Abstract
Background
Hyperthermia (heating to 43 °C) activates the innate immune system and improves bladder cancer chemosensitivity.
Objective
To evaluate the tissue penetration and safety of convective hyperthermia combined with intravesical mitomycin C (MMC) pharmacokinetics in live porcine bladder models using the Combat bladder recirculation system (BRS).
Methods
Forty 60 kg-female swine were anesthetized and catheterized with a 3-way, 16 F catheter. The Combat device was used to heat the bladders to a target temperature of 43 °C with recirculating intravesical MMC at doses of 40, 80, and 120 mg. Dwell-heat time varied from 30–180 min. Rapid necropsy with immediate flash freezing of tissues, blood and urine occurred. MMC concentrations were measured by liquid chromatography tandem-mass spectrometry.
Results
The Combat BRS system was able to achieve target range temperature (42–44 °C) in 12 mins, and this temperature was maintained as long as the device was running. Two factors increased tissue penetration of MMC in the bladder: drug concentration, and the presence of heat. In the hyperthermia arm, MMC penetration saturated at 80 mg, suggesting that with heating, drug absorption may saturate and not require higher doses to achieve the maximal biological effect. Convective hyperthermia did not increase the MMC concentration in the liver, heart, kidney, spleen, lung, and lymph node tissue even at the 120 mg dose.
Conclusions
Convective bladder hyperthermia using the Combat BRS device is safe and the temperature can be maintained at 43 °C. Hyperthermia therapy may increase MMC penetration into the bladder wall but does not result in an increase of MMC levels in other organs.
Introduction
Dating back to 1891, William Coley first used hyperthermia therapy (HT) as a treatment for systemic cancer treatment by inoculating patients with a cocktail of bacterial toxins to induce a febrile immune response that results in tumor regression [Citation1]. HT has advanced significantly since Coley. HT has been utilized to treat advanced stage ovarian, fallopian tube and peritoneal cancer on a clinical trial basis [Citation2,Citation3]. Similarly, HT has been utilized for localized bladder cancer, particularly in Europe [Citation4–6].
HT for the treatment of bladder cancer (BC) is a treatment where intravesical chemotherapy is heated to the fever range (41–44 °C) [Citation7]. This is different from thermal ablation where temperatures are raised to 60–90 °C to permanently kill cancer cells [Citation7]. The aim of HT is to improve cancer cell sensitivity to therapeutic agents, cause direct toxicity, and trigger anti-cancer immune responses [Citation8–10]. Another benefit of HT is that it may increase permeability of cellular lipid-protein membrane bilayers, resulting in increased drug penetration through the cell membrane [Citation11]. This is thought to be caused by a phenomenon known as enhanced permeability and retention (EPR) effect [Citation11–13]. Further, HT has also been shown to enhance drug transport by damaging the urothelium, which increases the rate of drug uptake into the bladder wall [Citation12]. HT can also cause transient changes to the cytoskeleton of cells leading to increased microvascular permeability and opening up pores between endothelial cells [Citation12].
The main limitation in the use of HT for bladder cancer has been the inability to effectively deliver locoregional hyperthermia to the target tissue and to monitor temperatures as the treatment is given. HT has been applied to the bladder using a variety of methods including external deep regional radiofrequency devices, intravesical radiofrequency, and intravesical radiofrequency antennae high frequency ultrasound (HIFU), all three of which are large, expensive and require continual supervision. Recently, Combat Medical (Hertfordshire, UK) have developed a convective hyperthermia system to address these limitations [Citation10,Citation14]. In this study, we aimed to evaluate the safety and tissue penetration of convective hyperthermia on intravesical mitomycin C (MMC) pharmacokinetics in live porcine bladder models using the Combat bladder recirculation system (BRS) device.
Methods
All animal studies were approved by the Duke University Institutional Animal Care and Use Committee and all methods were performed in accordance with guidelines and regulations. Forty swine were allocated to receive intravesical MMC at doses ranging from 40 mg to 120 mg with dwell time of MMC and HT ranging from 30 to 180 min. For the purpose of the study, dwell time of MMC also corresponded to the duration of HT.
Animal model
Female (breed) swine weighing between 60 to 80 kg were obtained from Wesley Looper Farms (Granite Falls, NC). Animals fasted for 12 h prior to the procedure. Anesthesia was achieved using ketamine (22 mg/kg IM) and acepromazine (1.1 mg/kg IM), injected using a 19 Ga butterfly needle. Animals were then placed on heated tables and isoflurane was administered via face mask prior to intubation. Animals were then intubated with a 6.0 to 6.5 mm endotracheal tube and anesthesia maintained with 0.5–3% isoflurane via a mechanical ventilation machine. A 20 Ga IV catheter was then placed in the ear vein for fluid administration (0.9% sterile saline solution) at a rate of 30–50 ml/hr. An 18 Fr orogastric tube was then passed for drainage of any residual stomach contents. Vital status monitoring of oxygen saturation, heart rate, core body temperature at the esophagus, and respiratory rate was monitored continuously and recorded every 15 min. Jaw tone and pedal withdrawal reflex was also assessed.
Implantation of temperature monitoring devices
Hair was removed by clippers at the operation sites. Standard sterile surgical technique was used throughout the procedure. Lower abdominal laparotomy was performed to place the temperature monitoring devices around the bladder. The temperature monitoring devices consisted of the Luxtron® Fiber Optic Thermometry Probes (Lumasense Technologies, Santa Clara, CA, USA) and the Ge or Si High Temperature NTC thermistors (Adsem Inc, Mountain View, CA, USA). All probes were cleaned with 70% ethanol and gas sterilized prior to use in the animals. We placed probes at 7 locations: rectum, vagina, internal bladder, external bladder (serosa), transmural bladder (mucosa), core body temperature at the esophagus and internal iliac vein. The rectal and vagina thermistors were placed manually and sutured in place. The internal and external bladder microprobes were fixed in place using 4-0 to 5-0 sutures, sterile Dermabond tissue adhesive (Johnson & Johnson, New Brunswick, NJ, USA) or sterile fibrin glue/sealant. The internal iliac vein probe was placed by accessing the ipsilateral femoral vein with a 16 Ga vascular access catheter and sutured in placed after it was connected to a 3-way stopcock, which was used for simultaneous blood draws and temperature measurement. The abdominal incision sites were then sutured closed.
Bladder hyperthermia treatment
Bladder hyperthermia was achieved using the Combat BRS device (Combat Medical, UK). The device uses an aluminum heat exchanger and peristaltic pump to heat and recirculate chemotherapy solutions in the bladder. We used the disposable package which contained a 16 F 3-way catheter with a temperature monitoring thermistor in its tip. For the first part of the study - to evaluate if HT can be achieved and maintained in a swine bladder; the swine bladder was heated and the temperature was maintained at 43 °C ± 1 °C for 1 h. Five swine were included in this part of the study. We placed eight teardrop thermistors (dome, base, anterior, posterior, right lateral, left lateral and base of strip wall of the bladder) and eight surface mounted thermistors (1 cm distance on a custom-made Kapton flexible strip) (). For the second part of our study, intravesical mitomycin C (MMC) was administered at a concentration dose of 40 mg (60 min, 180 min), 80 mg (60 min) and 120 mg (30 min, 120 min) in 50 ml of sterile saline. On top of the experimental dose, the Combat BRS system was primed with 40 mg of MMC in 40 ml of sterile saline. The MMC was reconstituted and diluted using sterile technique in a laminar flow hood and stored in a capped 60 ml syringe in a double bag Ziploc bag transport system. After hyperthermia was completed, the swine bladder was drained of its MMC (or saline) and all disposable parts of the Combat BRS system were collected and disposed of.
Biospecimen collection
Blood and urine were collected every 15 min in this study. Urine was collected via Foley catheter and blood was collected via the iliac vein central venous catheter. At the termination of the experiment, the swine were euthanized using 1 ml/10 lbs IV Euthasol solution (sodium pentobarbital 390 mg/mL and phenytoin sodium 50 mg/mL). A rapid necropsy was immediately performed, and tissues/organs sampled included bladder, liver, spleen, kidneys, heart, lungs, obturator lymph nodes and blood. The bladder was emptied and flushed with saline, and tissue was obtained from the mucosal layer of the bladder. Tissue took around 15–30 min to obtain per swine.
Drug level monitoring
Thirty-five swine were included in this portion of the study to measure the effect of dose, dwell time and temperature on the absorption of MMC in the bladder and various organs. Three swine were control, and only received normal saline instillation with HT. Liquid chromatography (LC) tandem-mass spectrometry (MS/MS) was used to measure mitomycin C in plasma, urine and tissues. For the purpose of the analysis, 1 g of tissue was assumed to be 1 ml. The LC-MS/MS system consisted of either Shimadzu 20 A series LC and Applied Biosystems/SCIEX API 5500 QTrap MS/MS instrument. Phenomenex C18 3 × 4 mm, (AJ0-4287) guard column and Agilent ZORBAX Eclipse Plus C18 50 × 4.6 mm, 1.8 μm (959941-902) analytical column were used for all assays.
For blood, 1 part liquid sample or calibration sample (typically 50 μL), 1 part mitomycin A (MMA) in water (internal standard, 20 ng/mL), and 4 parts chloroform/isopropanol (4/1) was added and vigorously agitated (speed 4, 40 s; Fast-Prep FP120, Thermo/Savant, USA). After centrifugation at 16,000 g for 5 min at 20 °C, 1.5 parts (typically 150 μL) of chloroform (lower) layer was subjected to a gentle stream of nitrogen (30 min) and dry residue reconstituted with 20 μL methanol followed by 40 μL of 1 mM ammonium acetate in water. The sample was transferred into injection vial and centrifuged at 16,000 g for 5 min at 4 °C, and 5 μL was injected into API 5500 LC-MS/MS system. For tissues, 4 parts of MMA in water, 10 parts of chloroform/isopropanol (4/1) and five 2.5-mm zirconia/silica beads (Biospec Products Inc.) were added and further processed as in case of plasma. Sample/water (+int. std.)/organic solvent ratio was adjusted to accommodate different sample sizes and expected MMC concentration range. For urine, samples were diluted 1/10,000 or 1/100,000 with water, mixed with 3 parts of 10 ng/mL MMA in water, and 10 μL was injected into the LC-MS/MS system. Mobile phase A: 1 mM ammonium acetate, 2% acetonitrile; mobile phase B: acetonitrile. Elution gradient: 0-1 min, 1-50% B; 1-1.5 min, 50% B; 1.5-1.7 min, 50-1% B. Run time 4 min. The mass spectrometer was operated in positive mode with the following MRM transitions (m/z): 335/242 [MMC-1st], 335/131 [MMC-2nd], 364/271 [MMA-1st], 364/243 [MMA-2nd].
Data analysis
HPLC data acquisition and quantification was performed by Analyst 1.6.2 software. R 3.5.1 for Rstudio 1.1.456 was used for data aggregation and plotting of figures.
Results
Temperature measurement of the bladder and surrounding organs
The Combat BRS system was able to achieve a temperature of 43 °C in under 5 min, and this temperature was maintained as long as the device was running (). The temperature in the outer serosa of the bladder required 20 min to reach 42 °C and the vagina required 45 min to reach a temperature of 42 °C. The rectum however maintained a temperature <40 °C throughout the entire procedure.
Mitomycin C concentration
shows the median concentration of MMC in various organs and body fluids in the thirty-two swine that received MMC, with varying drug concentration, dwell time and hyperthermia. The concentration of MMC in the bladder is higher with HT and this does not seem to affect the concentration of drug in the kidney, spleen, lung, lymph nodes, liver, heart and plasma, even at concentrations as high as 120 mg (). shows the concentration of MMC in the bladder tissue stratified by dwell time, drug dosage and hyperthermia. In the control arm, the median MMC concentration in the bladder wall was 0 ng/mL (IQR 0–5). In swine with MMC dwell time of 30 and 60 min, the concentration of MMC in the bladder tissue is affected by 2 factors: drug dose and the application of hyperthermia. In the hyperthermia arm, drug penetration saturated at 80 mg dose, suggesting that with heating, drug absorption may saturate and not require higher doses to achieve the maximal biological effect. However, increasing the dwell time of the drug to 120 and 180 min appear to have a negative effect on the concentration of MMC in the bladder tissue. The bladder was visually examined for thermal damage and no damage was visualized. No local toxic effect was visualized in the swine bladder.
Figure 4. Jitter plot evaluation mitomycin C concentration in various organs at necropsy sorted by body temperature and 43 °C using the Combat BRS bladder hyperthermia device. (Each dot represents a sample of different dwell times and dosages).
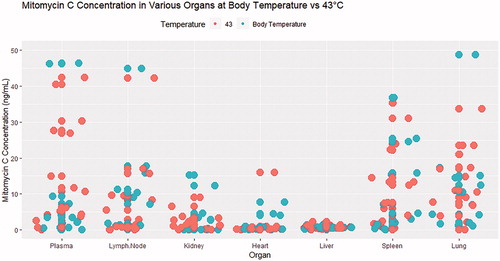
Table 1. Mitomycin C concentration in bladder wall based on dwell time, dose and hyperthermia.
Discussion
Hyperthermia therapy has been shown to improve cancer cell sensitivity to therapeutic agents, cause direct cytotoxicity, trigger anti-cancer immune responses and improve drug delivery for various chemotherapeutic agents to the bladder [Citation12,Citation13,Citation15,Citation16]. Multiple clinical trials treating patients with bladder cancer with intravesical chemotherapy and HT have shown promising results in both the adjuvant setting as well as neoadjuvant setting. However, many of these studies were conducted using radiofrequency-induced hyperthermia which is limited by cost, and operating requirements. In this paper, we aim to report the safety and tissue penetration of convective hyperthermia therapy using the Combat BRS device on MMC absorption in the bladder. We show that HT can safely be performed at a temperature of 43 °C in the bladder inner mucosa without excessive heat to the surrounding structures using the Combat BRS device; and HT increases the absorption of MMC into the bladder wall, without increasing the level of MMC in the liver, heart, kidney, spleen, lung, and lymph node tissue.
The Combat BRS bladder hyperthermia device uses an external, closed-circuit, fluid recirculation system to rapidly heat and maintain temperature within ±0.5 °C of the set temperature inside the bladder, while providing homogenous drug distribution and heating through the bladder using an aluminum heat exchanger. The ability of the Combat BRS device to consistently achieve temperatures between 41.5 °C and 43 °C across the bladder wall after 15 min of heating at a flow rate of 200 ml/min, indicates that conductive bladder hyperthermia should be sufficient to heat the entire bladder without overheating adjacent organs ().
In this study, we found that increasing the dwell time of MMC from 120 to 180 min appears to have a negative effect on the concentration of MMC in the bladder tissue. We hypothesize that this is due to two possible factors: 1) degradation of drug due to heat; 2) increase absorption and metabolism of the drug due to heat. This hypothesis is supported by the findings in , where swine with higher values of MMC in the plasma, lymph node, spleen and lung received higher dosages of intravesical MMC. In fact, swine with the highest concentration of MMC in the plasma, lymph node, spleen and lung were from the group receiving 120 mg of intravesical MMC. Nevertheless, the concentration of MMC in this cohort is still relatively low, given intravenous administration of MMC for other malignancies result in a serum concentration of 50 folds of this finding.
We utilized healthy swine that did not have any bladder pathology, given that no porcine bladder cancer model exists presently. Healthy urothelial mucosa has been shown to be less permeable than tumor tissue, hence we believe that intravesical chemotherapy with HT may actually result in an even greater increase of MMC absorption in patients with bladder cancer than demonstrated in our study [Citation17]. Our findings deferred from van Valenberg et al, where they found that MMC concentration did not differ between the cold MMC group and radiofrequency induced MMC group in normal urothelial tissue [Citation15]. This could be attributed to the usage of the Synergo system, which utilizes a 915 MHz antenna to heat the chemotherapeutic agent in the bladder, while having the drug solution constantly pumped out of the bladder and re-instilled after being cooled by the device. This finding could also be consequent to a different response in humans compared to pigs.
Our study contains several limitations that should be addressed. Firstly, although this is the largest large animal study to date evaluating the effect of intravesical chemotherapy and HT in the bladder, we are still limited by our sample size of 40 swine. Also, there appears to be a broad range within the 120 mg MMC and 30 min of HT group. Secondly, the swine were placed in supine position throughout the experiment hence small amounts of air collection did occur anteriorly inside the bladder and this could have created cold spots. This was mitigated by a modification of the Combat BRS device that now includes a tubing bubble trap. We obtained specimens from the posterior aspect of the bladder to minimize the likelihood of obtaining a specimen that was not exposed to intravesical chemotherapy. Thirdly, variability in bladder volume (and consequently bladder wall thickness) could not be eliminated despite efforts to maintain constant bladder distention. Animals fasted for 12 h prior to the procedure. Also, all swine were given a constant rate of normal saline infusion, but bladder distension could not be measured. We also did not evaluate the pH of the swine bladder, nor did we try to alkalinize the urine of the swine.
Nevertheless, we show for the first time in a swine model that we can safely achieve and maintain the bladder at a temperature of 43 °C using the Combat BRS bladder hyperthermia device and that HT up to 60 min may increase the concentration of MMC absorbed in the bladder. The maximal concentration of MMC in the bladder may be achieved using 80 mg MMC at 60 min of HT or using 120 mg MMC at 30 min of HT. Even at a concentration of 120 mg MMC, HT does not appear to increase the absorption of MMC systemically () and should be safe in humans.
Conclusions
Convective bladder hyperthermia using the Combat BRS device is safe and hyperthermia can be maintained as long as the device is left running. HT may increase MMC penetration into the bladder wall and does not result in an increase of MMC levels in the liver, heart, kidney, spleen, lung, and lymph node tissue. The use of hyperthermia may saturate drug delivery and allow for lower dosing regimens. These data support the use of the Combat BRS device to improve MMC penetration into the bladder wall, especially in a time of BCG shortage.
Disclosures statement
No potential conflict of interest was reported by the author(s).
Additional information
Funding
References
- Coley WB. II. Contribution to the knowledge of sarcoma. Ann Surg. 1891;14(3):199–220.
- Deraco M, Kusamura S, Virzi S, et al. Cytoreductive surgery and hyperthermic intraperitoneal chemotherapy as upfront therapy for advanced epithelial ovarian cancer: multi-institutional phase-II trial. Gynecol Oncol. 2011;122(2):215–220.
- Chua TC, Robertson G, Liauw W, et al. Intraoperative hyperthermic intraperitoneal chemotherapy after cytoreductive surgery in ovarian cancer peritoneal carcinomatosis: systematic review of current results. J Cancer Res Clin Oncol. 2009;135(12):1637–1645.
- Crezee H, Inman BA. The use of hyperthermia in the treatment of bladder cancer. Int J Hyperthermia. 2016;32(4):349–350.
- Owusu RA, Abern MR, Inman BA. Hyperthermia as adjunct to intravesical chemotherapy for bladder cancer. Biomed Res Int. 2013;2013:262313.
- Lammers RJ, Witjes JA, Inman BA, et al. The role of a combined regimen with intravesical chemotherapy and hyperthermia in the management of non-muscle-invasive bladder cancer: a systematic review. Eur Urol. 2011;60(1):81–93.
- Falk MH, Issels RD. Hyperthermia in oncology. Int J Hyperthermia. 2001;17(1):1–18.
- Hildebrandt B, Wust P, Ahlers O, et al. The cellular and molecular basis of hyperthermia. Crit Rev Oncol Hematol. 2002;43(1):33–56.
- Wust P, Hildebrandt B, Sreenivasa G, et al. Hyperthermia in combined treatment of cancer. Lancet Oncol. 2002;3(8):487–497.
- Longo TA, Gopalakrishna A, Tsivian M, et al. A systematic review of regional hyperthermia therapy in bladder cancer. Int J Hyperthermia. 2016;32(4):381–389.
- Maeda H, Tsukigawa K, Fang J. A retrospective 30 years after discovery of the enhanced permeability and retention effect of solid tumors: next-generation chemotherapeutics and photodynamic therapy-problems, solutions, and prospects. Microcirculation. 2016;23(3):173–182.
- van der Heijden AG, Dewhirst MW. Effects of hyperthermia in neutralising mechanisms of drug resistance in non-muscle-invasive bladder cancer. Int J Hyperthermia. 2016;32(4):434–445.
- van Valenberg FJP, Witjes JA, Aklan B, et al. Inducing intravesical hyperthermia of the ex-vivo porcine bladder wall: radiofrequency-induction versus recirculation using a custom-made device. Int J Hyperthermia. 2018;35(1):323–329.
- Tan WS, Kelly JD. Intravesical device-assisted therapies for non-muscle-invasive bladder cancer. Nat Rev Urol. 2018;15(11):667–685.
- van Valenberg FJP, van der Heijden AG, Lammers RJM, et al. Intravesical radiofrequency induced hyperthermia enhances mitomycin C accumulation in tumour tissue. Int J Hyperthermia. 2018;34(7):988–993.
- Tan WP, Longo TA, Inman BA. Heated intravesical chemotherapy: biology and clinical utility. Urol Clin North Am. 2020;47(1):55–72.
- Vats M, Mishra SK, Baghini MS, et al. Near infrared fluorescence imaging in nano-therapeutics and photo-thermal evaluation. IJMS. 2017;18(5):924.