Abstract
Purpose: This study assessed the efficacy and safety of computed tomography (CT)-guided percutaneous thermal ablation for hepatocellular carcinoma adjacent to the second porta hepatis.
Methods: From January 2011 to June 2018, 59 consecutive patients (51 men and 8 women aged 29–85 years) with 65 tumors (mean maximum tumor diameter: 20.5 ± 1.2 mm; range: 5–50 cm) adjacent to the second porta hepatis underwent percutaneous CT-guided thermal ablation. The complete ablation rate, local progression-free survival (LPFS), progression-free survival (PFS), prognostic factors for local progression, and safety of this technique were assessed.
Results: No more than three sessions were performed to complete a patient’s treatment. Technical success was achieved for all tumors. In the median follow-up of 18.5 months, local tumor progression occurred in 32.2% (19/59) of patients, while tumor progression occurred in 71.2% (42/59). The median LPFS was 37.4 months. The 1-, 2-, and 3-year LPFS rates were 86.2%, 66.5%, and 66.5%, respectively. The median PFS was 11.0 months. The 1-, 2-, and 3-year PFS rates were 47.9%, 27.0%, and 20.2%, respectively. The tumor size (P = .007) and distance between the tumor and inferior vena cava (P = 0.018) were the independent prognostic factors for local tumor progression.
Conclusion: We showed that CT-guided percutaneous microwave ablation and radiofrequency ablation are effective and safe treatments for tumors adjacent to the second porta hepatis, particularly in patients with lesions less than 3 cm and a distance between the tumor and inferior vena cava of more than 5 mm.
Introduction
Hepatocellular carcinoma (HCC) was the seventh most common malignancy and the fourth leading cause of cancer mortality worldwide in 2018; it accounts for 841,000 new cases and 782,000 deaths annually [Citation1]. Surgical resection is widely accepted as a curative treatment for HCC patients with solitary lesions and well-preserved liver function [Citation2]. However, resection of small tumors in unfavorable sites will sacrifice a large amount of functional liver parenchyma, thus increasing the complication risk and mortality rate [Citation3,Citation4]. Local ablative techniques, such as radiofrequency ablation (RFA) and microwave ablation (MWA), have emerged as viable alternatives for the treatment of HCC with satisfactory results. The European Association for the Study of the Liver considers radiofrequency ablation the standard treatment for patients with HCC at Barcelona Clinic Liver Cancer stage 0–A who are not eligible for surgery. Radiofrequency ablation is also recommended for patients with larger tumors (≥3 cm), multiple tumors (up to three), and advanced liver disease (Child-Pugh score B) [Citation5–8]. Furthermore, RFA is relatively safe, with a major complication rate of 2.2% [Citation9].
HCC adjacent to the second porta hepatis, which is anatomically defined as where three hepatic veins enter into the inferior vena cava immediately after leaving the liver, is a relative contraindication for resection [Citation10–12].With regard to thermal ablation for perivascular HCC, the heat-sink effect contributes to incomplete necrosis and a higher recurrence rate [Citation13–16]. It has been reported that surgery provided better long-term tumor control and overall survival in cases of perivascular HCCs than RFA [Citation17]. Moreover, the heat-sink effect typically occurs when ablation is performed in this particular location, as the tumor is enclosed by more than one large vessel, including hepatic veins and the inferior vena cava.
Previous studies have examined the effects of tumor ablation on adjacent vessels, including the artery, hepatic vein, and porta vein. However, to date, no study has reported the effects of percutaneous thermal ablation on tumors adjacent to the second porta hepatis. In this study, we aimed to retrospectively analyze the efficacy and safety of computed tomography (CT)-guided percutaneous thermal ablation as a treatment for HCC adjacent to the second porta hepatis as well as the prognostic factors that may influence the outcomes.
Materials and methods
Patients
This retrospective analysis was approved by the Sun Yat-sen University Cancer Center institutional ethics committee. Written informed consent was obtained from each patient before treatment. All patients were informed of the therapeutic indication, therapeutic effect, and possible complications. Between January 2011 and June 2018, a total of 3,277 consecutive patients with primary and secondary liver tumors underwent CT-guided MWA (n = 2,056) or RFA (n =1,251). These patients included those who refused or were considered unsuitable for surgical resection.
HCC adjacent to the second porta hepatis was defined as index tumors located in the second porta hepatis within 2 cm from the inferior vena cava and 5 mm from the hepatic vein based on image examination. Patients with (a) an HCC adjacent to the second porta hepatis, (b) a tumor size ≤5 cm, (c) no more than three tumors with a maximum diameter of 3 cm, (d) Child-Pugh class A or B liver disease, (e) no vascular tumor invasion or extrahepatic metastasis, (f) an Eastern Cooperative Oncology Group performance status score of ≤2, (g) a platelet count of >50 × 109/L, and (h) a prothrombin time ratio ≥70% were included in the study. From January 2011through June 2018, 3,277 patients with hepatic carcinoma or hepatic metastases underwent CT-guided percutaneous MWA or RFA in our department. Fifty-nine patients (51 men and 8 women; mean age: 57.7 ± 11.7 years; range: 29–85 years) with 65 tumors who underwent CT-guided MWA or RFA for HCC adjacent to the second porta hepatis in an outpatient setting and who fulfilled the inclusion criteria were enrolled in this study. For patients who had three tumors or a tumor larger than 3 cm, a combination treatment was used: transcatheter arterial chemoembolization (TACE) was performed before thermal ablation (n =20). A flowchart of the patient selection process is shown in . The baseline patient and tumor characteristics are summarized in .
Table 1. Baseline characteristics of patients and tumor.
Preparation
All patients underwent contrast-enhanced CT or magnetic resonance imaging (MRI) 2 weeks before surgery for a baseline imaging evaluation of the HCC. All of them underwent routine laboratory examinations, including complete blood count, blood chemistry, coagulation function, and viral titers (such as hepatitis B virus, hepatitis C virus, and human immunodeficiency virus).
Percutaneous ablation procedure
CT scans were performed to locate the liver tumors (PHILIPS 16-slice spiral CT, the Netherlands). If necessary, contrast-enhanced CT was used to detect the tumor from adjacent vessels. The type of ablation method (MWA or RFA) used was dependent on the tumor size, tumor location, or operator’s preference. The puncture routine was discussed and preoperatively decided to avoid vessel injuries and determine the patient’s body position during the procedure. The entire procedure was conducted under intravenous anesthesia (Propofol 1.5 ∼ 4.5 mg/kg continuous intravenous infusion per hour; AstraZeneca S.p.A., Italy) administered by an experienced anesthetist, and real-time electrocardiography monitoring was performed. Patients maintained spontaneous breathing with oxygen inhalation via a nasal tube (2–6 L/min). Routine disinfection was performed, and local anesthesia was administered around the selected puncture points. The applicator was inserted in a stepwise manner. Commercially available internally cooled RFA (STARmed, Gyeonggi-do, South Korea) and MWA (Vision Medical Institute, Nanjing, China) systems were used. For RFA, the output power was set according to the manufacturer’s recommended protocol; a multistep incremental increase in power was used as an algorithm for energy deposition. It was initiated at 120 W and was gradually increased by 20 W/min to 200 W. Then, it was automatically adjusted to the real-time resistance of the tissue to prevent tissue dehydration and loss of heat conduction. For MWA, the power was generally set at 50–70 W. The accumulated ablation time ranged from 5 to 20 min. For tumors larger than 3 cm, multiple-site ablations were performed or two applicators were inserted simultaneously. The objective of ablation was to obtain at least a 0.5-cm margin of normal hepatic tissue surrounding the tumor. After the ablation, the antenna path was cauterized to avoid bleeding and tumor seeding. All procedures were completed by one interventional radiologist (W.J.F) with more than 10 years of experience in tumor ablation ().
Figure 2. Contrast-enhanced magnetic resonance imaging (MRI) of a 58-year-old male patient diagnosed with a single HCC lesion (3.7 cm × 3.2 cm) adjacent to the second porta hepatis (arrow). The mass showed markedly high signal T2-weighted images (a) and low signal on transverse and coronal MRI in the hepatobiliary phase (b,c). (d) He underwent CT-guided multiple-site MWA in the supine position with the output of 70 W for 20 min in total. A month after the ablation, MRI results (e,f) showed that the lesion was completely necrotic without any enhancement.
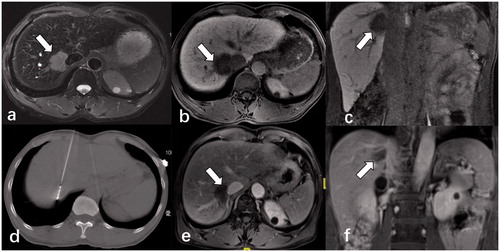
Chest and abdominal CT scans were performed immediately after the treatment to evaluate the necrotic conditions after ablation and any complications. Routine blood tests, biochemical tests, coagulation function tests, and chest X-rays (if the puncture routine went through lung tissue) were performed the day after ablation treatment.
Evaluation of treatment efficacy and complications
Patients underwent abdominal contrast-enhanced CT or MRI examinations 4–6 weeks after the ablation. The images were separately reviewed by two radiologists for diagnosis of residual tumor. Technical success was defined as no focal nodular enhancement around the target tumor ablation zone. Incomplete ablation was defined as the presence of arterial contrast enhancement and portal venous washout within the ablation zone suggestive of residual tumor 1 month after ablation. Local tumor progression was defined as an irregular peripheral enhancement in the original sites, which were previously considered to be completely ablated during the follow-up period. New tumor recurrence was defined as a lesion at least 2 cm away from the original tumor. Local tumor progression and new tumor recurrence were both considered tumor progression. Progression-free survival (PFS) was defined as the time from complete ablation until the date that local or new tumor recurrence was diagnosed with radiology. Local PFS (LPFS) was defined as the time from complete ablation until the date of local tumor recurrence. If complete tumor ablation was achieved, imaging follow-up was performed every 3 months. When the tumor was incompletely ablated, recurred, or a new tumor emerged, a repeat MWA or RFA was performed if the tumors fulfilled the original requirements. Molecular targeted therapy is also recommended in those cases. Complications were assessed based on the Society of Interventional Radiology (SIR) classification system and by standardization of terminology and reporting criteria on image-guided tumor ablation [Citation18,Citation19]. Major complications were defined as events that resulted in substantial morbidity and disability, which led to the need for additional invasive therapy and/or substantially lengthened hospitalization (SIR classifications C–E). All other complications were considered minor (SIR classifications A–B).
Statistical analyses
All statistical analyses were performed using SPSS software (version 24; SPSS, Chicago, IL). LPFS and PFS were calculated using the Kaplan–Meier method. Univariate and multivariate analyses were performed to determine significant factors associated with LPFS. Univariate analyses were performed using a log-rank test. Variables with a P-value of less than .10 in the univariate analysis were entered into the multivariate analysis, which was performed using a Cox proportional hazard regression model. P-values less than 0.05 were considered significant.
Results
During the follow-up of 18.5 months (range: 3.1–49.2 months), all 65 tumors from 59 patients were completely ablated. About 49 (75.4%) tumors appeared completely necrotic after the first ablation treatment. Approximately 13 (20%) tumors required a second ablation, and 3 (4.6%) required a third ablation. A total of 84 ablation procedures were performed in this study. The mean number of ablation sessions was 1.29 ± 0.55. The mean duration of ablation procedures was 11.5 ± 3.89 min (MWA, 10.6 ± 3.80 min vs. RFA, 12.91 ± 3.66 min; p < 0.05).
Local tumor progression was observed in 19 (32.2%) of 59 patients. Approximately 21% (4/19) of the tumors occurred in the nonperivascular site, while 79% (15/19) occurred in the perivascular site. Tumor progression was observed in 41 (69.5%) of the 59 patients. Five patients received molecular targeted therapy (Sorafenib) after tumor progression. The median LPFS was 37.4 months. The 1-, 2-, and 3-year LPFS rates were 86.2%, 66.5%, and 66.5%, respectively (. The median PFS was 11.0 months. The 1-, 2-, and 3-year PFS rates were 47.9%, 27.0%, and 20.2%, respectively (. Univariate analysis revealed that the maximum tumor size and Child-Pugh classification were significantly associated with better LPFS (P < .05). The Child-Pugh classification was not included in the multivariate analysis, as only a few cases (n =2) were classified as Child-Pugh B (). Tumor size and the distance between the tumor and the inferior vena cava (IVC) were included in the multivariate analysis (), which revealed that tumor size () and distance between the tumor and the IVC () were independent prognostic factors for LPFS (P < .05). Subgroup analysis of MWA and RFA showed that the mean tumor diameters were 2.1 ± 1.3 vs. 1.9 ± 1.2 cm (P = 0.542), respectively; the median LPFS was 37.1 vs. 42.3 months (P =0.17), respectively. Univariate and multivariate analyses revealed that LPFS did not differ significantly between MWA and RFA ().
Figure 3. (a) The median LPFS was 37.4 months. The 1-, 2-, and 3-year LPFS rates were 86.2%, 66.5%, and 66.5%, respectively. (b) The median PFS was 11.0 months. The 1-, 2-, and 3-year PFS rates were 47.9%, 27.0%, and 20.2%, respectively.
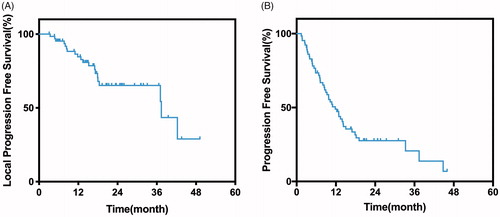
Figure 4. (a) Kaplan–Meier curves of LPFS in patients with tumor smaller than 3 cm (n =47; median LPFS: 42.3 months) or larger than 3 cm (n =18; median LPFS, 18.0 months; P =.007). (b) Kaplan–Meier curves of LPFS in patients with whose distance between tumor and IVC is larger than 5 mm (n =41; median LPFS: 42.3 months) or larger than 5 mm (n =24; median LPFS, 18.4 months; P =.018).
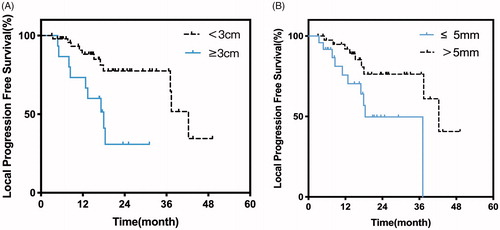
Table 2. Univariate analysis of the risk factors of local progression-free survival.
Table 3. Multivariate analysis of the risk factors of local progression-free survival.
Complications and side effects
No treatment-related deaths occurred in our study, and only one major complication was reported. The major complication rate was 1.2% (one case of tumor seeding), while the minor complication rate was 20.2% (17/84). Minor complications included mild hepatorrhagia (n =7), mild pulmonary bleeding (n =4), gallbladder hematocele (n =1), and self-limiting pneumothorax (n =5). Mild abdominal pain occurred in nine patients, and the pain was relieved after injection of opioids. High-grade fever (38 °C–38.9 °C) occurred in 11 patients, and 2 of them were administered antipyretic medication ().
Table 4. Incidence of complications related to ablation.
Discussion
Treatment for patients with tumors adjacent to the second porta hepatis was difficult. Traditional surgery involved the disadvantages of high morbidity and operation trauma. Recently, thermal ablation served with promising effect as a minimally invasive treatment for liver tumors. Several studies have reported it to be an effective alternative treatment for perivascular hepatic tumors. Kang et al. reported that the outcomes of RFA for small perivascular HCC were similar to those for nonperivascular HCC [Citation20]. However, the notable heat-sink effects that influenced our outcomes were also reported in previous studies [Citation14–16]. Due to concerns of limited efficacy and severe complications, the location of tumors near the second porta hepatis used to be regarded as a contraindication for ablation; until now, no research has been reported in this area. Results of the present study show that thermal ablation is a safe and feasible treatment for tumors adjacent to second porta hepatis.
In this study, we reported the LTP and LPFS of tumors adjacent to the second porta hepatis. The rate of local tumor progression (32.2%) was lower than that reported by Lu et al. (48%), which only focused on tumors that developed close to large vessels [Citation14]. However, the 1- and 3-year local tumor progression rates in our study were higher than those reported in the study by Huang et al. involving MWA (13.8% vs. 10.7% and 33.5% vs. 17.3%, respectively) [Citation15]. This difference can be explained by the fact that the second porta hepatis is a relatively unique location with more than one large vessel in the vicinity. With the influential heat-sink effect, residual disease may be more likely to appear.
In previous studies, tumor size and the nearness of a major vessel were considered to be the main risk factors for local tumor recurrence after ablation [Citation21,Citation22]. This widely accepted perspective was consistent with our finding that lesions less than 3 cm or a distance between tumor and the IVC of more than 5 mm were associated with better local tumor control, indicating that additional measures should be taken when treating tumors with these characteristics.
Generally, a circumferential safety margin of at least 0.5 cm covering the tumor is recommended for thermal ablation to avoid local recurrence [Citation23]. The heat-sink effect usually contributes to an inadequate ablation zone, including volume and shape, and makes it impractical to attain a safety margin for tumors within 5 mm of large vessels [Citation24–27]. In a previous study, the heat-sink effect usually occurred near vessels of 4 mm or larger [Citation13]. Similar to our findings, patients with tumors less than 5 mm from the IVC had a prolonged LPFS compared to those with tumors more than 5 mm from the IVC. In addition, the presence of vessels more than 3 mm in diameter adjacent to the tumor is an independent predictor of RFA treatment outcome [Citation14]. The hepatic vein and IVC are both large hepatic vessels. Therefore, in this unique location, we present some modified ablation strategies to overcome these problems while using caution to avoid vessel injury. First, for large perivascular tumors, a multipolar RFA system should be used rather than a monopolar system. Since monopolar modality is more susceptible to heat loss, a multipolar RFA device could achieve a larger ablation zone, even when large vessels are nearby [Citation28]. Compared with RFA, MWA allowed for higher and faster temperature peaks; it also appeared less sensitive to the heat-sink effect than monopolar RFA [Citation29]. Moreover, Brace et al. reported that perivascular tumors can be more effectively treated with multiple MWA antennas [Citation30]; therefore, MWA may be suitable for patients with perivascular tumors. However, no significant differences were observed between RFA and MWA in this study with LPFS of 42.2 months vs. 37.1 months (P = 0.17). This is probably due to a longer heating duration, and impedance monitoring during RFA may overcome the heat-sink effect to some extent. In addition, all of the tumors in our study were less than 5 cm, and MWA often displayed advantages of achieving higher temperature and larger ablation volume in the cases of relatively large tumors. Second, multiple-site ablation, also known as overlapping ablation, is recommended to avoid residual tumors; however, it would increase the risk for local recurrence because of tumor seeding as well as the risk of hemorrhage due to multiple displacements. Third, a temporary reduction in blood flow through arterial embolization, temporary vascular occlusion, or Pringle’s maneuver would efficiently reduce the heat-sink effect [Citation31–33]. However, extreme caution should be observed when performing the abovementioned methods, as they may lead to potential thrombosis and permanent occlusion. In the past, ethanol injection into the tumor has effectively enlarged the ablation zone without compromising the blood flow [Citation34]. Fourth, increased power output and prolonged ablation duration is recommended.
A previous retrospective study suggested that a combination of transcatheter arterial chemoembolization (TACE) and percutaneous RFA could improve local tumor control [Citation35]. However, in the present study, no significant difference was observed between the local recurrence rate of combination treatment and that of TACE alone. This finding can be explained by the fact that a majority of the tumors in our study were less than 3 cm in diameter (72.3%, 47/65). This result is in agreement with those of a previous study indicating that TACE combined with percutaneous RFA seemed ineffective against small HCCs, as most of them could achieve complete necrosis after ablation alone [Citation36].
Ren et al. reported that no deaths or complications occurred in patients who underwent MWA combined with percutaneous ethanol injection for treatment of liver tumors adjacent to the porta hepatis [Citation37]. Similarly, no treatment-related deaths occurred in our study. Only one major complication was reported: the patient had a tumor seeding at the abdominal wall and underwent resection. Although 17 cases of minor complications appeared in our study, none of the patients had obvious symptoms, and all recovered after observation. Thus, we may conclude that CT-guided ablation is a safe method for the treatment of tumors adjacent to the second porta hepatis.
All of the procedures in this study were performed under CT guidance. Although ultrasonography guidance has been proved effective and widely applied for ablation with the advantage of low expense and simplicity, CT-guided ablation makes it more practicable when a transpulmonary approach is required or when tumors in this unique location appear inconspicuous under ultrasonography guidance.
This study inevitablely had some limitations. The data were acquired from a single institution, and the sample size was small. Hence, a multicenter study with a larger sample size is warranted to confirm our findings. Additionally, some of the patients with multiple intrahepatic tumors underwent TACE for treatment of some lesions instead of targeting the tumor adjacent to the second porta hepatis. We are unable to prove that the patients did not benefit from TACE treatment. The data from patients with a single lesion who underwent TACE should be examined to explore this point. Therefore, a prospective trial providing a comparison between surgery and ablation is needed to determine which technique is more effective for the treatment of lesions close to the second porta hepatis.
Conclusion
This study demonstrated that patients with HCC adjacent to the second porta hepatis can be safely and effectively treated using CT-guided percutaneous thermal ablation therapy, especially for patients with tumors less than 3 cm in diameter and a distance between the tumor and the IVC of more than 5 mm who will achieve better local tumor control. Tumors adjacent to the second hepatic porta are no longer a contraindication for thermal ablation.
Disclosure statement
There is no conflict of interest regarding the publication of this article.
Additional information
Funding
References
- Bray F, Ferlay J, Soerjomataram I, et al. Global cancer statistics 2018: GLOBOCAN estimates of incidence and mortality worldwide for 36 cancers in 185 countries. CA Cancer J Clin. 2018;68:394–424.
- Llovet JM, Bruix J. Novel advancements in the management of hepatocellular carcinoma in 2008. J Hepatol. 2008;48:S20–S37.
- Benvegnu L. Natural history of compensated viral cirrhosis: a prospective study on the incidence and hierarchy of major complications. Gut. 2004;53:744–749.
- Fattovich G, Stroffolini T, Zagni I, et al. Hepatocellular carcinoma in cirrhosis: incidence and risk factors. Gastroenterology. 2004;127:S35–S50.
- Lau WY, Lai E. The current role of radiofrequency ablation in the management of hepatocellular carcinoma. Annals Surg. 2009;249:20–25.
- EASL–EORTC. Clinical practice guidelines: management of hepatocellular carcinoma. J Hepatol. 2012;56:908–943.
- Decadt B, Siriwardena AK. Radiofrequency ablation of liver tumours: systematic review. Lancet Oncol. 2004;5:550–560.
- Shibata T, Iimuro Y, Yamamoto Y. Small hepatocellular carcinoma: comparison of radio-frequency ablation and percutaneous microwave coagulation therapy. Radiology. 2002;223:331–337.
- Shiina S, Tateishi R, Arano T, et al. Radiofrequency ablation for hepatocellular carcinoma: 10-year outcome and prognostic factors. Am J Gastroenterol. 2012;107:569.
- Hemming AW, Reed AI, Langham MR, et al. Hepatic vein reconstruction for resection of hepatic tumors. Annals Surg. 2002;235:850–858.
- Nakamura S, Suzuki S, Konno H, et al. Resection of metastatic liver tumors with special reference to hepatic venous system. Hepatogastroenterology. 1998;45:24.
- Azoulay D, Andreani P, Maggi U, et al. Combined liver resection and reconstruction of the supra-renal vena cava: the Paul Brousse experience. Ann Surg. 2006;244:80.
- Lu DS, Raman SS, Vodopich DJ, et al. Effect of vessel size on creation of hepatic radiofrequency lesions in pigs: assessment of the “heat sink” effect. Am J Roentgenol. 2002;178:47–51.
- Lu DS, Raman SS, Limanond P, et al. Influence of large peritumoral vessels on outcome of radiofrequency ablation of liver tumors. J Vasc Interv Radiol. 2003;14:1267–1274.
- Huang S, Yu J, Liang P, et al. Percutaneous microwave ablation for hepatocellular carcinoma adjacent to large vessels: a long-term follow-up. Eur J Radiol. 2014;83:552–558.
- Gillams A, Lees W. The importance of large vessel proximity in thermal ablation of liver tumours. Radiology. 1999;213:123.
- Lee S, Kang TW, Cha DI, et al. Radiofrequency ablation vs. surgery for perivascular hepatocellular carcinoma: propensity score analyses of long-term outcomes. J Hepatol. 2018;69:70–78.
- Cardella JF, Kundu S, Miller DL, et al. Society of interventional radiology. Society of Interventional Radiology clinical practice guidelines. J Vasc Interv Radiol. 2009;20:S189–S191.
- Ahmed M, Solbiati L, Brace CL, et al. Image-guided tumor ablation: standardization of terminology and reporting criteria–a 10-year update. Radiology. 2014;273:241–260.
- Kang TW, Lim HK, Lee MW, et al. Perivascular versus nonperivascular small HCC treated with percutaneous RF ablation: retrospective comparison of long-term therapeutic outcomes. Radiology. 2014;270:888–899.
- Kim YS, Lim HK, Rhim H, et al. Ten-year outcomes of percutaneous radiofrequency ablation as first-line therapy of early hepatocellular carcinoma: analysis of prognostic factors. J Hepatol. 2013;58:89–97.
- Lee DH, Lee JM, Lee JY, et al. Radiofrequency ablation of hepatocellular carcinoma as first-line treatment: long-term results and prognostic factors in 162 patients with cirrhosis. Radiology. 2014;270:900–909.
- Nakazawa T, Kokubu S, Shibuya A, et al. Radiofrequency ablation of hepatocellular carcinoma: correlation between local tumor progression after ablation and ablative margin. Am J Roentgenol. 2007;188:480–488.
- Patterson EJ, Scudamore CH, Owen DA, et al. Radiofrequency ablation of porcine liver in vivo: effects of blood flow and treatment time on lesion size. Ann Surg. 1998;227:559.
- Chinn SB, Lee FT Jr, Kennedy GD, et al. Effect of vascular occlusion on radiofrequency ablation of the liver: results in a porcine model. Am J Roentgenol. 2001;176:789–795.
- Bitsch RG, Düx M, Helmberger T, et al. Effects of vascular perfusion on coagulation size in radiofrequency ablation of ex vivo perfused bovine livers. Invest Radiol. 2006;41:422–427.
- Frericks BB, Ritz JP, Albrecht T, et al. Influence of intrahepatic vessels on volume and shape of percutaneous thermal ablation zones: in vivo evaluation in a porcine model. Investig Radiol. 2008;43:211–218.
- Frericks BB, Ritz JP, Roggan A, et al. Multipolar radiofrequency ablation of hepatic tumors: initial experience. Radiology. 2005;237:1056–1062.
- Nault JC, Sutter O, Nahon P, et al. Percutaneous treatment of hepatocellular carcinoma: state of the art and innovations. J Hepatol. 2018;68:783–797.
- Brace CL, Laeseke PF, Sampson LA, et al. Microwave ablation with multiple simultaneously powered small-gauge triaxial antennas: results from an in vivo swine liver model. Radiology. 2007;244:151–156.
- Buscarini L, Buscarini E, Di Stasi M, et al. Percutaneous radiofrequency thermal ablation combined with transcatheter arterial embolization in the treatment of large hepatocellular carcinoma. Ultraschall Med. 1999;20:47–53.
- De Baere T, Bessoud B, Dromain C, et al. Percutaneous radiofrequency ablation of hepatic tumors during temporary venous occlusion. Am J Roentgenol. 2002;178:53–59.
- van Duijnhoven FH, Jansen MC, Junggeburt JM, et al. Factors influencing the local failure rate of radiofrequency ablation of colorectal liver metastases. Ann Surg Oncol. 2006;13:651–658.
- Kurokohchi K, Watanabe S, Masaki T, et al. Combined use of percutaneous ethanol injection and radiofrequency ablation for the effective treatment of hepatocelluar carcinoma. Int J Oncol. 2002;21:841–846.
- Peng ZW, Zhang YJ, Chen MS, et al. Radiofrequency ablation with or without transcatheter arterial chemoembolization in the treatment of hepatocellular carcinoma: a prospective randomized trial. J Clin Oncol. 2013;31:426–432.
- Peng ZW, Zhang YJ, Liang HH, et al. Recurrent hepatocellular carcinoma treated with sequential transcatheter arterial chemoembolization and RF ablation versus RF ablation alone: a prospective randomized trial. Radiology. 2012;262:689–700.
- Ren H, Liang P, Yu X, et al. Treatment of liver tumours adjacent to hepatic hilum with percutaneous microwave ablation combined with ethanol injection: a pilot study. Int J Hyper. 2011;27:249–254.