Abstract
Objective
To establish the technique of intratumoral combination therapy of radiofrequency hyperthermia (RFH) with herpes simplex virus-thymidine kinase/ganciclovir (HSV-TK/GCV) gene therapy for rat ovarian cancers.
Material and methods
This study consisted of three parts: (1) in vitro experiments to establish the ‘proof of principal’ that combination of RFH and HSV-TK gene therapy has the synergistic effect on human ovarian cancer cells; (2) creation of bioluminescence imaging-detectable rat ovarian cancer model; and (3) in vivo experiments using this rat model to validate the technical feasibility of the combination therapy. Cells and nude rats were divided into four groups: (i) combination therapy (HSV-TK/GCV + RFH); (ii) RFH; (iii) HSV-TK/GCV; and (iv) phosphate-buffered saline (PBS). Data were analyzed using Dunnett t-test or Kruskal–Wallis test.
Results
Cell proliferation assay demonstrated significantly greater reduction in viable cells with the combination therapy [0.52 (0.43, 0.61)] compared to other treatments [RFH 0.90 (0.84, 0.96), HSV-TK/GCV 0.71 (0.53, 0.88), PBS 1 (1, 1); p < .05]. For 24 rat models with bioluminescence imaging-detectable orthotopic ovarian cancer (n = 6 per group), optical imaging demonstrated significantly decreased relative bioluminescence signal with the combination therapy [0.81 (0.52, 1.08)] compared to other treatments [RFH 3.60 (2.34, 4.86), HSV-TK/GCV 2.21 (1.71, 2.71), PBS 3.74 (3.19, 4.29); p < .001]. Ultrasound imaging demonstrated the smallest relative tumor volume with the combination therapy [0.78 (0.45, 1.11) versus 3.50 (2.67, 4.33), 2.10 (0.83, 3.37), 3.70 (1.79, 5.61); p < .05].
Conclusion
The feasibility of intratumoral RFH-enhanced HSV-TK/GCV gene therapy was established on a unique rat model with molecular imaging-detectable orthotopic ovarian cancer.
Introduction
Ovarian cancer accounts for 2.5% of all malignancies in women and 5% of female cancer deaths [Citation1]. In 2018, there were approximately 22,240 newly diagnosed cases of ovarian cancer and 14,070 ovarian cancer deaths in the United States, representing the most common cause of death due to gynecologic malignancy [Citation1–3].
Early detection is the key to successful management of ovarian cancer; however, ovarian cancer is rarely diagnosed at an early stage because the disease causes few specific symptoms when it is localized to the ovary. Ovarian cancer has therefore long been labeled as the ‘silent killer’ [Citation2]. Approximately 75% of women with ovarian carcinoma present with stages III or IV disease without any chance of curative surgery [Citation2]. The current standard of care for ovarian cancer is an optimal cytoreductive surgery followed by a combination chemotherapy with platinum and taxane [Citation1–4]. Unfortunately, a majority of women with advanced-stage ovarian cancer will relapse due to platinum-resistant or refractory cancer, with a median time to recurrence of 16 months [Citation5].
Therefore, there has been growing interest in developing innovative strategies for targeted treatment of this pathology [Citation6]. Gene therapy represents a promising therapeutic option, in which introduction of therapeutic genetic material into specific target cells can alter gene expression as a means to treat the disease [Citation7]. Cancer treatment is the leading application for gene therapy, accounting for 65% of gene therapy clinical trials [Citation8]. The most heavily investigated tumor suicide gene therapy involves using the herpes simplex virus-thymidine kinase (HSV-TK) gene/ganciclovir (GCV) system, where a pro-drug, GCV, is activated by TK expressed in cells into the active triphosphate form, which kills not only the targeted tumor cells but also neighboring cells via the by-stander effect [Citation6]. This cancer suicide gene therapy system has been used as an effective and valuable approach in treatment of many cancer types, including hepatocellular carcinoma [Citation7], ovarian cancer [Citation8], and non-small cell lung cancer [Citation9]. Although a variety of preclinical studies in rodent models confirmed the efficacy of Herpes simplex virus-thymidine kinase/ganciclovir (HSV-TK/GCV), it has not been demonstrated in clinical studies, primarily due to the low gene transduction efficiency via systemic administration [Citation10] and the lack of a safe and highly efficient gene delivery system [Citation11]. Our previous studies demonstrated that radiofrequency hyperthermia (RFH) delivered by a radiofrequency (RF) electrode can enhance lentivirus-mediated gene transfection efficiency in mouse subcutaneous hepatic cancer xenografts [Citation12], rat orthotopic lung cancer [Citation13], and rat orthotopic esophageal cancer [Citation14]. Additionally, a direct intratumoral injection of high-dose HSV-TK/lentivirus genes using a multifunctional thermal perfusion RF electrode may improve the HSV-TK gene transfection and thus maximize its clinical potential [Citation14].
Animal models that closely mimic human ovarian cancer are crucial for understanding its pathogenesis and for testing new treatment strategies [Citation15]. Monitoring the development, progression, and treatment response of the disease is usually conducted by measuring the macroscopic morphological changes using conventional imaging techniques, such as computed tomography (CT), magnetic resonance imaging, or ultrasonography. However, these imaging modalities do not quantitatively reflect the bioactivities of tumors at a molecular level. It would be useful to develop an ovarian cancer animal model with imaging markers that facilitate the longitudinal monitoring of tumor biology by using a noninvasive molecular imaging modality. Therefore, the aim of this study was to create a rat ovarian cancer model that can be assessed using molecular imaging and to use this model for testing the feasibility of intratumoral RFH-enhanced HSV-TK/GCV gene therapy.
Materials and methods
Study design
Our Institutional Animal Care and Use Committee approved the experimental protocol in nude rats. This study included three phases: (1) in vitro experiments to evaluate the synergistic effect of RFH combined with HSV-TK/GCV gene therapy on human ovarian cancer cells; (2) creation of a rat model with bioluminescence optical imaging-detectable orthotopic ovarian tumors; (3) in vivo experiments using this model to validate the technique of intratumoral RFH-enhanced HSV-TK/GCV gene therapy.
In vitro experiments
Tumor cell line with sustained luciferase expression
Human ovarian cancer cells (SK-OV-3, ATCC, Manassas, VA) were cultured in McCoy's 5 A medium (ATCC® 30-2007™) supplemented with 10% fetal bovine serum in a humidified atmosphere of 5% CO2. The cells were then transfected with mCherry/Luciferase/lentivirus based on the manufacturer’s protocol (GeneCopoeia Inc., Rockville, MD) to create sustained mCherry/Luciferase-positive cells. The cells with stable expression of mCherry/Luciferase were selected using fluorescence activated cell sorting (FACS) technique (Aria II, Bio-rad, Hercules, CA). Cells were maintained and propagated by a few passages, and fluorescent microscopy confirmed that more than 95% of cells retained mCherry fluorescence throughout the study.
Combination therapy of RFH and GFP/HSV-TK gene therapy
Recombinant lentivirus-carrying green fluorescence protein (GFP)/HSV-TK was produced by transient transfection of 293FT cells according to the manufacturer’s protocol (Applied Biological Materials Inc, Richmond, the British Columbia, Canada). Briefly, 10 μg of GFP/HSV-TK lentiviral expression vector (pLenti-III-HSVtk, LV047, Applied Biological Materials Inc.), 10 μg of 3rd generation packaging system mix (Applied Biological Materials Inc.), and 80 μL of transfection reagent (Applied Biological Materials Inc.) were used to transfect 293FT cells. The viral supernatant was concentrated and purified using Ultra-Pure Lentivirus Purification Kit based on the manufacturer’s protocol (Applied Biological Materials Inc). Viral titer was determined based on the expression of GFP in transduced 293FT cells as previously described [Citation12,Citation14].
2 × 104 Luciferase-positive SK-OV-3 cells were cultured in each chamber of four-chamber cell culture slides (Nalge Nunc International, Rochester, NY) in a 37 °C water bath. The cells in four groups received different treatments: (1) combination of GFP/HSV-TK gene therapy at a MOI of 20/well with RFH at 41–42 °C for 30 min, followed by GCV exposure; (2) RFH alone; (3) GFP/HSV-TK gene therapy at a MOI of 20/well alone with subsequent GCV exposure; and (4) PBS as the control. Additional two groups of cells were used to evaluate GFP/HSV-TK gene expression level with and without RFH enhancement: (1) cells transfected with lentiviral GFP/HSV-TK gene followed by RFH at 41–42 °C for 30 min and (2) cells transfected with lentiviral GFP/HSV-TK gene alone. Fluorescent microscopy was used to observe GFP fluorescence in cells for 7 days. RFH was delivered to cells by a custom-made 0.022-inch RF probe attached under the bottom of one chamber and then connected to a RF generator. A sterilized 1.1-mm fiber optic temperature probe was attached to the bottom of the 4th chamber and connected to a thermometer (Photon Control, Burnaby BC, Canada) for real-time monitoring of the temperature during RFH. The cells with expression of GFP were quantified using fluorescence activated cell sorting (FACS) technique (Aria II, Bio-rad, Hercules, CA).
Cell viability was evaluated by MTS assay (3-(4,5-dimethylthiazol-2-yl)-2,5-diphenyltetrazolium bromide) after 72-h exposure to GCV. MTS agent was added to the medium, and the cells were incubated for 4 h. The absorbance of the cell medium was measured at 490 nm using SpectraMax iD3 plate reader (SpectraMax, Molecular Devices, Sunnyvale, CA). Relative cell proliferations were calculated using the equation: (Atreated − Ablank)/(Acontrol − Ablank). Cells on slides were subsequently counterstained with DAPI (Vector Laboratories, Burlingame, CA) and imaged with fluorescent microscopy. Six experiments were repeated for each group.
In vitro bioluminescent imaging (BLI) was performed to further evaluate the treatment effect on cells. Cells in each group were incubated with 5 μL of 100 mg/ml Pierce D-Luciferin (Thermo Fisher Scientific, Rockford, IL) for 20 min. Cells collected from the chambers were resuspended in 50-μL PBS and then mixed with 50 μL of 1% agarose (Invitrogen, Carlsbad, CA) in a transparent cylindrical glass tube. BLI was performed for each glass tube (Bruker In-Vivo Xtreme, Billerica, MA). Bioluminescence signal intensity was calculated as the mean of all detected photon counts within a manually drawn region of interest (ROI) using Bruker MI software. Relative signal intensity (RSI) was determined by the following equation: RSI = SIT/SIC, where SI represents signal intensity, T represents the treatment group, and C represents the control group.
In vivo experiments
Creation of rat models with molecular imaging-detectable orthotopic ovarian cancer
Twenty-four female athymic nude rats (Charles River Laboratories, Wilmington, MA) aged 6–8 weeks were used to create a rat orthotopic ovarian cancer model. The rats were anesthetized with mask inhalation of 2% isoflurane delivered in oxygen at a flow rate of 1 L/min. A 2-cm incision was made on the left flank by means of aseptic surgery to expose the left ovary. 0.5–1 × 107 mCherry/Luciferase-labeled SK-OV-3 cells, mixed in 20 μL of PBS and 20 μL of Matrigel (Corning Inc. Bedford, MA), were slowly injected into the ovary. A gelatin sponge was applied to compress the cell injection site to prevent cell suspension leakage and bleeding. Finally, the incision was closed with absorbable sutures in layers.
The rats with intraovarian cell inoculations were monitored by BLI and ultrasound imaging once a week to detect the tumor formation in the ovary. BLI was performed with the Bruker In-Vivo Xtreme X-ray/optical imaging system, equipped with a highly sensitive cooled charge-coupled camera mounted in a light-tight box (Bruker In-Vivo Xtreme, Billerica, MA). The commercially available Bruker MI software (Bruker, Billerica, MA) was used to acquire and analyze the images. Twenty minutes after intraperitoneal administration of D-luciferin potassium salt in saline (150 mg/kg body weight), animals were placed in an air-tight box in the imaging chamber with continuous inflow of 2% isoflurane in oxygen. BLI was acquired at an exposure time of 8 min and emission wavelength of 530 nm and superimposed on the X-ray images. ROIs covering the entire tumor with bioluminescence signal were selected, and the total photon counts were determined. At the same time point, ultrasound images of the ovaries were acquired to further confirm the formation of ovarian cancer and measure the size of the tumors. Three weeks after the intraovarian cancer cell inoculation, 10 rats were sacrificed every week within 8 weeks, with 1 rat per week in the 6 weeks and 2 per week the rest of 2 weeks, for pathological confirmation of ovarian cancer.
RFH combined with HSV-TK gene therapy for orthotopic rat ovarian cancer
Twenty-four female nude rats bearing orthotopic ovarian tumors were randomized to four treatment groups (n = 6/group) when the tumor size reached 8–10 mm: (1) combination therapy group with intratumoral injection of lentiviral GFP/HSV-TK gene, followed by RFH at 41–42 °C for 30 min, and then intraperitoneal injection of 5 mg/kg GCV daily for 7 days; (2) intratumoral RFH alone; (3) intratumoral injection of lentiviral GFP/HSV-TK gene alone; and (4) PBS as the control. 50 μL HSV-TK/lentivirus (1 × 108 pfu) was directly injected into the tumor via the perfusion needles of an 18 G multipolar RF electrode (Welfare medic, Beijing, China) under ultrasound imaging guidance (Sonosite Inc, Bothell, WA). The intratumoral RFH with intratumral gene injection was performed by precisely placing the RF electrode with multichannel injection needles into the tumor under ultrasound imaging guidance. After confirming the appropriated position of the RF electrode within the tumor, the electrode was connected to RF generator (Welfaremedic, Beijing, China) to deliver thermal energy to the tumors, at approximately 42 °C for 30 min. The RF electrode has multiple functions that enable delivery of RFH, therapeutic drug injection, and temperature monitoring simultaneously. The thermal couples integrated in the RF needles functioned to measure the temperature change, which was displayed on the computer of the RF generator in a real-time mode. Immediately after the intratumoral gene delivery, RFH at 41–42 °C was generated while the temperature within the tumor was monitored by the thermal sensors at the perfusion needle tip. GCV (50 mg/kg) was intraperitoneally administered for 7 days immediately after the gene delivery. BLI was used to follow up the changes of the bioluminescence signals and size of tumors at 7 and 14 days after the treatments.
Ultrasound imaging was performed to measure tumor size changes at 0, 7, and 14 days after the treatments. The tumor size was measured in three orthogonal axes, with the X and Y axes defining the largest planar dimension and the Z axis defining the depth. The volume of each tumor was calculated using the following equation: tumor volume=X*Y*Z*π/6. Data were normalized to relative tumor volume (RTV) using the equation: RTV=VDn/VD0, where V stands for tumor volume, Dn represents the number of days after treatment, and D0 represents the day before treatment.
BLI was used to acquire the bioluminescence signals of tumors at 0, 7, and 14 days after the treatments. After intraperitoneal injection of 150 mg/kg of D-luciferin, images were captured at an exposure time of 8 min and emission wavelength of 530 nm. Signal intensity was quantified using the Bruker software. RSI was calculated using the equation: RSI = SIDn/SID0, where SI stands for signal intensity, Dn represents the number of days after treatment, and D0 represents the day before treatment.
Western blotting to quantify GFP/HSV-TK gene expression in cells
Ovarian tumors were processed by homogenization. The expression level of GFP gene in tumor cells were quantified by western blotting analysis according to the protocol provide by Thermo Fisher Scientific (Thermo Fisher Scientific, Rockford, IL). The level of gene expression was quantified by measuring the density of the immunoreactive bands and subsequently normalizing it to the relative areas (RA) according to the equation: RA=ARFH+gene/Agene, where A represents the chemiluminescence area.
Pathology
Tumor tissues were fixed in 4% paraformaldehyde solution, embedded in paraffin, and sectioned at 5 μm. The tissue slides were stained with hematoxylin and eosin (H&E) to confirm the formation of cancer nodules. Apoptosis of treated tumors was analyzed by immunohistochemical staining using the terminal deoxynucleotidyl transferase-mediated dUTP nick end labeling (TUNEL) apoptosis detection kit (R&D Systems, Minneapolis, MN). Ki-67 antibody was used to evaluate the cell proliferation according to the manufacturer's protocol (Abcam, Cambridge, MA). We used ImageJ software (NIH Bethesda, MD) to count the cells with positive staining of TUNEL and Ki67.
Statistical analysis
Results are expressed as mean ± 95% confidence interval. Statistical analyses were performed using the software SPSS 22.0 (SPSS Inc., Chicago, IL). Dunnett t-test was used to estimate the relative viabilities of cells assessed by the cell proliferation assay, relative BLI signals, apoptotic index, tumor size, as well as Ki-67 levels to compare different treatment groups. Kruskal–Wallis test was used to compare the relative BLI of cells and apoptotic index of rat tumors between the combination group and each of the other groups. Two-tailed p value of less than .05 was considered statistically significant.
Results
Synergistic effect of RFH with HSV-TK gene therapy on ovarian cancer cells
Confocal microscopy () and the time-to-GFP expression curve (Figure1(b)) showed that GFP expression reached its peak on day 5 after cells were transfected with lentiviral GFP/HSV-TK gene. Confocal microscopy qualitatively demonstrated that a smaller number of cells survived the combination therapy compared to the other three treatment groups (). Cell proliferation analysis by MTS assay further confirmed the enhanced therapeutic effect of HSV-TK gene therapy by RFH with the lowest cell viability shown in the combination therapy group versus the other treatment groups [relative cell proliferation 0.52 (0.43, 0.61) versus 0.90 (0.84, 0.96), 0.71 (0.53, 0.88), and 1 (1, 1); all p < .05] ().
Figure 1. Human ovarian cancer cells (SK-OV-3) were transfected with HSV-TK/GFP lentiviral particles. Fluorescent microscopy and quantitative assay by flow cytometry show the increasing expression of GFP in the cells over the period of 7 days, reaching the peak 5 days after gene transduction and maintaining a relatively high level over the following days.
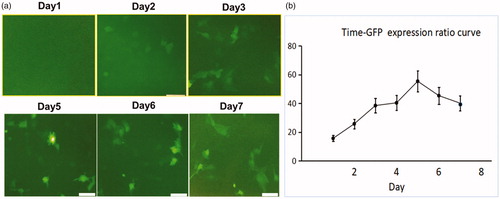
Figure 2. In vitro experiments tested the therapeutic effect of different treatments on ovarian cancer cells transfected with lentiviral mCherry/Luciferase gene. (a) Confocal microscopy showed a significantly smaller number of cells surviving the HSV-TK/GCV gene therapy combined with RFH compared to other three groups. (b) MTS assay showed the lowest cell viability in the combination therapy group (*p < .05). (c) BLI of cells further confirmed the synergistic effect of the combination therapy, shown as a significantly decreased photon signal of cells in the combination therapy group (*p < .05). (d, e) Western blotting quantification of GFP expression level in cells treated with and without RFH enhancement demonstrated an increased gene transduction with RFH (p < .05, n = 6 per group).
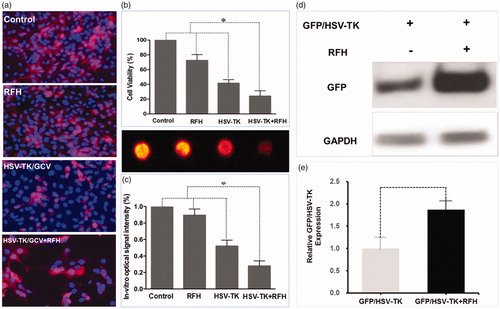
In addition, BLI of cells quantitatively demonstrated a significant decrease in relative photon signal intensity for the combination therapy group compared to the other treatment groups [0.34 (0.02, 0.66) versus 0.87 (0.64, 1.07), 0.54 (0.17, 0.91), and (1.00, 1.00), p < .05] (). Quantification of GFP expression in cells by western blotting analysis showed that GFP/HSV-TK gene expression level was increased by 1.78-fold (p < .01) with RFH compared to without ().
Creation of a rat model with orthotopic ovarian cancer
BLI analysis and histology confirmed the technical feasibility of directly inoculating SK-OV-3 cells in the rat ovary to create an orthotopic ovarian cancer model (). Within 2–3 weeks after the percutaneous intraovarian tumor cell inoculation (), tumor formation was confirmed by bioluminescence signals detected by optimal imaging () as well as H&E staining (). The regression analysis demonstrated the linear correlation between the tumor size and bioluminescence signal (y = 190.93x − 1206.3, y represents the bioluminescence signal and x represents the tumor size, R2=0.9115, p < .001) ().
Figure 3. Ovarian cancer cells were injected into the rat ovary to create an orthotopic cancer model. (a) The left ovary was exposed by aseptic surgery. (b) 0.5–1 × 107 ovarian cancer cells were injected into the ovary through a 27 G needle. (c, d) BLI detected the bioluminescence signal of the tumor 2 weeks after the cancer cell inoculation. (e, f) Gross specimen showed the orthotopic tumors in the ovary, which was further confirmed by histologic analysis (g). (h) Regression analysis revealed a significant correlation between bioluminescence and tumor size (R2=0.9115, p < .05).
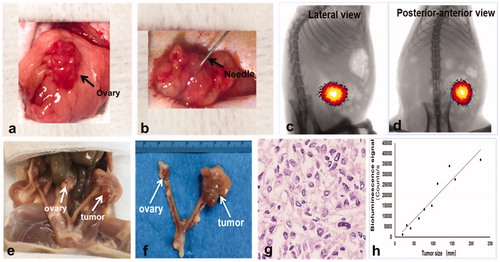
Enhanced HSV-TK gene therapy by RFH on rat orthotopic ovarian cancer
Under ultrasound imaging guidance, GFP/HSV-TK/lentivirus was precisely injected into the tumor tissue using the perfusion needle of an RF electrode (). The follow-up BLI () demonstrated a significantly lower relative photon signal intensity of tumors in the combination therapy group compared to other treatment groups [0.81 (0.52, 1.08) versus 3.60 (2.34, 4.86), 2.21 (1.71, 2.71), and 3.74 (3.19, 4.29); p < .001]. Ultrasound imaging () further confirmed that the tumor growth was significantly inhibited, as evidenced by a lower RTV, with the combination treatment compared to other treatments [0.78 (0.45, 1.11) versus 3.50 (2.67, 4.33), 2.10 (0.83, 3.37), 3.70 (1.79, 5.61), all p < .05]. Gross pathology revealed the smallest tumor size in the combination therapy group compared to other treatment groups (). The TUNEL assay demonstrated remarkably increased apoptosis in the combination therapy group in comparison to other groups [0.53 (0.29, 0.77) versus 0.02 (0.01, 0.04), 0.03 (0.01, 0.05), 0.001 (0.001, 0.02), all p < .05] (). Immunohistochemical analysis by Ki-67 staining showed suppression of cell proliferation with the combination therapy compared to other treatments [0.12 (0.05, 0.19) versus 0.49 (0.45, 0.53), 0.28 (0.21, 0.35), and 0.57(0.46, 0.68); all p < .001] (). Furthermore, the western blotting analysis of gene expression in tumor tissues () displayed a 2.21-fold increase in GFP/HSV-TK gene expression with the combination therapy, representing a 64% increase in the gene expression with intratumoral RFH compared to without (p < .05).
Figure 4. Ultrasound imaging-guided intratumoral gene therapy of rat orthotopic ovarian cancer. (a) A multipolar RF/perfusion electrode was used to deliver RFH and HSV-TK/lentiviral particles simultaneously into the tumor. (b) Under ultrasound imaging guidance, the RF/perfusion electrode was precisely positioned into the ovarian tumor.
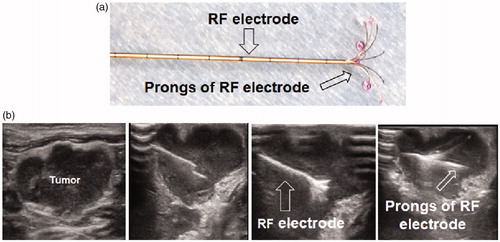
Figure 5. Four groups of nude rats with orthotopic ovarian cancer were treated with intratumoral PBS, RFH, HSV-TK/GCV gene therapy, and combination of both. Optical/X-ray images showed the bioluminescence signal of tumors. Compared to the other three groups treated with PBS, RFH, HSV-TK/GCV gene therapy alone, the combination therapy significantly retarded tumor growth, manifesting as a significant decrease in bioluminescence signals (red-yellow colors) (***p < .001).
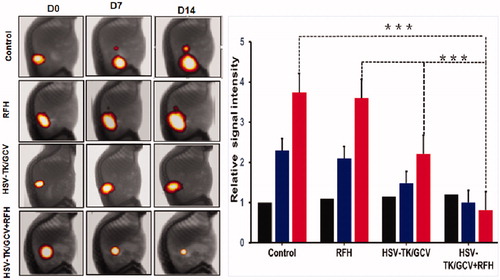
Figure 6. Ultrasound imaging follow-up of rat orthotopic ovarian tumors in four different treatment groups (PBS, RFH alone, HSV-TK/GCV alone, HSV-TK/GCV plus RFH), which demonstrated a significant decrease in tumor size for the combination therapy group compared to the other three groups (*p<.05, ***p<.001).
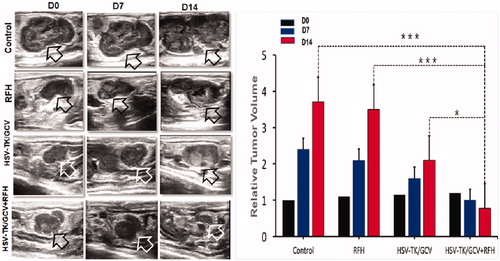
Figure 7. (a–d) Gross specimens of tumors confirmed the smallest tumor size in the combination therapy group (upper panel). (e–h) TUNEL staining for apoptosis analysis (200× magnification) showed more apoptotic cells in the combination therapy group than the other three groups. (i–l) Ki-67 staining demonstrated a substantial inhibition of cancer cell proliferation in the combination therapy group, as evidenced by the fewest number of brown-stained cells. (m, n) Western blot analysis of GFP gene expression in tumor tissues showed a greater than two-fold increase in gene expression for ovarian tumors treated with RFH enhancement compared to those treated with intratumoral lentiviral HSV-TK gene transduction alone (*p<.001).
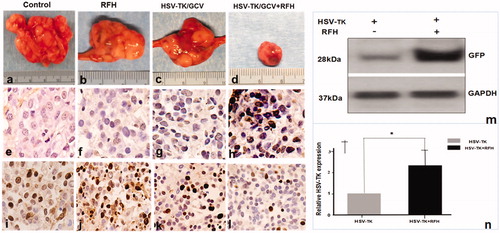
Discussion
An animal model of human ovarian cancer that can closely mimic its pathophysiologic environment offers an essential opportunity to innovate novel treatment options and assess their therapeutic efficacy. We created a human ovarian cancer cell line with stable expression of luciferase by transfecting the cells with lentiviral mCherry/luciferase gene. Using this cell line, we established the methodology of developing a rat model with orthotopic ovarian cancer that closely mimics human ovarian cancer and can be monitored by real-time, noninvasive BLI. During the longitudinal observation of the tumors with stable BLI markers, we found a strong correlation between the bioluminescent signal and tumor viability and thus employed BLI as a tool to assess therapeutic effects of various intratumoral interventions.
Most preclinical studies in ovarian cancer were performed using immunodeficient rodent models such as nude and SCID mice with subcutaneous ovarian cancer xenografts [Citation16,Citation17]. However, these subcutaneous ovarian cancer models do not resemble the pathophysiologic environment of human ovarian cancer and ignore any potentially important effects of interaction between the tumor and the host organ. Intraovarian injection of low-dose 2,4-dimethoxybenzaldehyde (DMBA) combined with repetitive gonadotropin hormone stimulation can induce primary ovarian cancer in virgin Sprague Dawley rats [Citation15]. This chemically induced ovarian cancer demonstrated the histopathology and path of dissemination to other organs similar to human ovarian cancer, but the cancer formation took 6–12 months and could not be monitored by noninvasive molecular imaging. Fu et al. reported the first orthotopic transplant model for human ovarian cancer. Histologically intact patient specimens of ovarian cancer were transplanted by microsurgical techniques under the capsule of the nude mouse ovary. The human tumors grew locally and gave rise to a patient-like metastatic pattern including the parietal peritoneum, colon, omentum, and ascites [Citation18,Citation19]. For the creation of intraperitoneal ovarian cancer model in nude mice using human ovarian cancer cell line stably expressing luciferase, Vassileva et al. transfected the cells with firefly luciferase gene plasmid [Citation20]. The clones of luciferase-positive cells were then selected by culturing the cells with the medium containing G418 sulfate. This process took more than 2 months.
In our study, ovarian cancer cells were transfected with commercially available lentiviral mCherry/luciferase gene, which integrated into the host cell genome and maintained the permanent expression of luciferase in the cells. This method of cell clone creation and isolation is more convenient and time-saving than the technique proposed by Vassileva et al. This animal model offers an additional capability of using molecular optical imaging to longitudinally follow-up the size and biological changes of the treated ovarian cancers. In addition, the rat models with molecular imaging-detectable ovarian cancers are suitable for developing the new image-guided minimally invasive interventional technique. Our study confirmed that this rat model is suitable in size to accommodate the investigation of using clinically used radiofrequency ablation (RFA) device to deliver local hyperthermia.
Using this bioluminescence-competent ovarian cancer model, we have successfully validated the feasibility and efficacy of combining RFH with intratumoral HSV-TK/GCV gene therapy. Our study provides evidence that RFH enhances the gene therapeutic effect on cancer cells in vitro and on orthotopic tumors in vivo: (i) the cell group treated by the combination therapy had the lowest cell viability and bioluminescence signal; (ii) the rat ovarian tumors treated by the combination therapy showed the greatest reduction in tumor volume and bioluminescence signal over the 14-day course of imaging follow-up; and (iii) the cells and rat ovarian tumors with GFP/HSV-TK gene transfection showed a significantly increased gene expression level following the 30-min RFH treatment compared to no RFH treatment.
Our study also demonstrated the technical feasibility of simultaneously delivering lentiviral GFP/HSV-TK gene therapy and RFH in ovarian tumors under ultrasound imaging guidance. Preclinical studies of mice and rat cancer models have confirmed the efficacy of HSV-TK/GCV gene therapy on tumors [Citation14,Citation21–23]. However, in most of these studies, the therapeutic genes were administrated via the intravenous injection. The main challenge with systemic administration is that it cannot deliver sufficiently high concentration of genetic material into tumors without risking off-target toxicity. In our study, GFP/HSV-TK/lentivirus was directly injected into rat ovarian tumors through the perfusion needle of a multifunctional RF electrode, thus establishing a technique of image-guided local delivery of high-dose therapeutic genes into the target tumor while simultaneously applying thermal energy to enhance the tumoricidal effects. This combinatorial locoregional approach is expected to reduce the amount of circulating lentivirus, thus minimizing the toxicity related to immunogenicity caused by the exogenous genes and improving the safety profile of gene therapies in patients.
Our study has limitations, including a short follow-up period of 14 days to assess therapeutic effects. This limit was set because the tumors in the control group might grow larger than 10% of the host body weight after 14 days, which was prohibited by the Institutional Animal Care and Use Committee. We also did not compare the treatment efficacy between the intratumoral and systemic administration approaches to the HSV-TK/GCV gene therapy. The acquisition efficiency of bioluminescence signal using the emission filter at 530 nm may be lower than using the filters with larger wavelengths, but the long acquisition time (8 min) compensated the decrease of signal using the filter at smaller wavelength without increasing the noise. We will try to use the filter with different emission wavelength and compare the difference in signal intensity in future studies.
In conclusion, we have successfully established a method of creating a rat model with bioluminescence imaging-detectable orthotopic ovarian cancer. This unique model allowed for confirmation of the synergistic therapeutic effect of combining RFH and intratumoral HSV-TK/GCV-mediated suicide gene therapy, as guided and monitored by noninvasive imaging modalities. This new interventional oncologic technique integrates the advances in molecular imaging, RF technology, and direct intratumoral gene therapy and demonstrates a potential for a novel locoregional therapy for unresectable ovarian cancer.
Disclosure statement
No potential conflict of interest was reported by the author(s).
Additional information
Funding
References
- Torre LA, Trabert B, DeSantis CE, et al. Ovarian cancer statistics, 2018. CA Cancer J Clin. 2018;68(4):284–296.
- Jelovac D, Armstrong DK. Recent progress in the diagnosis and treatment of ovarian cancer. CA Cancer J Clin. 2011;61(3):183–203.
- Modugno F, Edwards RP. Ovarian cancer: prevention, detection, and treatment of the disease and its recurrence. Molecular mechanisms and personalized medicine meeting report. Int J Gynecol Cancer. 2012;22(Supp 2):S45–S57.
- Dyer M, Richardson J, Robertson J, et al. NICE guidance on bevacizumab in combination with paclitaxel and carboplatin for the first-line treatment of advanced ovarian cancer. Lancet Oncol. 2013;14(8):689–690.
- Berek JS, Crum C, Friedlander M. Cancer of the ovary, fallopian tube, and peritoneum. Int J Gynaecol Obstet. 2015;131(Suppl 2):S111–S122.
- Sato T, Yanagisawa T. The engineered thymidylate kinase (TMPK)/azidothymidine (AZT)-axis offers efficient bystander cell killing effect for suicide gene therapy for cancer. Nihon Yakurigaku Zasshi Folia Pharmacologica Japonica. 2016;147(6):326–329.
- Sangro B, Mazzolini G, Ruiz M, et al. A phase I clinical trial of thymidine kinase-based gene therapy in advanced hepatocellular carcinoma. Cancer Gene Ther. 2010;17(12):837–843.
- Hung C-F, Chiang AJ, Tsai H-H, et al. Ovarian cancer gene therapy using HPV-16 pseudovirion carrying the HSV-tk gene. PLoS One. 2012;7(7):e40983.
- Zhang J-F, Wei F, Wang H-P, et al. Potent anti-tumor activity of telomerase-dependent and HSV-TK armed oncolytic adenovirus for non-small cell lung cancer in vitro and in vivo. J Exp Clin Cancer Res. 2010;29(1):52.
- Kurdow R, Schniewind B, Boehle AS, et al. Resistance developing after long-term ganciclovir prodrug treatment in a preclinical model of NSCLC. Anticancer Res. 2004;24(2B):827–831.
- Ayen A, Jimenez Martinez Y, Marchal JA, et al. Recent progress in gene therapy for ovarian cancer. Int J Mol Sci. 2018;19:pii: E1930.
- Wang J, Shi Y, Bai Z, et al. Radiofrequency hyperthermia-enhanced herpes simplex virus-thymidine kinase/ganciclovir direct intratumoral gene therapy of hepatocellular carcinoma. Int J Hyperth. 2017;33:170–177.
- Ji J, Weng Q, Zhang F, et al. Non-small-cell lung cancer: feasibility of intratumoral radiofrequency hyperthermia-enhanced herpes simplex virus thymidine kinase gene therapy. Radiology. 2018;288(2):612–620.
- Shi Y, Wang J, Bai Z, et al. Radiofrequency hyperthermia-enhanced herpes simplex virus-thymidine kinase/ganciclovir direct intratumoral gene therapy of esophageal squamous cancers. Am J Cancer Res. 2016;6(9):2054–2063.
- Stewart SL, Querec TD, Ochman AR, et al. Characterization of a carcinogenesis rat model of ovarian preneoplasia and neoplasia. Cancer Res. 2004;64(22):8177–8183.
- Chen MW, Yang ST, Chien MH, et al. The STAT3-miRNA-92-Wnt signaling pathway regulates spheroid formation and malignant progression in ovarian cancer. Cancer Res. 2017;77(8):1955–1967.
- Hernandez L, Kim MK, Lyle LT, et al. Characterization of ovarian cancer cell lines as in vivo models for preclinical studies. Gynecol Oncol. 2016;142(2):332–340.
- Fu X, Hoffman RM. Human ovarian carcinoma metastatic models constructed in nude mice by orthotopic transplantation of histologically-intact patient specimens. Anticancer Res. 1993;13(2):283–286.
- Kiguchi K, Kubota T, Aoki D, et al. A patient-like orthotopic implantation nude mouse model of highly metastatic human ovarian cancer. Clin Exp Metastasis. 1998;16(8):751–756.
- Vassileva V, Moriyama EH, De Souza R, et al. Efficacy assessment of sustained intraperitoneal paclitaxel therapy in a murine model of ovarian cancer using bioluminescent imaging. Br J Cancer. 2008;99(12):2037–2043.
- Singh S, Cunningham C, Buchanan A, et al. Toxicity assessment of intratumoral injection of the herpes simplex type I thymidine kinase gene delivered by retrovirus in patients with refractory cancer. Mol Ther. 2001;4(2):157–160.
- Hasenburg A, Fischer D, Tong XW, et al. Histologic and immunohistochemical analysis of tissue response to adenovirus-mediated herpes simplex thymidine kinase gene therapy of ovarian cancer. Int J Gynecol Cancer. 2002;12(1):66–73.
- Alvarez Jg-N RD, Wang M, Barnes MN, et al. Adenoviral-mediated suicide gene therapy for ovarian cancer. Mol Ther. 2000;2(5):P524–530.