Abstract
Purpose: To evaluate the feasibility and therapeutic efficacy of 1.5-T MRI-guided and monitored microwave ablation in patients with hepatic malignant tumors.
Materials and Methods: This retrospective study was approved by the ethics committee of the First Affiliated Hospital of Fujian Medical University. Thirty-five patients (32 men, three women) with 48 lesions (maximum diameter < 3 cm) underwent microwave ablation under the guidance and monitoring of a 1.5-T MRI. The MRI-compatible microwave generator was appended with a magnetic shield case, and the cable was combined with a choke coil for shielding the Larmor processing frequency. The average age of the patients was 56.51 ± 11.36 years (31–77 years). Twenty-eight patients (37 lesions) displayed hepatocellular carcinoma and seven patients (11 lesions) had metastatic liver carcinoma. The mean maximum tumor diameter was 12.31 ± 4.72 mm (range 5.0–25.7 mm).
Results: MRI scans were performed simultaneously without electromagnetic interference during ablation. The average maximum diameter of the hypointense zone of the last monitored T2WI sequence, hypointense zone of post-procedure T2WI sequence, and hyperintense zone of post-procedure T1WI were 28.82 ± 7.58 mm, 29.79 ± 7.91 mm, and 28.28 ± 8.37 mm, respectively, with no observed statistical difference (F = 0.434, p = .649). The technical success rate was 100%. The average follow-up duration was 11.43 ± 5.29 (4–33) months. The technique efficacy rate was 100%.
Conclusion: MRI-guided and monitored microwave ablation of hepatic malignant tumors is feasible and potentially safe and effective.
MRI-guided microwave ablation of hepatic malignant tumors is feasible.
The MRI monitoring scan could accurately reflect the scope of ablation lesion.
On T1WI, the ablation lesions of liver showed the ‘target sign’.
Key points
Introduction
Microwave ablation (MWA) is widely used in the treatment of hepatic malignant tumors, with good curative effects [Citation1–4]. MWA is usually performed under ultrasound (US) or computed tomography (CT) guidance [Citation1,Citation3]. However, the accurate monitoring of the ablation process is difficult. The low resolution of tissue in unenhanced CT and the metal artifacts caused by the microwave antenna have an adverse impact on image quality of tumors, and sometimes, axial scans cannot display the full length of the microwave antenna. In addition, the unenhanced CT during ablation cannot clearly demonstrate the boundary of ablated lesions. The gases in the lungs and intestines interfere with ultrasound imaging and certain lesions, such as subphrenic lesions, cannot be displayed clearly on US. Furthermore, deep lesions are difficult to observe on US owing to the effects of high echogenicity during ablation. Enhancement can be used with CT and US to improve visualization, and fusion imaging can be used by MRI with excellent results [Citation5,Citation6]. However, both US and CT are inadequate for the comparison between the ablated lesion and the original lesion, the evaluation of immediate postoperative efficacy, and especially, the detection of minimal residual lesions [Citation7,Citation8].
Magnetic resonance imaging (MRI) is a promising guiding method for MWA, with the advantages of arbitrary direction imaging, high tissue resolution, and unique temperature sensitivity [Citation9–11]. However, general MWA systems are not compatible with MRI, and only a few institutes perform MRI-guided MWA [Citation9,Citation10]. The objective of the present study was to evaluate the feasibility of MRI-guided and monitored ablation of hepatic malignant tumors using a MRI compatible MWA system.
Materials and methods
Subjects
This retrospective study was approved by the ethics committee of the First Affiliated Hospital of Fujian Medical University. All patients provided written informed consent. Between September 2015 and December 2017, 299 patients with hepatic malignant tumors underwent percutaneous MWA. A total of 121 patients were guided by CT, 100 patients by US, and 78 patients by MRI. Of 78 cases with MRI-guided MWA, 35 patients (32 men and three women) with 48 pathologically confirmed hepatic malignant tumors were included this study (). The inclusion criteria were as follows: at least one tumor was visible on MRI only and could not be detected using unenhanced CT or US, maximum lesion diameter of <3 cm and ≤3 lesions (hepatocellular carcinoma), <3 cm and ≤5 lesions (hepatic metastasis), liver function of Child–Pugh grade A or B, and the absence of extrahepatic metastases. The exclusion criteria were as follows: diffuse intrahepatic tumor, extrahepatic spread, portal thrombosis, Child–Pugh class C cirrhosis, prothrombin activity less than 50%, or a platelet count lower than 50 cells × 109/L. Four patients had two lesions, three patients displayed three lesions, and one showed four lesions. The average age of the patients was 56.51 ± 11.36 years old (range of 31–77 years old). Twenty-eight patients had hepatocellular carcinoma and seven displayed hepatic metastasis. The average maximum diameter of the tumors was 12.31 ± 4.72 mm (range of 5.0–25.7 mm) (). In another 43 patients who received MRI-guided MWA, twenty-eight patients had a maximum lesion diameter of >3 cm, eight patients with HCC had more than three tumors, and seven patients with hepatic metastasis had more than five tumors.
Table 1. Patient characteristics.
Equipment
In the present study, the MRI-compatible microwave ablation system (2450 MHz, MTC-3C, Vision Medical, Nanjing, China) and a microwave antenna (MTC-3CA-II, 2.0 mm, 15 cm, Vision Medical, Nanjing, China) were used. The microwave generator was appended a magnetic shield case (made of aluminum alloy), and the head and tail of the cable (2.5 m) included a choke coil for shielding the Larmor processing frequency of 1.5 T MRI (63.75 MHz). Therefore, the MRI monitored scanning could be performed simultaneously without electromagnetic interference during ablation.
MWA was guided by a 1.5-T MRI scanner (Signa Infinity TwinSpeed with Excite, GE, WI, USA). Torso body or cardiac coil was used during MRI. The MRI scanning sequences and parameters used in our study have been listed below:
Fat-suppressed fast-recovery fast spin echo (fs FRFSE) T2WI: repetition time (TR) 8500.0 ms, echo time (TE) 85.0 ms, flip angle (FA) 90°, echo train length (ETL) 17, slice thickness (ST) 5.0 mm, GAP 1.0 mm, field of view (FOV) 280 × 380, number of excitations (NEX) 1, and scanning time (T) 70–90 s.
Monitoring fs FRFSE T2WI: TR 2600.0 ms, TE 85.0 ms, FA 90°, ETL 17, ST 5.0 mm, GAP 1.0 mm, FOV 280 × 380, NEX 1, and T 35 s.
Three-dimensional dynamic T1 weighted imaging (3D Dyn T1WI): TR 4.8 ms, TE 1.1 ms, FA 45°, ETL 1, ST 3.0 mm, FOV 280 × 380, NEX 1, and T 12 s.
The fs FRFSE T2WI and monitoring fs FRFSE T2WI sequence used respiratory gating-controlled scanning, and the 3 D Dyn T1WI sequences used breath-holding scanning.
MWA procedure
Preoperative preparation
Approximately two weeks before the surgery, contrast-enhanced MRI was performed to confirm the location, size, and number of lesions. The appropriate patient position depended on the need for puncture. Blood pressure, heart rate, and respiration were monitored. Before the procedure, patients were intramuscularly administered 10 mg morphine hydrochloride to minimize pain.
Procedure
Prior to MWA, an unenhanced MRI (fs FRFSE T2WI and 3 D Dyn T1WI sequence) was performed to localize each lesion (). A set of cod liver oil capsules were placed on the skin as a guide for antenna placement, and the 3 D Dyn T1WI sequence was performed to determine the puncture path, which was as short as possible and avoided important structures. Iodine disinfection and 2% lidocaine local anesthesia were provided, and the coil was wrapped with a sterile cover. Under MRI guidance, a microwave antenna was gradually inserted into the center of the tumor. The antenna tip was beyond the distal tumor 0.5–1 cm. During the puncture, multiple scans were performed to ensure that the needle was in the right direction, and the scan direction was parallel to the microwave antenna. The cable and water pump were connected to the MWA apparatus. Ablation was performed at 80–90 W power for 3–15 min, simultaneously; a series of monitoring fs FRFSE T2WI sequences were performed continuously to monitor the ablated scope every 35 s (). The MWA was powered off until the ablated area covered the entire lesion and beyond the edge 0.5–1 cm. An unenhanced MRI scan (fs FRFSE T2WI and 3 D Dyn T1WI) was performed to assess the immediate treatment outcomes and complications (). If the ablative zone, which was demarcated a T1 hyperintense or T2 hypointense area, did not cover the target tumor, ablation was continued with the same antenna position or the antenna was repositioned (). Once the lesion was completely ablated, the puncture tract was ablated, and the antenna was removed ().
Figure 2. A 35-year-old man presented with recurrent hepatocellular carcinoma after radiofrequency ablation. (A) A nodule is seen in the left lateral lobe of the liver. The fsFRFSE T2WI sequence shows a hyperintense lesion (arrow). (B) The 3 D Dyn T1WI sequence shows a hypointense lesion.
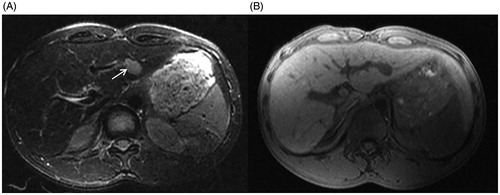
Figure 3. The procedure of MRI-guided MWA in the same patient as in . (A) During ablation, the monitoring fsFRFSE T2WI was performed every 35 s, the microwave antenna appears as a thin strip-like structure with low signal intensity (arrow) and the signal intensity of tumor was gradually decreased with ablative time. Images are presented as pre-ablation (upper left), 280 s post-ablation (upper right), and 425 s (lower left). The post-ablative scanning shows a residual lesion is present on the right border, the residual lesion is hyperintense on T2WI (lower right). (B) The residual lesion is hypo-intensity shown on T1WI (arrow). (C) The microwave antenna was adjusted, and a complementary ablation was performed. The monitoring fsFRFSE T2WI demonstrated the signal intensity of tumor gradually decreased with ablative time; the ablated normal liver tissue was isointense or slightly hypointense with a thin hyper-intensive rim (arrow). Images are presented preablation (upper left), 315 s post-ablation (upper right), and post-ablation (lower left). After ablation, the characteristic ‘target sign’ is seen on the 3 D Dyn T1WI sequence (lower right). The ablated lesion still appears hypointense, whereas the surrounding ablated area appears hyperintense. (D) On T2WI, the ablated lesion appears slightly hypointense with 2 strips of hyperintense at the antenna path (arrow).
Follow-up
Follow-up contrast-enhanced liver MRI was performed at one-month postoperative and every 3–4 months thereafter. The follow-up imaging was assessed by a radiologist with 15 years of abdominal MRI experience. The patients were followed until June 2018 or death.
Data analysis
The MRI pre- and post-ablative appearance of the tumors and lesions were observed. The maximum diameter of the hypointense zone of the last monitored fs FRFSE T2WI sequence, hypointense zone of the post-procedure fs FRFSE T2WI sequence, and the hyperintense zone of the post-procedure 3 D Dyn T1WI were recorded. MWA duration and complications were recorded.
Technical success was assessed based on the unenhanced MRI immediately following the MWA. The procedure was considered to be technically successful if the ablation zone completely overlapped the target tumor [Citation12]. Technique efficacy was defined as complete ablation at initial follow-up MRI 1-month after MWA [Citation12]. If no enhancement of the original lesion was observed, it was assumed that the tumor ablation was complete. Local tumor progression was defined as detection of enhanced nodules in the surrounding ablation zone during follow-up.
Statistical analyses were performed by SPSS (version 22.0, IBM, Chicago, IL, USA). Continuous variables were shown as means ± standard deviation. The analysis of variance (ANOVA) was used to assess quantitative data. Differences were considered significant when P values were smaller than 0.05.
Results
MRI findings
Pre-ablative imaging
The tumor demonstrated hyperintense on T2WI and hypointense on 3 D Dyn T1WI (). The microwave antenna appeared as a strip of low signal intensity on each MRI sequence ().
Monitoring imaging
The signal intensity of the tumors was gradually decreased with ablative time on monitoring fs FRFSE T2WI images. The ablative scope was gradually expanded, and the ablated normal liver tissue was slightly hypointense with a thin hyperintensive rim ().
Post-ablative imaging
At the center of the ablated lesion, a long T1 and long T2 strip signal of antenna path was observed. The ablation zone appeared as a spherical hypointense area surrounded by a thin hyperintense rim on T2WI. On T1WI, there were three layers signal intensity. The ablated tumor signal still appeared hypointense on T1WI, whereas the surrounding normal liver tissue that had been ablated appeared hyperintense with a clear boundary, which constituted a typical ‘target sign’. The outermost ablated lesion was a thin hypointense rim that surrounded the hyperintense lesion ().
MWA duration and complications
The mean duration of the MWA procedure was 65.69 ± 25.74 min (range 31–123 min). During the ablation, six patients showed obvious pain and one patient vomited, but each patient was able to tolerate the procedure after analgesia. Post-procedure, two patients exhibited a high fever (more than 38.5 °C), however their body temperature returned to normal after a few days of anti-infection treatment. We observed no instances of serious complications, such as massive hemorrhaging, biliary fistula, diaphragmatic perforation, or jaundice.
Therapeutic effect
The technical success rate was 100%. The average maximum diameter of the hypointense zone of the last monitored fs FRFSE T2WI sequence was 28.82 ± 7.58 mm (16.3–46.7 mm), the hypointense zone of the post-procedure fs FRFSE T2WI sequence was 29.79 ± 7.91 mm (15.8–48.1 mm), and the hyperintense zone of the post-procedure 3 D Dyn T1WI was 28.28 ± 8.37 mm (13.3–47.7 mm); we observed no statistically significant difference (F = 0.434, p = .649). The average follow-up duration was 11.43 ± 5.29 months (range 4–33 months). No local recurrence was found (). The technique efficacy rate was 100%. New lesions were found in 16 patients (13 HCC and three metastases) during follow-up.
Figure 4. 11 months after MWA, the MRI of the same patient as in and . (A) The fsFRFSE T2WI demonstrated the ablated lesion was isointense. (B) The unenhanced 3 D Dyn TIWI demonstrated the ablated lesion was hyperintense (arrow). (C) The enhanced equilibrium phase MRI displayed an unenhanced ablated lesion (arrow).
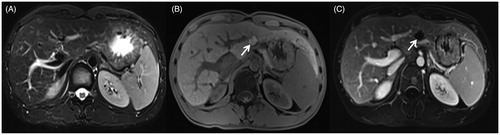
Discussion
MRI has many advantages, such as lack of ionizing radiation, high resolution, no bone and gas artifacts, displays lesions that are difficult to observe on CT and US, visualization of blood vessels without a contrast agent, and multi-directional imaging capability [Citation5]. Yu NC et al. analyzed data from 638 consecutive adult patients with cirrhosis who received liver transplants within six months of imaging at their institution, the lesion-based sensitivities of US, CT, and MRI were 46%, 65%, and 72%, respectively [Citation13]. Of the 48 tumors in the present cases, 13 tumors could not be detected on enhanced US or CT.
The greatest advantage of MRI for MWA is the display of ablated tissue, which appears hyperintense on T1WI and hypointense on T2WI with clear, well-defined edges. MRI signal intensity is closely related to tissue water content. As ablation reduces the water content of the tissue, the T1 and T2 values decrease and the lesions appear hyperintense on T1WI and hypointense on T2WI [Citation14,Citation15]. By comparing spoiled gradient (SPGR) sequence images obtained after hepatic ablation using enhanced CT imaging obtained 1-week post-operatively, Khankan et al. found that the use of unenhanced T1WI scans to evaluate the extent of ablation was accurate [Citation16]. Shyn PB, et al. found that the cryo-ablated tumor (‘ghost’) was often visualized with MRI, enabling delineation of the ablation margin on at least one or more MRI sequences [Citation17]. In addition, the present study found that in liver lesions the signal intensity differed between the ablated tumor tissue and the surrounding ablated normal liver tissue [Citation18]. The hepatic sinusoids of normal liver tissue surrounding tumors were significantly expanded under the heat effect and were filled with many red blood cells. After ablation, the red blood cells and liver tissue showed coagulative necrosis, and the signal intensity was significantly increased on T1WI in accord with bleeding. However, the tumor area lacked hepatic sinusoid, only a small amount of red blood cells oozed and accumulated. This apparent difference in the amount of red blood cells between the ablated area of the original tumor foci and the ablated liver tissue was the formative reason of the ‘target sign’ shown on T1WI [Citation19]. Therefore, we considered that this sign is useful to ascertain the extent of ablation and guide the ablation of any residual tumor.
CT and ultrasound can be performed during MWA. However, CT only displays the decreased density of the ablative lesion, and the boundary is unclear. Due to bubbles generated during MWA, the ultrasonogram of the ablated area shows hyper-echo with a blurred boundary and interference of the deep structure. During conventional MWA, the magnetic field was interrupted and the monitoring imaging could not be performed. In our study, the microwave generator, connecting cable, and antenna were made with MRI-compatible materials and modified by shield and choke. Therefore, the scanning of T2WI sequences could be performed at the same time of ablation without magnetic field interference. In our study, the monitoring imaging could quasi real-time display the signal change of the tumor and surrounding tissue, monitor the scope of ablation, which could effect judgement and normal tissue protection, thus provide a visualized ablation.
Hoffmann R et al. performed MRI-guided and monitored MWA in 15 patients with 18 hepatic malignancies [Citation20]; the monitoring sequences were T1 Flash 2 D, T1 VIBE, and T2TrueFISP. In our study, the fsFRFSE T2WI was used to monitor the ablative zone, and the signal intensity decreased during ablation. As the border of ablated tissue was injured and hyperemia occurred, a slightly hyper-intensive rim was formed. Based on our experience, the signal of the T1 Flash 2 D, T1 VIBE, and T2TrueFISP sequences were insensitive or slightly changed during ablation, and the fsFRFSE T2WI sequence could reflect the ablative scope more accurately than those of the other sequences, although the scan time was longer (35 s).
Image fusion and virtual navigation are receiving great interest as a potential guiding method useful to overcome the limitations of single US or CT [Citation21]. This approach sketches the target lesion, calibrates the direction, real-time monitors the puncture cite, and is more widely applicable than guidance by MRI. However, the deformation and displacement by respiratory movement and position change reduces the accuracy of image registration. Upon evaluation of efficacy, MRI is more intuitive and accurate than the image fusion approach.
The present study has certain shortcomings. Our study is a retrospective study. MRI is a more complex procedure compared with CT or US, and the present study lacked comparison with other guiding modalities. Patients with pacemakers or metal implants cannot undergo MRI. The 35 s monitoring imaging using temporal resolution was not real-time. Our study used closed MRI, although this procedure ensured high field strength, fast imaging, and image clarity, the operation time was prolonged because of the need to repeatedly move the examination bed during the procedure. In addition, temperature-measurement software was not used to monitor the ablation process in real time. Finally, the study included only 35 patients with 48 lesions and had a short follow-up duration, because the purpose of this study was to determine the feasibility of MRI-guided and monitored MWA of hepatic malignant tumors. In future studies, a larger sample size and a longer follow-up will be required to explore the clinical significance of this technique.
In conclusion, we have shown that the MWA of hepatic tumors can be performed under 1.5-T conventional MRI guidance and monitoring. The procedure was found to be feasible, with good curative effects and few complications.
Disclosure statement
No potential conflict of interest was reported by the authors.
References
- Filippiadis DK, Spiliopoulos S, Konstantos C, et al. Computed tomography – guided percutaneous microwave ablation of hepatocellular carcinoma in challenging locations: safety and efficacy of high-power microwave platforms. Int J Hyperthermia. 2018;34(6):863–869.
- Qi H, Zhang H, Wan C, et al. CT-guided microwave ablation through the lungs for treating liver tumors near the diaphragm. Oncotarget. 2017;8(45):79270–79278.
- Wang G, Sun Y, Cong L, et al. Artificial pleural effusion in percutaneous microwave ablation of hepatic tumors near the diaphragm under the guidance of ultrasound. Int J Clin Exp Med. 2015;8:16765–16771.
- Tinguely P, Fusaglia M, Freedman J, et al. Laparoscopic image-based navigation for microwave ablation of liver tumors-A multi-center study. Surg Endosc. 2017;31(10):4315–4324.
- Mauri G, Porazzi E, Cova L, et al. Intraprocedural contrast-enhanced ultrasound (CEUS) in liver percutaneous radiofrequency ablation: clinical impact and health technology assessment. Insights Imaging. 2014;5(2):209–216.
- Calandri M, Mauri G, Yevich S, et al. Fusion imaging and virtual navigation to guide percutaneous thermal ablation of hepatocellular carcinoma: a review of the literature. Cardiovasc Intervent Radiol. 2019;42(5):639–647.
- Lin ZY, Chen J, Deng XF. Treatment of hepatocellular carcinoma adjacent to large blood vessels using 1.5T MRI-guided percutaneous radiofrequency ablation combined with iodine-125 radioactive seed implantation. Eur J Radiol. 2012;81(11):3079–3083.
- Ringe KI, Wacker F, Raatschen HJ. Is there a need for MRI within 24 hours after CT-guided percutaneous thermoablation of the liver?. Acta Radiol. 2015;56(1):10–17.
- Murakami K, Naka S, Shiomi H, et al. Initial experiences with MR Image-guided laparoscopic microwave coagulation therapy for hepatic tumors. Surg Today. 2015;45(9):1173–1178.
- Sato K, Morikawa S, Inubushi T, et al. Alternate biplanar MR navigation for microwave ablation of liver tumors. MRMS. 2005;4(2):89–94.
- Limanond P, Zimmerman P, Raman SS, et a1. Interpretation of CT and MRI after radiofrequency ablation of hepatic malignancies. AJR. 2003;181(6):1635–1640.
- Ahmed M, Solbiati L, Brace CL, et al. Image-guided tumor ablation: standardization of terminology and reporting criteria–a 10-year update. Radiology. 2014;273(1):241–260.
- Yu NC, Chaudhari V, Raman SS, et al. CT and MRI improve detection of hepatocellular carcinoma, compared with ultrasound alone, in patients with cirrhosis. Clin Gastroenterol Hepatol. 2011;9(2):161–167.
- Dong J, Geng X, Yang Y, et al. Dynamic imaging and pathological changes in pig liver after MR-guided microwave ablation. BMC Cancer. 2018;18(1):397.
- Jia ZY, Chen QF, Yang ZQ, et al. Early assessment of coagulation necrosis after hepatic microwave ablation: a comparison of non-enhanced and enhanced T1-weighted images. Abdom Radiol. . 2017;42(6):1781–1787.
- Khankan AA, Murakami T, Onishi H, et, al. Hepatocellular carcinoma treated with radio frequency ablation: an early evaluation with magnetic resonance imaging. J Magn Reson Imaging. 2008;27(3):546–551.
- Shyn PB, Mauri G, Alencar RO, et al. Percutaneous imaging-guided cryoablation of liver tumors: predicting local progression on 24-hour MRI. AJR Am J Roentgenol. 2014;203(2):W181–91.
- Lin Z-Y, Li G-L, Chen J, et al. Effect of heat sink on the recurrence of small malignant hepatic tumors after radiofrequency ablation. J Can Res Ther. 2016;12(7):153–138.
- Chen YP, Lin ZY, Song QQ, et al. Experimental study on MRI-guided radiofrequency ablation in treatment of VX2 tumor adjacent to blood vessels in rabbit liver. Chin J Interven Imaging Therapy. 2016;13:500–504.
- Hoffmann R, Rempp H, Keßler DE, et al. MR-guided microwave ablation in hepatic tumours: initial results in clinical routine. Eur Radiol. 2017;27(4):1467–1476.
- Mauri G. Expanding role of virtual navigation and fusion imaging in percutaneous biopsies and ablation. Abdom Imaging. 2015;40(8):3238–3239.