Abstract
Purpose
To evaluate whether ‘invisible’ liver tumors on CT can be treated by stereotactic radiofrequency ablation (SRFA) using fusion of pre-ablation MRI.
Methods
In this retrospective case–control study, 60 patients (14 with Hepatocellular carcinoma (HCC) and 46 with metastatic liver tumors) with CT ‘invisible’ lesions underwent SRFA using MRI-fusion between June 2005 and June 2018 (‘fusion group’). For comparison, 60 patients who underwent SRFA without image fusion were selected using nearest neighbor propensity score matching (‘control group’). Endpoints consisted of local tumor control, safety, overall and disease-free survival.
Results
Major complications occurred in 6/69 ablations (8.7%) in the fusion group and in 6/89 ablations (6.7%) in the control group (p = 0.434). Primary technical efficacy rate (i.e., successful initial ablation) was 96.6% (28/29) for HCC and 97.9% (166/170) for metastatic disease in the fusion group and 100% (33/33) and 93.3% (184/194) in the control group, respectively (p = 0.468 and 0.064). Local recurrence (LR) was observed in 1/29 (3.5%) HCCs and in 6/170 metastases (4.0%) in the fusion group and 1/33 (3.0%) and 21/196 (10.7%) in the control group, respectively. The LR rate of metastasis in the control group was significantly higher (p = 0.007), although differences in OS and DFS did not reach statistical significance.
Conclusions
Image fusion using pre-procedural MRI allows for ablation of CT-‘invisible’ liver tumors that are otherwise untreatable. Moreover, local oncological control was higher in metastatic liver tumors versus matched controls which suggests it could be useful tool for all stereotactic radiofrequency ablation procedures.
Introduction
Adequate visualization of target tumors is fundamental to image-guided interventions including percutaneous tumor ablation. Over the past few years, advances in magnetic resonance imaging (MRI) have brought about improved definition of primary and metastatic liver tumors [Citation1,Citation2]. More specifically, sensitivity levels of contrast-enhanced MRI exceed contrast enhanced CT (CE-CT) for the detection of hepatocellular carcinoma (HCC) (84–88.5 versus 69–78.8%) [Citation3,Citation4] and metastases (86.9–100% versus 80.2–98.0%).
Fusion imaging has been developed to exploit the advantages of multiple imaging modalities and is routinely used for hybrid imaging technique such as PET-CT. More recently, the use in interventional oncological treatments has gained interest for example ultrasound (US)-guided thermal ablations with electromagnetic tracking [Citation5,Citation6].
Stereotaxy (derived from the Greek meaning ‘solid arrangement’) allows for the planning of complex trajectories using three-dimensional imaging datasets with precise transformation into real patients using a Cartesian coordinate system [Citation7]. Where target tumors are poorly visualized on CT, the navigation systems’ software can fuse MR images with planning CT data to enable precise trajectory planning and ablation zone coverage including an appropriate treatment margin.
The purpose of the present study is to evaluate whether image fusion using pre-ablation MR images is feasible to treat primary and secondary liver tumors that are ‘invisible’ on planning CT. The impact of image fusion on oncological outcome will also be evaluated.
Materials and methods
Study cohort
The local institutional review board approved this retrospective single-center study and all included patients provided their informed consent. Each case was reviewed, and the treatment plan approved by consensus in multidisciplinary tumor board meetings. Nine hundred ninety-one consecutive patients were treated by SRFA between June 2003 and June 2018. Eighty-three patients with initial palliative intention to treat and those with benign liver tumors were excluded (). Sixty sequential patients were treated in 69 ablation sessions, in which image fusion was required in the planning process (defined as the ‘fusion group’) due to ‘invisible’ lesions in CT. For comparison, 60 patients with clearly visible lesions on CT were treated in 89 SRFA sessions without the use of image fusion (defined as the ‘control group’). Patients were selected by nearest neighbor propensity score matching using the R package ‘MatchIt’ using age, sex, tumor type, number, size, and liver function (Child-Pugh Score) as matching variables. shows the baseline characteristics of both the study and control groups.
Table 1. Patient characteristics of 60 patients with 199 liver nodules undergoing 69 SRFA sessions with image fusion and of 60 patients with 229 liver nodules in 89 sessions without image fusion (control group).
Exclusion criteria for SRFA comprised a platelet count of < 50,000/mm3, prothrombin activity < 50%, and a tumor location close to (<10 mm) the central bile ducts. Tumor diagnosis was confirmed by classic tumor enhancement patterns on multiphasic contrast MRI or CT and in inconclusive cases validated by biopsy.
Image fusion used for planning
In the fusion group, pre-ablation MR datasets (sequence with best target visibility, acquired the day before RFA) were fused with the planning CT. This was performed either due to very poor CT visibility of the target tumors on the contrast enhanced planning-CT (62 SRFA sessions) or impaired renal function (7 SRFA sessions), which precluded contrast administration. Images were superimposed on each other using the rigid body fusion software StealthMerge™ (Medtronic, Mansfield, MA) with the ‘auto-merge’ algorithm and by additional ‘point merge’ using a minimum of five clearly identifiable anatomic landmarks such as vascular branching, surgical clips or calcifications in the area/segment of interest. The result was checked by visual inspection of the borders of the liver surface in the respective segment and by correlation of anatomical landmarks in the area of interest that were not used for image-to-image registration. If the inaccuracy exceeded more than 5 mm, the ‘point merge’ was iteratively refined. The approximate time required for ‘auto-merge’ is 2 min and 5 min for ‘point merge’. An example fusion is shown in .
Figure 2. Example images of fused images by superimposition on each other by StealthMergeTM in a patient with impaired renal function. (A, B) Showing fused images of the non-contrast enhanced planning CT with MR images of the previous day. (C, D) Corresponding fused images with 50:50 overlay (native CT and MR) (D). (E, F) Anatomical landmarks used for check of fusion (crosshair).
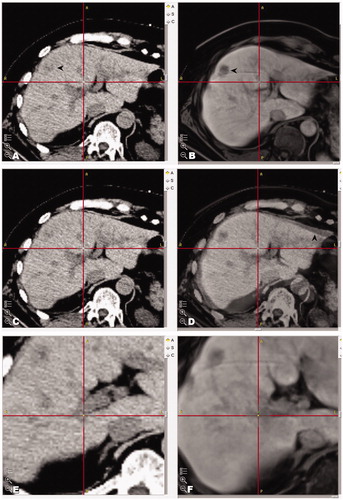
SRFA procedure
The method of SRFA has previously been reported in detail [Citation8–10]. Briefly, the key aspects are as follows:
Preparation stage: The procedure is performed under general anesthesia using muscle paralysis and immobilization facilitated by a single (Bluebag, Medical Intelligence Schwabmünchen, Germany) or double vacuum fixation technique (BodyFix, Medical Intelligence Schwabmünchen, Germany). About 10–15 registration markers (Beekley Spots, Beekley Corporation, Bristol, CT), broadly attached to the skin, are used for image-to-patient registration.
Planning stage: First, a contrast enhanced CT (CECT) (Siemens SOMATOM Sensation Open, sliding gantry with 82 cm diameter, Siemens AG, Erlangen, Germany) is acquired with 3 mm slice thickness in arterial and portal-venous phase and transferred to an optical navigation system (Stealth Station Treon plus, Medtronic Inc., Louisville, KY). In the fusion group of the present study, pre-ablation MR datasets were then fused with planning CT. One or multiple trajectories are then planned with multiplanar and 3D reconstructed images, using the navigation system’s software.
Execution stage – coaxial needle placement: To eliminate respiratory motion, temporary disconnections of the endotracheal tube are performed during the planning CT, during each stereotactic needle placement and final control CT. After registration, a quick registration accuracy check and sterile draping, the ATLAS aiming device (Elekta PSC Medical Intelligence Inc., Schwabmuenchen, Germany) facilitates navigated trajectory alignment. 15G x 17.2 cm coaxial needles (Bard Inc., Covington, GA) are then introduced through the aiming device without real-time imaging. The coaxial needles serve as guides for the RF probes. For verification of correct needle placement, an unenhanced CT is performed and fused with the planning CT using the navigation system’s image 3D registration algorithm. A 16G biopsy sample is obtained via one of the coaxial needles in patients with lack of histological confirmation.
Execution stage – ablation: A maximum of three 17G RF-electrodes (Cool-tip, Medtronic, Mansfield, MA, 25cm in length with 3 cm exposure) are introduced through the coaxial needles for serial tumor ablation. RF ablation is performed using the unipolar Cool-tip_RF generator (Cool-tip, Medtronic, Mansfield, MA) including the Cool-tip_RF switching controller. The standard ablation time for three electrodes is 16 minutes, although when an increase of impedance (the so-called ‘roll-off effect’) is encountered, the ablation process is considered to be finished. To prevent bleeding and potential tumor seeding, track ablation is carried out prior to repositioning and during final removal. For cases which required >3 ablation zones, RFA probes are removed after ablation is finished and re-inserted into the other co-axial needles sequentially until the process is complete.
Confirmation stage: After ablation, a completion CECT in the arterial and portal venous phase is fused with the planning CT for verification of ablation zone coverage and for assessment of complications.
An example of a typical SRFA procedure which can be completed in a morning or afternoon session is shown in .
Figure 3. Case of a 71-year old male with two max. 2.4 cm large colorectal cancer liver metastases. (A) Unenhanced CT-image of an ‘invisible’ colorectal liver metastasis (black arrowhead). (B) Corresponding MR image (diffusion weighted sequence). (C) Maximum intensity projection (MIP) of the control CT with 7 coaxial needles in position. (D) Fused images of the control and planning CTs showing inserted coaxial needles and superposition of planned paths. (E, F) The black arrowheads illustrate the ablation zones on MR images at 3 months after SRFA without evidence of local recurrence.
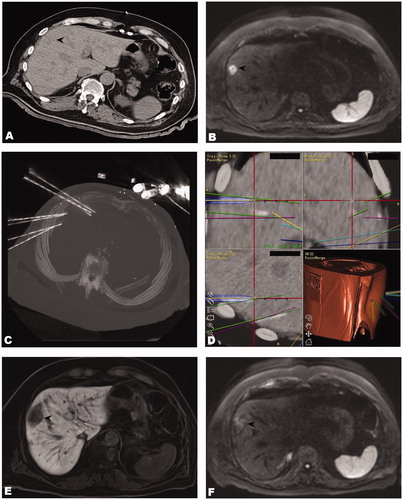
Due to the advantages of navigation, hydrodissection as a proven means of diaphragmatic separation could be avoided in all cases.
Endpoints
Technical success was defined as sufficiently accurate coaxial needle placement (deviation < 1 cm at all needle tips) according to predefined plans. Technical efficacy and local recurrence rate (LR) were determined using follow-up contrast-enhanced CT or MR scans performed at 1 month and 3-month intervals following RF ablation, whereby images were evaluated by two experienced abdominal radiologists in consensus (radiologist 1 with 20 years’ experience, and radiologist 2 with 11 years’ experience). Primary technical efficacy rate was evaluated for each tumor, defined as the absence of residual disease on 1-month follow-up CT. Secondary technical efficacy rate was defined as tumors that required repeat ablation due to residual tumor. Appearance of new nodules within or directly adjacent to the ablation zone or initial tumor defined LR. New nodules distant to the ablation zone and/or to initial tumor location were defined as distant tumor recurrence. Complications were defined according to the Society of Interventional Radiology (SIR) Standards of Practice Committee classification [Citation11]. Disease-free (DFS) and overall survival (OS) were calculated from the date of initial SRFA to the date of death attributable to malignancy or other causes (i.e., event) or to the most recent follow-up visit (i.e., censoring).
Statistics
Statistical analysis was performed using IBM SPSS version 24 (IBM, Armonk, NY). Data were expressed as total numbers, median and range. OS and DFS were evaluated using the Kaplan Meier method and group comparisons using the log-rank test. Differences between categorical variables were evaluated with the X2 test, and between independent continuous variables using the Mann–Whitney U test. A p value < 0.05 was considered as statistically significant.
Results
Patient characteristics
In the fusion group, 14 patients with HCC (2 females, 12 males) and 46 patients with metastatic liver tumors (17 females, 29 males) had a median age of 64.5 (45–77) and 62.0 (7–78) years, respectively (). The majority (58.7%, 27/46) of metastatic liver tumors originated from colorectal cancer. The median tumor size was 2.9 (0.5–11) for 29 HCC nodules and 2.5 cm (0.8–9) for 170 metastatic tumor nodules. A median of 1 HCC (1–5) and 2 metastases (1–12) were treated per ablation session (69 sessions in total). At baseline, 6 (42.8%) patients with HCC and 16 (34.8%) patients with metastases had a solitary liver tumor, 4 (28.6%) and 10 (21.7%) had two tumors, and 4 (28.6%) and 20 (43.5%) patients had three or more tumors. 12 (85.7%) patients were cirrhotic (9 (75%) Child–Pugh A, 3 (25%) Child–Pugh B). No (0%) patients with HCC and 24 (52.2%) with metastasis underwent chemotherapy, 2 patients with HCC (14.3%) and 13 (28.3%) with metastases underwent hepatic resection, 2 (14.3%) with HCC and 3 (6.5%) patients with metastases underwent conventional RF ablation prior to SRFA. Two patients with HCC underwent transcatheter arterial chemoembolization (TACE).
In the control group, 14 patients with HCC (4 females, 10 males) and 46 patients with metastatic liver tumors (17 females, 29 males) with a median age of 67.5 (53–83) and 62.0 (27–79) years respectively, underwent SRFA without image fusion. The median size of 33 HCC and 196 metastatic nodules was 2.9 (0.5–11) and 2.5 cm (0.8–9), respectively.
Per ablation session, RFA probes were inserted through 1–20 (median 3) coaxial needles.
Periprocedural complications
In the fusion group, overall major complication rate was 8.7% (6 of 69 ablations), with 7.1% (1 of 14 ablations) for HCC patients and 9.1% (5 of 55 ablations) in patients with metastatic disease. Three patients developed perihepatic hemorrhage which was managed by angiographic coiling. Two pneumothoraces and one massive pleural effusion required treatment with chest tubes. Five of six major complications were successfully treated by the interventional radiologist in the same anesthetic session. The median hospital stay after SRFA was 4.5 days, ranging from 2 to 35 days.
In the control group, overall major complication rate was 6.7% (6 of 89 SRFA sessions) for HCC patients 5.9% (1 of 17 ablations) and 6.9% (5 of 72 ablations) for patients with metastatic disease. Complications comprised a liver abscess, intra-/perihepatic hemorrhage (3 patients), a pneumothorax and a pleural effusion. There was no statistically significant difference in overall major complication rate (p = 0.434) including when stratified for tumor type (p = 0.708 for HCC, p = 0.450 for metastases).
The number of inserted coaxial needles was significantly higher in those patients who experienced major bleedings requiring embolization, with median 14.5 versus 7 needles (p = 0.008). No perioperative deaths occurred in either the fusion or control groups.
Technical efficacy
In the fusion group, 194/199 tumors were successfully ablated at initial SRFA (97.5% primary technical efficacy rate). Based on tumor type, primary technical efficacy rates were 96.6% (28/29) for HCC and 97.9% (166/170) for metastatic disease. No unsuccessfully ablated tumors were re-treated.
In the control group, 217/229 tumors were successfully ablated at initial SRFA (primary technical efficacy 94.8%). Based on tumor type, primary technical efficacy rates were 100% (33/33) for HCC and 93.3% (184/196) for metastatic disease. Ten out of 12 tumors were retreated, resulting in a secondary technical efficacy of 99.1% (227/229).
There was no significant difference of overall primary and secondary efficacy rates versus the fusion group (p = 0.116, p = 0.171). There was also no significant difference based on tumor type (p = 0.468 and p = 0.468 for HCCs and p = 0.064 and p = 0.278 for metastases, respectively). Detailed information is shown in and .
Table 2. Cases with local recurrence after SRFA.
Table 3. Tumor-based therapy success rates.
Tumor recurrence
LR in the fusion group developed in 7 of 199 tumors (3.5%, , median imaging follow-up 10 months, range 3–160 months). Based on tumor type, LR was found in 1 of 29 HCCs (3.5%), and 6 of 170 metastases (4.0%). Distant hepatic recurrence was found in 22 patients (32.8%), and extrahepatic metastasis in 11 patients (16.4%).
LR in the control group was 9.6% (23/229 tumors) which was significantly higher than in the fusion group (p = 0.009). Based on tumor type, LR rate was 3.0% (1/33) in HCC and 10.7% (21/196) for metastasis. There was a significant difference versus the study group for metastasis (p = 0.007) but not for HCC (p = 0.721).
Overall and disease-free survival
In the fusion group, OS rates at 1-, 3-, and 5- years from the date of the first SRFA were 92.3%, 70.3%, and 44.0%, respectively for HCC with a median OS of 42.7 months (95% CI 37.2–48.3) ( and ). OS rates at 1-, 3- and 5- years were 94.6%, 53.2% and 36.5% for metastatic disease with a median OS of 46.0 months (95% CI 27.2–70.5).
Figure 5. Disease-free survival after initial SRFA of patients with HCC (A) and metastatic disease (B).
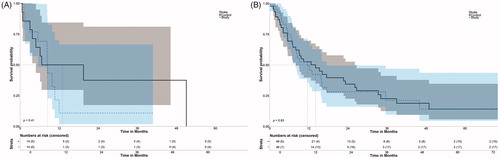
DFS rates for HCC at 1- and 3- years were 20.0% and 20.0%, respectively, with a median DFS of 8.41 months (95% CI 5.5–8.6). Among patients with metastatic disease, DFS rates at 1-, 3- and 5 years were 49.3%, 21.1% and 14.1% with a median DFS of 11.6 months (95% CI 7.1–17.4).
In the control group, OS for HCC at 1-, 3- and 5- years was 76.2%, 40% and 0% (median 25.3 months, 10.5–40.1), respectively, for HCC and 83.9%, 48.7%, 32.8% (median 32.4 months, 18.2 and 46.6%), respectively, for metastatic disease. DFS rates at 1-, and 3- and 5- years were 50%, 37.5% and 0% (median 13.0, 95% CI 4.8–24.4) and 52.6%, 22.6% and 14.1% (median 14.1, 7.98–26.1), respectively.
There was no significant difference in OS for HCC and metastasis (fusion versus control group), whereby p = 0.24 and p = 0.60, respectively. There was also no significant difference in DFS, whereby p = 0.41 and p = 0.83, respectively.
Discussion
The major finding of our study is that image fusion of MRI to CT datasets in the planning stage of SRFA is feasible and can improve local oncological outcome for liver metastases in comparison with a matched control cohort of CT visible lesions. In contrast, no such differences were found for HCC which is probably due to greater visibility of the tumor border for HCC versus metastases whereby treatment of the entire tumor with a sufficient treatment margin is crucial for good local control [Citation12]. The observation that LR rate of metastatic disease is significantly lower in the fusion group versus the control group (3.5% versus 10.7%, p = 0.007) highlights that despite a possible fusion error, better visualization of elusive metastatic tumor borders results in better local tumor control.
Our LR rate of 4% for metastatic liver tumors compares favorably with the conventional RF ablation literature which quotes rates of 8.7–48% for small colorectal liver metastasis [Citation13–15], 11–25% in small breast cancer liver metastasis [Citation16] and 4.2–50% in small- and medium-sized neuroendocrine tumor liver metastasis [Citation17–19]. Furthermore, our results are similar to hepatic resection whereby a meta-analysis conducted by Amerongen et al. [Citation13] quoted LR rates of 2.1–13%.
Our primary technical efficacy rates of 96.6% for HCC and 97.9% for metastatic disease were not significantly different to the matched controls and are comparable to the conventional RF ablation literature for tumors <3 cm [Citation20–22].
We had a major complication rate of 7.1% for patients with HCC and 9.1% for patients with metastatic disease whereby no significant difference was found versus matched controls. Hence, fusion did not negatively affect safety in our study. However, these rates are toward the upper end reported in the conventional RF ablation literature which ranges from 2.6 to 19.4% for small HCC [Citation23,Citation24], 1.3 to 12% in small colorectal liver metastasis [Citation13–Citation15], 0 to 1.1% in small breast cancer liver metastasis [Citation16] and 1 to 12% in small and medium neuroendocrine tumor liver metastasis [Citation17–Citation19]. This relatively high complication rates may be explained by the use of multiple coaxial needles, each of which confers risk.
Our median OS of 42.7 months in HCC patients and 46.0 months in metastatic disease with a median respective DFS of 8.41 and 11.6 months is comparable with the reported literature whereby a median OS of 42–93.5 months has been reported for small HCC [Citation23,Citation24], 27–53.2 months for small colorectal liver metastasis [Citation13–Citation15], 11–60 months for small breast cancer liver metastasis [Citation16] and 66–123 months for small and medium neuroendocrine tumor liver metastasis [Citation17–Citation19].
In principle, image fusion combines the benefits of two separate imaging modalities without their drawbacks [Citation25,Citation26], for example the real time guidance offered by ultrasound with the high visibility conferred by MRI. When applied to SRFA, image fusion of MRI and CT data is particularly appealing in case of poor CT visibility, and easily implemented by the navigation system’s software. CT is the workhorse of image guided tumor ablation because of its rapid, cheap and easy acquisitions with high levels of diagnostic accuracy for common complications such as pneumothorax and active hemorrhage [Citation27], although lower contrast resolution means tumors may be poorly illustrated [Citation3,Citation4]. While MRI is often superior for tumor detection, localization and definition [Citation3,Citation4,Citation28], interventional MRI is expensive, time consuming and requires dedicated setup with safety implications [Citation29]. In this way, fusion may be used to deliver SRFA using benefits of interventional MRI, without the added complexity.
Limitations
This study is limited by its retrospective design, which did not allow for a power calculation. In particular, while there were differences in overall survival between the fusion and control groups (median OS 42.7 months versus 25.3 for HCC and 46.0 versus 32.4 months for metastatic disease), these differences did not reach statistical significance which may have been the case with a dedicated prospective, powered study. In addition, the retrospective design also leads to heterogeneity in other treatment regimens, which can confound results. The matching process itself has some limitations because the specific and complex anatomical features of each tumor of all included patients are difficult to reliably identify in the matching process. Finally, our study was conducted at a single center with high levels of experience with SRFA which requires additional equipment. As such, our results are unlikely to be generalizable to the treatment of liver cancer by Interventional Radiologists worldwide. However, we have previously shown that inexperienced operators can achieve the same results as experienced operators with 2 months of training [Citation30].
In conclusion, image fusion with pre-procedural MRI in the planning stage of stereotactic radiofrequency ablation allows for ablation of otherwise ‘invisible’ tumors and also improves local oncological outcome for metastatic tumors versus matched controls. However, no such relationship was found for HCC and no benefit was found regarding technical efficacy, major complication rate, overall survival and disease-free survival.
Ethical approval
All procedures performed in studies involving human participants were in accordance with the ethical standards of the institutional and/or national research committee and with the 1964 Helsinki declaration and its later amendments or comparable ethical standards.
Author Contributions
PS contributed to study concepts, study design, data acquisition, data analysis, statistical analysis and manuscript preparation and editing. EJ and BH contributed to manuscript editing and review. DP, GL, PW and GE contributed to data acquisition and manuscript review. BR contributed to study concepts, study design, data acquisition, data analysis and manuscript review.
Disclosure statement
No potential conflict of interest was reported by the author(s).
Informed consent
Informed consent was obtained from all individual participants included in the study.
Consent for publication was obtained for every individual person’s data included in the study.
References
- Samanci C, Sobhani F, Ucbilek E, et al. Magnetic resonance imaging in diagnosis and monitoring of hepatocellular carcinoma in liver transplantation: a comprehensive review. Ann Transplant. 2016;21:68–76.
- Choi SH, Lee JM, Yu NC, et al. Hepatocellular carcinoma in liver transplantation candidates: detection with gadobenate dimeglumine-enhanced MRI. Ajr. 2008;191(2):529–536.
- Di Martino M, Marin D, Guerrisi A, et al. Intraindividual comparison of gadoxetate disodium-enhanced MR imaging and 64-section multidetector CT in the detection of hepatocellular carcinoma in patients with cirrhosis. Radiology. 2010;256(3):806–816.
- Akai H, Kiryu S, Matsuda I, et al. Detection of hepatocellular carcinoma by Gd-EOB-DTPA-enhanced liver MRI: comparison with triple phase 64 detector row helical CT. Eur J Radiol. 2011;80(2):310–315.
- Minami Y, Minami T, Hagiwara S, et al. Ultrasound–ultrasound image overlay fusion improves real-time control of radiofrequency ablation margin in the treatment of hepatocellular carcinoma. Eur Radiol. 2018;28(5):1986–1993.
- Maybody M, Stevenson C, Solomon SB. Overview of navigation systems in image-guided interventions. Tech Vasc Interv Radiol. 2013;16(3):136–143.
- Bale R, Widmann G, Stoffner DI. Stereotaxy: breaking the limits of current radiofrequency ablation techniques. Eur J Radiol. 2010;75(1):32–36.
- Bale R, Widmann G, Haidu M. Stereotactic radiofrequency ablation. Cardiovasc Intervent Radiol. 2011;34(4):852–856.
- Bale R, Widmann G, Schullian P, et al. Percutaneous stereotactic radiofrequency ablation of colorectal liver metastases. Eur Radiol. 2012;22(4):930–937.
- Schullian P, Johnston EW, Putzer D, et al. Stereotactic radiofrequency ablation of subcardiac hepatocellular carcinoma: a case-control study. Int J Hyperthermia. 2019;36(1):876–885.
- Omary RA, Bettmann MA, Cardella JF, Society of Interventional Radiology Standards of Practice Committee, et al. Quality improvement guidelines for the reporting and archiving of interventional radiology procedures. J Vasc Interv Radiol. 2003;14 (9 Pt 2):S293–S295.
- Laimer G, Schullian P, Jaschke N, et al. Minimal ablative margin (MAM) assessment with image fusion: an independent predictor for local tumor progression in hepatocellular carcinoma after stereotactic radiofrequency ablation. Eur Radiol. 2020;30(5):2463–2472.
- van Amerongen MJ, Jenniskens SFM, van den Boezem PB, et al. Radiofrequency ablation compared to surgical resection for curative treatment of patients with colorectal liver metastases – a meta-analysis. HPB (Oxford). 2017;19(9):749–756.
- Solbiati L, Ahmed M, Cova L, et al. Small liver colorectal metastases treated with percutaneous radiofrequency ablation: local response rate and long-term survival with up to 10-year follow-up. Radiology. 2012;265(3):958–968.
- Shady W, Petre EN, Gonen M, et al. Percutaneous radiofrequency ablation of colorectal cancer liver metastases: factors affecting outcomes – a 10-year experience at a single center. Radiology. 2016;278(2):601–611.
- Bale R, Putzer D, Schullian P. Local treatment of breast cancer liver metastasis. Cancers (Basel). 2019;11(9):1341.
- Fairweather M, Swanson R, Wang J, et al. Management of neuroendocrine tumor liver metastases: long-term outcomes and prognostic factors from a large prospective database. Ann Surg Oncol. 2017;24(8):2319–2325.
- Mohan H, Nicholson P, Winter DC, et al. Radiofrequency ablation for neuroendocrine liver metastases: a systematic review. J Vasc Interv Radiol. 2015;26(7):935–942.e1.
- Karabulut K, Akyildiz HY, Lance C, et al. Multimodality treatment of neuroendocrine liver metastases. Surgery. 2011;150(2):316–325.
- Lencioni RA, Allgaier HP, Cioni D, et al. Small hepatocellular carcinoma in cirrhosis: randomized comparison of radio-frequency thermal ablation versus percutaneous ethanol injection. Radiology. 2003;228(1):235–240.
- Shiina S, Teratani T, Obi S, et al. A randomized controlled trial of radiofrequency ablation with ethanol injection for small hepatocellular carcinoma. Gastroenterology. 2005;129(1):122–130.
- Sotirchos VS, Petrovic LM, Gonen M, et al. Colorectal cancer liver metastases: biopsy of the ablation zone and margins can be used to predict oncologic outcome. Radiology. 2016;280(3):949–959.
- Luo W, Zhang Y, He G, et al. Effects of radiofrequency ablation versus other ablating techniques on hepatocellular carcinomas: a systematic review and meta-analysis. World J Surg Onc. 2017;15(1):126. Available at https://doi.org/10.1186/s12957-017-1196-2.
- Ng KKC, Chok KSH, Chan ACY, et al. Randomized clinical trial of hepatic resection versus radiofrequency ablation for early-stage hepatocellular carcinoma. Br J Surg. 2017;104(13):1775–1784.
- Makino Y, Imai Y, Igura T, et al. Usefulness of the extracted-overlay function in CT/MR-ultrasonography fusion imaging for radiofrequency ablation of hepatocellular carcinoma. Dig Dis. 2013;31(5–6):485–489.
- Xu ZF, Xie XY, Kuang M, et al. Percutaneous radiofrequency ablation of malignant liver tumors with ultrasound and CT fusion imaging guidance. J Clin Ultrasound. 2014;42(6):321–330.
- Garcia-Blazquez V, Vicente-Bartulos A, Olavarria-Delgado A, EBM-Connect Collaboration, et al. Accuracy of CT angiography in the diagnosis of acute gastrointestinal bleeding: systematic review and meta-analysis. Eur Radiol. 2013;23(5):1181–1190.
- Vreugdenburg TD, Ma N, Duncan JK, et al. Comparative diagnostic accuracy of hepatocyte-specific gadoxetic acid (Gd-EOB-DTPA) enhanced MR imaging and contrast enhanced CT for the detection of liver metastases: a systematic review and meta-analysis. Int J Colorectal Dis. 2016;31(11):1739–1749.
- Barkhausen J, Kahn T, Krombach GA, et al.; German Association of Chairmen in Academic Radiology (KLR). White paper: interventional MRI: current status and potential for development considering economic perspectives, part 1: general application. Rofo. 2017;189(7):611–623.
- Widmann G, Schullian P, Haidu M, et al. Stereotactic radiofrequency ablation (SRFA) of liver lesions: technique effectiveness, safety, and interoperator performance. Cardiovasc Intervent Radiol. 2012;35(3):570–580.