its surrounding normal tissue in vivo. Int J Radiat Oncol Biol Phys. 1980;6(11):1507–1517.
- Westra A, Dewey WC. Variation in sensitivity to heat shock during the cell-cycle of Chinese hamster cells in vitro. Int J Radiat Oncol Biol Phys. 1971;19:467–477.
- George KC, Streffer C, Pelzer T. Combined effects of X rays, Ro 03-8799, and hyperthermia on growth, necrosis, and cell proliferation in a mouse tumor. Int J Radiat Oncol Biol Phys. 1989;16(4):1119–1122.
- Field SB, Morris CC. The relationship between heating time and temperature: its relevance to clinical hyperthermia. Radiother Oncol. 1983;1(2):179–186.
- Sherar M, Liu FF, Pintilie M, et al. Relationship between thermal dose and outcome in thermoradiotherapy treatments for superficial recurrences of breast cancer: data from a phase III trial. Int J Radiat Oncol Biol Phys. 1997;39(2):371–380.
- Jones EL, Oleson JR, Prosnitz LR, et al. Randomized trial of hyperthermia and radiation for superficial tumors. J Clin Oncol. 2005;23(13):3079–3085.
- Van Rhoon GC. Is CEM43 still a relevant thermal dose parameter for hyperthermia treatment monitoring? Int J Hyperth. 2016;32(1):50–62.
- Nielsen OS, Overgaard J. Importance of preheating temperature and time for the induction of thermotolerance in a solid tumour in vivo. Br J Cancer. 1982;46(6):894–903.
- Dewhirst MW, Viglianti BL, Lora-Michiels M, et al. Basic principles of thermal dosimetry and thermal thresholds for tissue damage from hyperthermia. Int J Hyperth. 2003;19(3):267–294.
- Sapareto SA, Dewey WC. Thermal dose determination in cancer therapy. Int J Radiat Oncol Biol Phys. 1984;10(6):787–800.
- Kroesen M, Mulder HT, van Holthe JML, et al. Confirmation of thermal dose as a predictor of local control in cervical carcinoma patients treated with state-of-the-art radiation therapy and hyperthermia. Radiother Oncol. 2019;140:150–158.
- Franckena M, Fatehi D, de Bruijne M, et al. Hyperthermia dose-effect relationship in 420 patients with cervical cancer treated with combined radiotherapy and hyperthermia. Eur J Cancer. 2009;45(11):1969–1978.
- Leopold KA, Dewhirst M, Samulski T, et al. Relationships among tumor temperature, treatment time, and histopathological outcome using preoperative hyperthermia with radiation in soft tissue sarcomas. Int J Radiat Oncol Biol Phys. 1992;22(5):989–998.
- Moher D, Liberati A, Tetzlaff J, et al. Preferred reporting items for systematic reviews and meta-analyses: the PRISMA statement. PLoS Med. 2009;6(7):e1000097.
- Agresti A, Categorical data analysis. In: Balding DJ, Bloomfield P, Cressie NAC, et al., editors. Categorical data analysis. 2nd ed. Hoboken, (NJ): Wiley Interscience; 2002. p. 54.
- Hayden JA, Van Der Windt DA, Cartwright JL, et al. Assessing bias in studies of prognostic factors. Ann Intern Med. 2013;158(4):280–286.
- Hand JW, Machin D, Vernon CC, et al. Analysis of thermal parameters obtained during phase III trials of hyperthermia as an adjunct to radiotherapy in the treatment of breast carcinoma. Int J Hyperth. 1997;13(4):343–364.
- Dragovic J, Seydel HG, Sandhu T, et al. Local superficial hyperthermia in combination with low-dose radiation therapy for palliation of locally recurrent breast carcinoma. J Clin Oncol. 1989;7(1):30–35.
- Gonzalez Gonzalez D, van Dijk JD, Blank LE. Chestwall recurrences of breast cancer: results of combined treatment with radiation and hyperthermia. Radiother Oncol. 1988;12(2):95–103.
- Phromratanapongse P, Steeves RA, Severson SB, et al. Hyperthermia and irradiation for locally recurrent previously irradiated breast cancer. Strahlentherapie Und Onkol. 1991;167:93–97.
- Refaat T, Sachdev S, Sathiaseelan V, et al. Hyperthermia and radiation therapy for locally advanced or recurrent breast cancer. Breast. 2015;24(4):418–425.
- Gabriele P, Ferrara T, Baiotto B, et al. Radio hyperthermia for re-treatment of superficial tumours. Int J Hyperth. 2009;25(3):189–198.
- Seegenschmiedt HM, Karlsson UL, Sauer R, et al. Superficial chest wall recurrences of breast cancer: prognostic treatment factors for combined radiation therapy and hyperthermia. Radiology. 1989;173(2):551–558.
- Sannazzari GL, Gabriele P, Orecchia R, et al. Results of hyperthermia, alone or combined with irradiation, in chest wall recurrences of breast cancer. Tumori 1989;75(3):284–288.
- Kapp DS, Barnett TA, Cox RS, et al. Hyperthermia and radiation therapy of local-regional recurrent breast cancer: prognostic factors for response and local control of diffuse or nodular tumors. Int J Radiat Oncol Biol Phys. 1991;20(5):1147–1164.
- Kapp DS, Cox RS, Barnett TA, et al. Thermoradiotherapy for residual microscopic cancer: elective or post-excisional hyperthermia and radiation therapy in the management of local–regional recurrent breast cancer. Int J Radiat Oncol Biol Phys. 1992;24(2):261–277.
- Lee HK, Antell AG, Perez CA, et al. Superficial hyperthermia and irradiation for recurrent breast carcinoma of the chest wall: prognostic factors in 196 tumors. Int J Radiat Oncol Biol Phys. 1998;40(2):365–375.
- Arcangeli G, Casale C, Colistro F, et al. One versus four heat treatments in combination with radiotherapy in metastatic mammary carcinoma. Int J Radiat Oncol Biol Phys. 1991;21(6):1569–1574.
- Lindholm C-E, Kjellen E, Nilsson P, et al. Prognostic factors for tumour response and skin damage to combined radiotherapy and hyperthermia in superficial recurrent breast carcinomas. Int J Hyperth. 1995;11(3):337–355.
- Linthorst M, Baaijens M, Wiggenraad R, et al. Local control rate after the combination of re-irradiation and hyperthermia for irresectable recurrent breast cancer: results in 248 patients. Radiother Oncol. 2015;117(2):217–222.
- Linthorst M, van Geel AN, Baaijens M, et al. Re-irradiation and hyperthermia after surgery for recurrent breast cancer. Radiother Oncol. 2013;109(2):188–193.
- Van Der Zee J, Van Der Holt B, Rietveld PJM, et al. Reirradiation combined with hyperthermia in recurrent breast cancer results in a worthwhile local palliation. Br J Cancer. 1999;79:483–490.
- Li G, Mitsumori M, Ogura M, et al. Local hyperthermia combined with external irradiation for regional recurrent breast carcinoma. Int J Clin Oncol. 2004;9(3):179–183.
- Oldenborg S, Griesdoorn V, Van Os R, et al. Reirradiation and hyperthermia for irresectable locoregional recurrent breast cancer in previously irradiated area: size matters. Radiother Oncol. 2015;117(2):223–228.
- Ben-Yosef R, Kapp DS, B-Y R. Persistent and/or late complications of combined radiation therapy and hyperthermia. Int J Hyperth. 1992;8(6):733–745.
- Hehr T, Lamprecht U, Glocker S, et al. Thermoradiotherapy for locally recurrent breast cancer with skin involvement. Int J Hyperth. 2001;17(4):291–301.
- Lee ER, Kapp DS, Lohrbach AW, et al. Influence of water bolus temperature on measured skin surface and intradermal temperatures. Int J Hyperth. 1994;10(1):59–72.
- Bakker A, Kolff MW, Holman R, et al. Thermal skin damage during reirradiation and hyperthermia is time-temperature dependent. Int J Radiat Oncol Biol Phys. 2017;98(2):392–399.
- Engin K, Tupchong L, Waterman FM, et al. Patchwork fields in thermoradiotherapy for extensive chest wall recurrences of breast carcinoma. Breast Cancer Res Tr. 1993;27(3):263–270.
- Lindholm C, Kjellen E, Nilsson P, et al. Microwave-induced hyperthermia and radiotherapy in human superficial tumours: clinical results with a comparative study of combined treatment versus radiotherapy alone. Int J Hyperth. 1987;3(5):393–411.
- Dobšíček Trefná H, Crezee H, Schmidt M, et al. Quality assurance guidelines for superficial hyperthermia clinical trials: I. Clinical requirements. Int J Hyperth. 2017;33(4):471–482.
- Schooneveldt G, Bakker A, Balidemaj E, et al. Thermal dosimetry for bladder hyperthermia treatment. An overview. Int J Hyperth. 2016;32(4):417–433.
- Bakker A, Holman R, Rodrigues D, et al. Analysis of the required number of sensors for adequate monitoring of skin temperature distribution during superficial microwave hyperthermia treatment. Strahlentherapie Und Onkol. 2018;194:485–486.
- Cox RS, Kapp DS. Correlation of thermal parameters with outcome in combined radiation therapy-hyperthermia trials. Int J Hyperth. 1992;8(6):719–732.
- Edelstein-Keshet L, Dewhirst MW, Oleson JR, et al. Characterization of tumour temperature distributions in hyperthermia based on assumed mathematical forms. Int J Hyperth. 1989;5(6):757–777.
- Oleson JR, Samulski TV, Leopold KA, et al. Sensitivity of hyperthermia trial outcomes to temperature and time: implications for thermal goals of treatment. Int J Radiat Oncol Biol Phys. 1993;25(2):289–297.
- Corry PM, Jabboury K, Kong JS, et al. Evaluation of equipment for hyperthermic treatment of cancer. Int J Hyperth. 1988;4(1):53–74.
- Perez CA, Gillespie B, Pajak T, et al. Quality assurance problems in clinical hyperthermia and their impact on therapeutic outcome: a report by the Radiation Therapy Oncology Group. Int J Radiat Oncol Biol Phys. 1989;16(3):551–558.
- Oldenborg S, Van Os RM, Van Rij CM, et al. Elective re-irradiation and hyperthermia following resection of persistent locoregional recurrent breast cancer: a retrospective study. Int J Hyperth. 2010;26(2):136–144.
- Keilholz L, Seegenschmiedt MH, Martus P, et al. Prospective randomized study to compare once versus twice weekly hyperthermia applications and conventional radiotherapy for chestwall recurrences of breast cancer: 10 year results. Proceedings of the 39th Annual ASTRO Meeting; 1997 Oct 19–23; Orlando (FL); 1997. p. 265.
- Kapp DS, Petersen IA, Cox RS, et al. Two or six hyperthermia treatments as an adjunct to radiation therapy yield similar tumor responses: results of a randomized trial. Int J Radiat Oncol Biol Phys. 1990;19(6):1481–1495.
- Greenhalgh DG, Lawless MB, Chew BB, et al. Temperature threshold for burn injury: an oximeter safety study. J Burn Care Rehabil. 2004;25(5):411–415.
- Yarmolenko PS, Moon EJ, Landon C, et al. Thresholds for thermal damage to normal tissues: an update. Int J Hyperth. 2011;27(4):320–343.
- Ohguri T, Harima Y, Imada H, et al. Relationships between thermal dose parameters and the efficacy of definitive chemoradiotherapy plus regional hyperthermia in the treatment of locally advanced cervical cancer: data from a multicentre randomised clinical trial. Int J Hyperth. 2018;34(4):461–468.
- Issels RD, Mittermüller J, Gerl A, et al. Improvement of local control by regional hyperthermia combined with systemic chemotherapy (ifosfamide plus etoposide) in advanced sarcomas: updated report on 65 patients. J Cancer Res Clin Oncol. 1991;117(S4):S141–S7.
- Wust P, Rau B, Gellerman J, et al. Radiochemotherapy and hyperthermia in the treatment of rectal cancer. Recent Results Cancer Res. 1998;146:175–191.
- Sneed P, Stauffer P, Gutin P, et al. Interstitial irradiation and hyperthermia for the treatment of recurrent malignant brain tumors. Neurosurgery. 1991;28(2):206–215.
- Emami B, Perez CA, Konefal J, et al. Thermoradiotherapy of malignant melanoma. Int J Hyperth. 1988;4(4):373–381.
- Seegenschmiedt MH, Martus P, Fietkau R, et al. Multivariate analysis of prognostic parameters using interstitial thermoradiotherapy (IHT-IRT): tumor and treatment variables predict outcome. Int J Radiat Oncol Biol Phys. 1994;29(5):1049–1063.
- Dewhirst MW, Sim DA. The utility of thermal dose as a predictor of tumor and normal tissue responses to combined radiation and hyperthermia. Cancer Res. 1984;44:4772–4780.
- Dewhirst MW, Connor WG, Sim DA, et al. Importance of minimum tumor temperature in determining early and long-term responses of spontaneous canine and feline tumors to heat and radiation. Cancer Res. 1984;44(1):43–50.
- Dewhirst MW, Sim DA. Estimation of therapeutic gain in clinical trials involving hyperthermia and radiotherapy? Int J Hyperth. 1986;2(2):165–178.
- Denman DL, Legorreta RA, Kier AB, et al. Therapeutic responses of spontaneous canine malignancies to combinations of radiotherapy and hyperthermia. Int J Radiat Oncol Biol Phys. 1991;21(2):415–422.
- Oei AL, Vriend LEM, Crezee J, et al. Effects of hyperthermia on DNA repair pathways: one treatment to inhibit them all. Radiat Oncol. 2015;10(1):1–13.
- Nielsen OS. Effect of fractionated hyperthermia on hypoxic cells in vitro. Int J Radiat Biol. 1981;39:73–82.
- Dewhirst MW, Vujaskovic Z, Jones E, et al. Re-setting the biologic rationale for thermal therapy. Int J Hyperth. 2005;21(8):779–790.
- Kok HP, Crezee J, Franken NAP, et al. Quantifying the combined effect of radiation therapy and hyperthermia in terms of equivalent dose distributions. Int J Radiat Oncol Biol Phys. 2014;88(3):739–745.