Abstract
Purpose
In our previous study, a novel cryo-thermal therapy that could stimulate the maturation of innate immune cells to subsequently activate the CD4+Th1 cell-dominated antitumor response was developed. However, why cryo-thermal therapy can induce the maturation of innate immunity remains unknown.
Methods
In this study, western blot and ELISA were used to analyze the levels of damage-associated molecular patterns (DAMPs, including heat shock protein 70 (HSP70), calreticulin and high-mobility group box protein 1) in situ and in the peripheral blood at different times after cryo-thermal therapy or traditional radiofrequency ablation. The effects of these three DAMPs on myeloid-derived suppressor cells (MDSCs), dendritic cells (DCs) and macrophages were investigated by antibody neutralization in vitro. The phenotypic and functional changes in MDSCs, DCs and macrophages were analyzed using FACS and qRT-PCR. An anti-HSP70 antibody was injected intravenously at 6 h after cryo-thermal therapy on days 1 and 2 and mouse survival was monitored.
Results
Cryo-thermal therapy could trigger the release of DAMPs in situ and in the peripheral circulation, which could downregulate the proportion and suppressive signature of MDSCs, and promote the M1 macrophages polarization and DCs maturation. Among three DAMPs, HSP70 played the most evident role in M1 macrophage polarization. In vivo neutralization of HSP70 in the early stage of treatment could significantly decrease the survival rate of cryo-thermal therapy treated mice.
Conclusions
Local cryo-thermal therapy not only destroyed solid tumors thermally and mechanically but also induced the release of a large amount of DAMPs to effectively trigger a systemic antitumor response.
Introduction
Breast cancers, especially triple-negative breast cancer, are the greatest threat to the health and wellness of women [Citation1]. Some breast cancers have low immunogenicity and induce immune tolerance to evade immune surveillance [Citation2–4]. Recent data show that conventional cancer therapies, such as chemotherapy and radiotherapy, rely heavily on the immune response to be effective. With an increased understanding of tumor immune surveillance, immunotherapy has emerged as a promising treatment strategy for breast cancer.
Emerging evidence has raised questions related to improving the efficacy of immunotherapeutic approaches. To trigger antitumor immunity, researchers have realized that in addition to therapeutically exploiting and utilizing innate or adaptive immune cells directly, enhancing the immunogenicity of cancer cells is also necessary [Citation5,Citation6]. The immunogenicity of malignant cells has recently been acknowledged to be a critical determinant of efficacy in cancer therapy. Dying cancer cells can release or expose damage-associated molecular patterns (DAMPs) via the process of immunogenic cell death (ICD) induced by cancer treatments. ICD is characterized by the presence of major DAMPs, including calreticulin (CRT), the heat shock protein 70 (HSP70) and high-mobility group box protein 1 (HMGB1). As alarm signals detected by the innate immune system, DAMPs have the potential to activate innate immunity, which directs the subsequent development of adaptive immunity [Citation7–10]. HSP70 located in the endoplasmic reticulum can activate the innate immune response by binding to Toll-like receptors (TLRs) on dendritic cells (DCs) and macrophages [Citation11]. CRT in the endoplasmic reticulum can promote the maturation of DCs [Citation12,Citation13], and trigger tumor-specific immune responses [Citation14]. HMGB1 is typically located in the nucleus, and only a limited amount of HMGB1 exists in the cytoplasm [Citation15]. As an endogenous TLR agonist released during the middle and late stages of death and apoptosis [Citation16–18], HMGB1 can stimulate neutrophils, macrophages and monocytes to produce inflammatory cytokines [Citation19]. DAMPs bind to surface pattern recognition receptors on antigen-presenting cells (APCs) and promote the maturation of APCs and migration to the lymphoid organs [Citation20]. Generally, mature APCs express high levels of antigen-presenting major histocompatibility complex (MHC) molecules, the costimulatory molecules CD80/86 and the inflammatory cytokines IL-12 and IFNs, leading to further activation of the T cell-mediated immune response [Citation21,Citation22].
Therefore, results for immunotherapy indicate the need to study the interface between dying cancer cells and the immune system to efficiently exploit this interaction to enhance antitumor therapeutic efficacy. Another type of immunotherapy for inducing tumor cells to undergo ICD to increase immunogenic potential is emerging. Conventional cancer treatments, such as chemotherapy and radiation therapy, and other physical therapies have been studied extensively for their ability to induce ICD.
In our previous study, a novel local cryo-thermal therapy was developed to stimulate systemic antitumor immunity in animal models [Citation23]. The therapeutic effect of local cryo-thermal therapy was clearly demonstrated by using mice bearing subcutaneous 4T1 murine mammary carcinomas or experimental lung metastases of murine B16F10 melanoma, and the mice had long-term survival rates over 70% and 80%, respectively [Citation24,Citation25]. Moreover, we recently identified that cryo-thermal therapy could drive M1 macrophage polarization to remodel the host immune environment by promoting subsequent DC activation and maturation, CD4+ T cell differentiation into Th1 cells and CTLs, and cytotoxic CD8+ T cell generation, leading to effective antitumor immune memory capable of inhibiting distant tumor metastasis [Citation26]. Through the precise control of the process of cryo-thermal therapy involving rapid LN2 cooling followed by rapid RF heating, the tumor center was fully coagulated, while in the peripheral zone, tumor cells and newly formed tumor vessels were completely disrupted through the synergistic effects of thermal and mechanical stresses and blood reperfusion [Citation27]. However, whether local cryo-thermal therapy induces ICD and promotes the release of DAMPs to effectively activate the innate immune system has not been addressed.
In this study, we aimed to investigate whether cryo-thermal therapy induces the release of a large amount of DAMPs to promote APC activation and maturation. By using a subcutaneous 4T1 murine model, we tested the levels of DAMPs (such as HSP70, HMGB1 and CRT) released in situ and in the peripheral circulation after cryo-thermal therapy and investigated the roles of these DAMPs in APC activation and maturation. Our results showed dramatically increased release of HSP70, HMGB1 and CRT in situ and in the peripheral circulation after cryo-thermal therapy. These DAMPs, especially HSP70, effectively decreased the level of myeloid-derived suppressor cells (MDSCs) and impaired MDSC immunosuppressive molecules expression while fostering macrophage M1 polarization and DC maturation. Hence, cryo-thermal therapy-induced DAMP release could provoke an innate immune response, resulting in the triggering of T cell-mediated antitumor immunity, as reported in our previous studies. Our studies suggested that local cryo-thermal therapy could be a potential immunotherapeutic approach for not only destroying solid tumors thermally and mechanically but also promoting ICD, along with the release of a large amount of DAMPs, to trigger systemic antitumor immunity.
Materials and methods
Animal studies
All animal experiments were approved by the Animal Welfare Committee of Shanghai Jiao Tong University, and the experimental methods were performed in accordance with the guidelines of Shanghai Jiao Tong University Animal Care (approved by Shanghai Jiao Tong University Scientific ethics committee). Four-week-old female BALB/c mice were obtained from Shanghai SLAC Laboratory Animal Co., Ltd., China, then housed and fed sterile food with standard mice nutritional formula and sterile water in the isolated cages of 12 h light/dark cycle environment.
Animal tumor model
The murine mammary carcinoma 4T1 cell line was provided by Shanghai First People’s Hospital, China. Cells were cultured in DMEM medium in addition with 10% fetal bovine serum, together with 100 U/ml penicillin, and 100 g/ml streptomycin (Shanghai Sangon, China). 4T1 cells (5 × 105) were inoculated subcutaneously at the right femoral region of the mice (at the age of 6–8 weeks) and monitored the tumor growth every day. The tumor volumes were calculated using the formula: V (mm3) = π/6 × L (major axis) × W2 (minor axis).
The cryo-thermal treatment procedures
The cryo-thermal therapy system which developed in our laboratory was composed of liquid nitrogen for cooling and radiofrequency for heating. A probe was designed for the cryo-thermal treatment of subcutaneous tumor which was compatible with the system. The probe was designed in a concave-shaped tip of 10 mm in diameter to suitable for the size of mouse tumors, reduce the effect of contact thermal resistance and obtain a constant thermal delivery during the treatment. Twenty-one days after tumor inoculation, the average of tumor size was reached about 0.2 cm3, then mice were randomly divided into three groups: (1) tumor-bearing group without any treatment (control); (2) Traditional radiofrequency ablation (RFA) group with RF heating on primary tumor at the temperature of 50 °C for 15 min (the temperature was controlled at the bottom of tumor); (3) cryo-thermal group with the primary tumor fast freezing at the temperature of −20 °C for 5 min followed by RF heating at the temperature of 50 °C for 10 min. Before the treatment, mice were anesthetized with 1.6% pentobarbital sodium (0.5 ml/100g, i.p.) and tumor site was sanitized with alcohol and iodine tincture. All the procedures were carried out aseptically.
Western blot analysis
To collect the tumor interstitial fluid (TIF), tumor tissues were harvested in 6 and 48 h after the treatments, then wrapped with Nylon filter paper (50 μm) and centrifuged at 2000 rpm for 20 min. The serums of each group were collected on 6 h, 1 day, 2 days and 3 days after treatments. Total proteins of 30 μg quantified with BCA method were separated onto the 4–20% gradient Tris-Glycine precast gels and transferred to PVDF membranes. Blot was probed with anti-HSP70 (Cell Signaling Technology, USA), anti-HMGB1 (Abcam, UK) and anti-CRT (Abcam, UK). Each shown Western blot result was a representative from three separate experiments.
HSP70 ELISA
The concentration of HSP70 from each group serums on 6 h, day 1, day 2 and day 3 after treatments were determined through enzyme-linked immunosorbent assay (ELISA kit, Abcam) following the manufacturer’s instructions. Four biological replicates and two technical replicates were presented.
Isolation of splenic MDSC, macrophage and DCs
Spleens were harvested from the untreated mice on day 24 after tumor cell inoculation. The splenocytes were separated by Gentle MACSD issociator (MiltnyiBiotec, Germany). Following the removal of RBCs and tissue debris, MDSC cells were isolated by using MDSC isolation microbreads kit (MiltnyiBiotec, Germany) on MS columns. CD68+ (PE) macrophages were isolated through PE selection kit (Stem cell, USA). DCs were isolated through CD11c+ selection kit (Stem cell, USA). The isolated cells with a purity of >90% were used for experiments.
Flow cytometry analysis
Mice were sacrificed on day 2 after the treatments and the spleens were collected to prepare for single-cell suspension of splenocytes through GentleMACS™ dissociator (Miltenyi Biotec). Then, the red blood cells were removed using erythrocyte-lysing reagent containing 0.15 M NH4Cl, 1.0 M KHCO3 and 0.1 mM Na2·EDTA. The splenocytes were stained with fluorescent labeled antibodies for 30 min at 4 °C. The staining antibodies including FITC anti-mouse CD11b, PE anti-mouse Gr-1, AF488 anti-mouse CD11c, APC anti-mouse F4/80, Percp/cy5.5 anti-mouse CD86 and PE anti-mouse MHC II were all purchased from Biolegend (USA). Data were acquired on FACS Aria II flow cytometer (BD, USA).
RNA isolation and real-time PCR
Total RNA extracted from isolated MDSCs, CD68+ macrophages and CD11c+ DCs from different group using TRIzol Reagent (TaKaRa, Dalian, China), and obtained the cDNA using PrimerScript RT reagent kit (TaKaRa, Dalian, China). The SYBR Premix Ex Taq (TaKaRa) and cDNA samples were amplified in 384-well plates. Quantitative real-time PCR (qRT-PCR) was performed on ABI 7900HT sequence detection system and SDS software (Applied Biosystems, Foster City, CA, USA). Relative expression levels of mRNA were normalized to glyceraldehyde 3-phosphate determined by the Ct value and assessed using relative quantification (ΔΔCt method).
In vitro DAMPs neutralization
MDSCs, macrophages and DCs isolated from the spleen of control (23 days after tumor cell inoculation) or cryo-thermal treated mice (2 days after treatment) were cultured with 500 μl DMEM which contain 20% serums from normal, tumor-bearing and cryo-thermal-treated mice for 24 h in vitro with the addition of anti-HSP70 (15 μl), anti-CRT (15 μl) or anti-HMGB1 (15 μg antibodies for each well). The cells were cultured in 24-pore plate for 1 million cells per well. Three parallel duplications were set for each group.
In vivo HSP70 neutralization
The 4T1 tumor-bearing mice were built as described above and were divided into three groups randomly, including tumor-bearing control, cryo-thermal therapy and cryo-thermal therapy following anti-HSP70 antibody neutralization. The mice in cryo-thermal therapy following anti-HSP70 antibody neutralization group were injected with 200 μl anti-HSP70 antibody (i.v.) at 6 h, day 1 and day 2 after cryo-thermal therapy and survival times of mice in different group were observed (6 mice in each group).
Statistical analysis
The statistics were analyzed using one-way ANOVA through Graph Pad Prism 6. Results were shown as mean ± SD. *p < .05, **p < .01 and ***p < .001.
Results
Cryo-thermal therapy enhanced the release of DAMPs in situ and in the peripheral circulation
Our previous studies indicated that cryo-thermal therapy could better augment tumor necrosis in situ than could RF ablation [Citation24]. DAMP release after tumor therapy participates in the activation of the antitumor immune response [Citation10,Citation28]. To identify the roles of DAMPs in the innate immunity elicited by cryo-thermal therapy, we first analyzed the levels of HSP70, HMGB1 and CRT released into the TIF after treatments. As shown in , cryo-thermal therapy triggered a large amount of CRT and HSP70 release in tumors at 6 h after treatment, and the levels of CRT and HSP70 release were decreased at 48 h. In contrast, after RFA, the levels of CRT and HSP70 release were much lower than those at 6 h after cryo-thermal therapy, but the levels of CRT and HSP70 release at 48 h were increased to levels similar to those after cryo-thermal therapy. Moreover, both cryo-thermal and RFA therapies induced the same level of HMGB1 release at 48 h after treatment ().
Figure 1. The levels of DAMPs in situ and peripheral circulation after cryo-thermal therapy. (A) The levels of HSP70, CRT and HMGB1 released in interstitial fluid at 6 h and 48 h after RFA and cryo-thermal treatments were analyzed by western blot; (B) The levels of CRT and HMGB1 in serum at 6, 24, 48 and 72 h after RFA and cryo-thermal treatments were analyzed by western blot; (C) The HSP70 concentration in serum of three groups from 6 to 72 h after treatments were measured by using ELISA; (D) Representative HSP70 concentration in serum from three groups at 48 h after treatments. Data were shown as mean ± SD. *p < .05, **p < .01, ***p < .001 by one-way ANOVA.
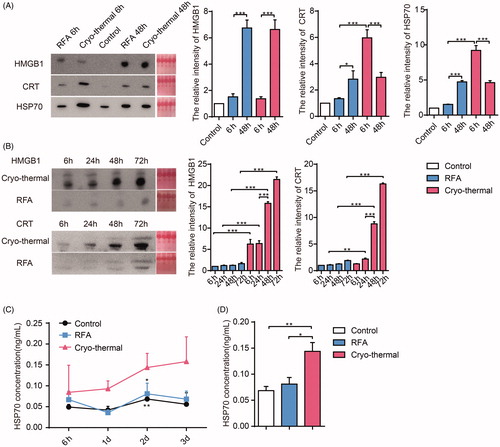
Cryo-thermal therapy could not only lyse tumor cells but also injure the tumor microvasculature and increase capillary permeability. We hypothesized that DAMPs released in tumor tissues after cryo-thermal therapy also leak into the peripheral circulation. We next analyzed the levels of HSP70, HMGB1 and CRT in the serums. The levels of all three DAMPs in the cryo-thermal group were increased at 72 h after treatment and were much higher than those in the RFA group (). Overall, cryo-thermal therapy could enhance the release of DAMPs both in situ and in the peripheral circulation, while RFA could only trigger the release of DAMPs in situ, which could not enter the peripheral circulation.
Released DAMPs elicited by cryo-thermal treatment decreased the proportion of MDSCs and inhibited their suppressive signature
MDSCs can inhibit both innate and adaptive immunity through (i) suppressing the activation of effector T cells, NK cells and DCs; (ii) promoting the differentiation of Th2 cells and Tregs; and (iii) promoting the M2 polarization of macrophages [Citation29]. Our previous studies showed that cryo-thermal therapy could downregulate the proportion of MDSCs in the spleen [Citation24]. To study whether DAMPs regulate the suppressive signature of MDSCs, MDSCs isolated from tumor-bearing mouse spleens (23 days after inoculation) were cultured in normal, tumor-bearing (23 days after inoculation) or cryo-thermal-treated mouse serum (2 days after cryo-thermal therapy) in vitro. The proportion of MDSCs (CD11b+Gr-1+) was analyzed by using FACS. Compared with that in the normal group, the percentage of MDSCs in the tumor-bearing mouse group was increased, but in the cryo-thermal therapy group, the proportion of MDSCs was reduced to a normal level ().
Figure 2. The proportion and suppressive signature of MDSCs after cryo-thermal therapy following DAMPs neutralization. (A) MDSCs isolated from the spleens of tumor-bearing mice were cultured with serums from normal, tumor-bearing control or cryo-thermal treated mice in vitro for 24 h, and the proportion of MDSCs was tested by FACS; (B) MDSCs isolated from the spleens of tumor-bearing mice were cultured with serums from normal, tumor-bearing control or cryo-thermal treated mice in vitro for 24 h with/or without the addition of anti-HSP70, anti-CRT or anti-HMGB1, and the proportions of MDSC were tested by using FACS; (C) The expression levels of suppressive molecules of MDSC were tested by using qRT-PCR after MDSCs were cultured with normal, tumor-bearing control or cryo-thermal treated mice serum. (D) Suppressive molecules of MDSCs were tested by using qRT-PCR after MDSCs were cultured with tumor-bearing control or cryo-thermal treated mice serum with or without the addition of anti-HSP70, anti-CRT or anti-HMGB1. Data were shown as mean ± SD. *p < .05, **p < .01, ***p < .001 by one-way ANOVA.
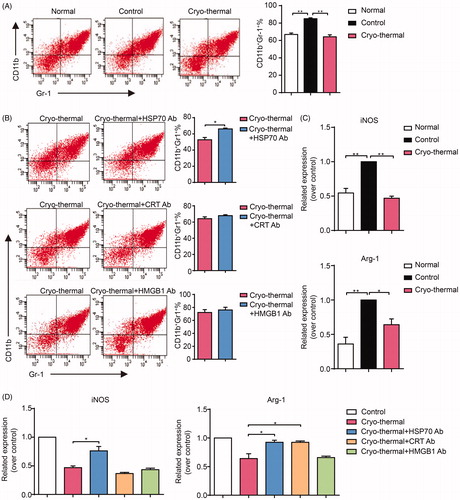
The proportion of MDSCs was detected using FACS, and the immunosuppressive properties of MDSCs were examined by qRT-PCR. The percentage of MDSCs was increased when MDSCs were cultured in cryo-thermal treated mouse serum with the addition of the anti-HSP70 antibody compared with serum without the addition of antibodies, while with the addition of the anti-CRT or anti-HMGB1 antibody, the percentage of MDSCs was almost unchanged (). The expression of immunosuppressive molecules in MDSCs, such as Arg-1 and iNOS, were decreased when MDSCs were cultured in cryo-thermal treated mouse serum (). However, the mRNA levels of Arg-1 and iNOS were increased with the addition of the anti-HSP70 antibody. With the addition of the anti-CRT antibody, only the mRNA level of Arg-1 was upregulated, while with the addition of the anti-HMGB1 antibody, the mRNA levels of Arg-1 and iNOS in MDSCs were not changed (). Taken together, the above results indicated that HSP70 released after cryo-thermal therapy could decrease the proportion of MDSCs and impair the immunosuppressive molecules expression of MDSCs.
DAMPs released in response to cryo-thermal therapy promoted the M1 polarization of macrophages
Tumor-associated macrophages can be polarized into M1 or M2 macrophages in response to different stimuli. The M2 polarization of macrophages, which is associated with tumor progression, is characterized by low expression of MHC II and high secretion of IL-10 [Citation25,Citation30,Citation31]. In contrast, M1 macrophages, which are characterized by the production of proinflammatory and immunostimulatory cytokines, express high levels of MHC I and II and thus play a critical role in tumor antigen presentation [Citation32]. To explore the role of DAMPs in macrophage polarization, macrophages isolated from the spleens of tumor-bearing mice (23 days after inoculation) were cultured in normal, tumor-bearing (23 days after inoculation) or cryo-thermal treated mouse serums (2 days after cryo-thermal therapy) with or without the addition of the anti-HSP70, anti-CRT or anti-HMGB1 antibodies. Twenty-four hours later, the proportion of M1 macrophages was analyzed by FACS, and related molecules representing macrophage functions were examined by qRT-PCR. The proportion of M1 macrophages (F4/80+CD86+MHC II+) was upregulated when macrophages were cultured in cryo-thermal treated mouse serums (). Consistently, the expression of chemokines or cytokines in macrophages, such as CXCL10 and IL-12, were significantly increased, while the expression of immunosuppressive molecules in macrophages, including Arg-1, iNOS and IL-10, were markedly decreased (). The level of IL-6, which is associated with acute inflammation in the early stage, was also reduced (). The expression of CD206, which mediates antigen phagocytosis, was decreased after macrophages were cultured with cryo-thermal treated mouse serum. After neutralizing CRT or HSP70, the percentage of M1 macrophages was noticeably decreased, while after neutralizing HMGB1, the percentage of M1 macrophages was not obviously changed (). Further analysis of related molecules representing the functions of macrophages showed that the levels of CD86, MHC II, CXCL10 and IL-12 were decreased after neutralizing the three DAMPs. Specifically, with the addition of the anti-HSP70 antibody, the levels of TNF-α, CD206 and CCL2 were downregulated, while the levels of immunosuppressive molecules, including Arg-1, iNOS and IL-10, were upregulated (). With the addition of the anti-CRT antibody, the expression levels of Arg-1, CD206 and IL-10 were increased, but the levels of these molecules were not changed with the addition of the anti-HMGB1 antibody (). Overall, DAMPs released after cryo-thermal therapy could promote the M1 polarization of macrophages. Among the three DAMPs, HSP70 had the largest effect on M1 macrophage polarization, followed by CRT, and the minimum effect on M1 macrophage polarization was observed with targeting HMGB1.
Figure 3. DAMPs elicited by cryo-thermal promoted M1 macrophage polarization. (A-B) Macrophages isolated from the spleens of tumor-bearing mice were cultured in serums from normal, tumor-bearing control or cryo-thermal treated mice in vitro for 24 h. LPS (100 ng/ml) and IFN-γ (100 ng/ml) were used to treat the macrophages as positive control. The proportions of macrophage were analyzed by using FACS (A) and the immune related molecules were tested by using qRT-PCR (B); (C-D)Macrophages isolated from the spleens of tumor-bearing mice were cultured in serums from tumor-bearing control or cryo-thermal treated mice with or without the addition of anti-HSP70, anti-CRT or anti-HMGB1 in vitro for 24 h, and the proportions of macrophage were analyzed by using FACS (C) and the immune related molecules were tested by using qRT-PCR (D). Data were shown as mean ± SD. *p < .05, **p < .01, ***p < .001 by one-way ANOVA.
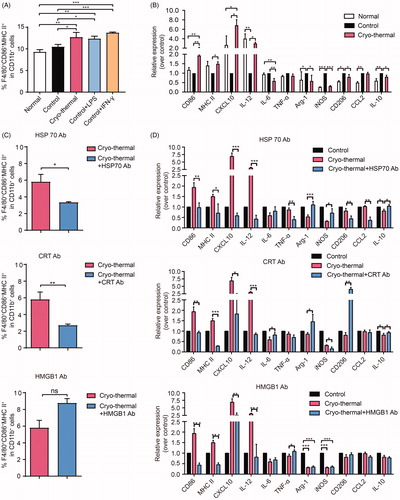
DAMPs elicited by cryo-thermal therapy promoted DC maturation
In the tumor environment, immature DCs can induce anergy in T cells and the differentiation of Tregs [Citation33], while mature DCs can effectively present tumor antigens and stimulate cytotoxic T cell activation. In addition, mature DCs can also change the immunosuppressive environment and induce a long-lasting antitumor immune response [Citation34]. To explore the roles of DAMPs in DC maturation, CD11c+ DCs isolated from the spleens of tumor-bearing mice (23 days after inoculation) were cultured in normal, tumor-bearing (23 days after inoculation) or cryo-thermal treated mouse serum (2 days after cryo-thermal therapy) with or without the addition of the anti-HSP70, anti-CRT or anti-HMGB1 antibody. The proportion of mature DCs (CD86+MHC II+DCs) was analyzed by FACS, and the expression levels of related molecules were examined by qRT-PCR. The percentages of CD11c+CD86+MHC II+ mature DCs were significantly increased when DCs were cultured in cryo-thermal treated mouse serums (). In addition, compared with those in tumor-bearing control DCs, the levels of inflammatory cytokines and chemokines in DCs cultured with cryo-thermal treated mouse serums, such as TNF-α, IL-12, IL-7, IL-15 and CXCL10, were markedly increased, while the levels of immunosuppressive molecules, such as IL-10, PD-L1, STAT3, IDO1 and HO-1, were significantly downregulated (). The levels of IL-1β and IL-6 in DCs cultured with cryo-thermal treated mouse serum were decreased, whereas the level of IDO2 was increased (). The expression of VEGFR2 and FOXO3 in DCs cultured with control or cryo-thermal treated serums showed no obvious differences (). After neutralizing CRT, HMGB1 or HSP70, the percentage of mature DCs was decreased (). Further analysis of related molecules showed that the levels of IL-7 and IDO2 were decreased, while the expression of PD-L1 was increased after neutralizing the three DAMPs (). With the addition of the anti-HSP70 or anti-HMGB1 antibody, the level of IDO-1 was upregulated, and the expression of IL-15 was decreased with the addition of the anti-HMGB1 antibody (). Other molecules showed no significant change after neutralizing the three DAMPs. In conclusion, the three DAMPs released after cryo-thermal therapy could promote DC phenotypic maturation and partially stimulate the expression of inflammatory cytokines, as well as inhibit immunosuppressive molecule expression.
Figure 4. DAMPs elicited by cryo-thermal promoted phenotypic DCs maturation. (A-B) DCs isolated from the spleens of tumor-bearing mice were cultured with normal, tumor-bearing control or cryo-thermal treated mice serums in vitro for 24 h. LPS (100 ng/ml) and IFN-γ (100 ng/ml) were used to treat the DCs as positive control. The proportions of CD86+MHC II+ mature DCs were analyzed by using FACS (A). The immune related molecules were tested by using qRT-PCR (B); (C-D) DCs isolated from the spleens of tumor-bearing mice were cultured with tumor-bearing control or cryo-thermal treated mice serums with or without the addition of anti-HSP70, anti-CRT or anti-HMGB1 in vitro for 24 h, and the proportions of DC were analyzed by using FACS (C) and the immune related molecules were tested by using qRT-PCR (D). Data were shown as mean ± SD. *means comparing with control, *p < .05, **p < .01, ***p < .001 by one-way ANOVA.
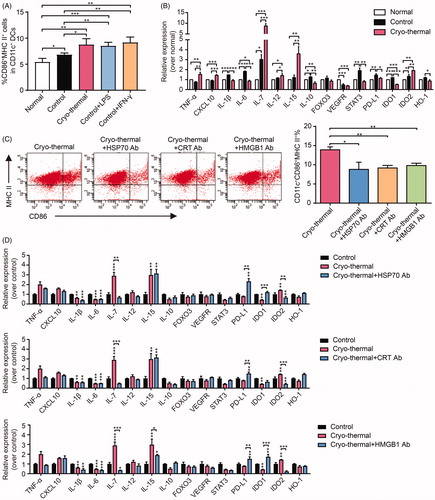
Neutralizing extracellular released HSP70 in vivo shortened the long-term survival time of cryo-thermal treated mice
The above results indicated that the polarization of M1 macrophages and maturation of DCs maturation could be triggered by the three DAMPs released after cryo-thermal therapy, especially HSP70. Moreover, only HSP70 released after cryo-thermal therapy could decrease the frequency of MDSCs and impair the immunosuppressive signature of MDSCs. To further demonstrate the role of HSP70 in antitumor immunity, 200 μg anti-HSP70 antibody was injected intravenously at 6 h after cryo-thermal therapy on day 1 and day 2 (n = 6 in each group), and mouse survival time was recorded. As shown in , half of the cryo-thermal treated mice were very healthy and robust, while all of the mice in the anti-HSP70 antibody neutralization group died within 82 days. These results indicated that HSP70 released after cryo-thermal therapy played a key role in the activation of antitumor immunity.
Discussion
In this study, we revealed the roles of DAMPs released after cryo-thermal therapy in stimulating antitumor immunity against 4T1 murine mammary carcinomas. Our studies showed that a large amount of DAMPs (HSP70, CRT and HMGB1) was not only released in situ after cryo-thermal therapy but also entered the peripheral circulation. Moreover, the three released DAMPs (HSP70, CRT and HMGB1) could promote the M1 polarization of macrophages and maturation of DCs. In particular, HSP70 had the most important roles in M1 macrophage polarization and DC maturation, and only HSP70 released after cryo-thermal therapy could decrease the frequency of MDSCs and impair the immunosuppressive molecules expression of MDSCs. Neutralizing extracellular released HSP70 in vivo shortened the long-term survival time of cryo-thermal treated mice. We suggest that extracellular HSP70 released after cryo-thermal therapy plays the most important role in restoring the systemic immune state and triggering the antitumor immune response.
The adaptive immune response is crucial in inducing strong and durable antitumor immunity [Citation35]. However, the activation and expansion of tumor-specific T cells require the maturation of the innate immune system [Citation36]. Mature APCs can activate naïve T cells through tumor-associated antigen presentation, costimulatory signaling and inflammatory cytokine secretion [Citation37]. Our previous studies reported that cryo-thermal therapy could promote APC maturation, then mature APCs induced the differentiation of CD4+ T cells into the Th1 and CTL subsets, inhibited the differentiation of the Th2 and Treg subsets, and enhanced the cytotoxicity of CD8+ T cells [Citation26], which indicated that mature innate immune cells, including macrophages and DCs, were key mediators of the cryo-thermal-induced long-term antitumor immune response. However, why cryo-thermal therapy induces the maturation of APCs remain unknown.
DAMPs have been considered to have central effects on tumor cell organization and microenvironment alteration as well as T cell activation [Citation38]. However, in the process of tumor growth, the death of tumor cells, which is caused by hypoxia or genetic/mechanical stress, also chronically releases DAMPs [Citation28]. The chronic release of DAMPs by tumor cells leads to immune tolerance in APCs, whereas the release of a large amount of DAMPs after tumor cells are lysed induces acute inflammation [Citation39]. Therefore, the patterns of cell death can determine the modes of DAMP release and subsequent strength and type of the immune response. The thermal and mechanical stresses caused by cryo-thermal therapy facilitated tumor cell membrane disruption, which led to the acute release of tumor-derived DAMPs in situ [Citation24]. In addition, cryo-thermal therapy could damage blood vessels and increase the permeability of blood vessels [Citation23], which helped locally released DAMPs enter the peripheral circulation and subsequently induced a systemic immune response.
Cryo-thermal therapy could induce tumor cell necrosis characterized as first the destruction of the cell membrane and then fusion of the cytoplasm and late nuclear material, which was broken up into small particles [Citation24]. HSP70 and CRT are mainly expressed in the endoplasmic reticulum, while HMGB1 is located in the nucleus [Citation11,Citation12,Citation15]. The fact that cryo-thermal therapy-induced HSP70 and CRT release (at 6 h after treatment) occurred much earlier than HMGB1 release (at 48 h after treatment) also indicated that the major pattern of cryo-thermal therapy-induced cell death was necrosis.
In this study, cryo-thermal therapy-induced HSP70 release effectively reduced the proportion of MDSCs and inhibited MDSC immunosuppressive molecules expression. In addition, the cryo-thermal therapy-induced release of these three DAMPs upregulated the proportions of M1 macrophages and mature DCs, which indicated that cryo-thermal therapy-derived DAMPs could effectively reverse immunosuppression and systemically activate innate immune cells. We found that HSP70 and CRT were able to induce M1 macrophage polarization and that the period of M1 macrophage polarization (at 24 h after treatment) was much earlier than that of DC maturation (data not shown), which indicated that the earlier release of HSP70 and CRT was first detected by macrophages, not DCs. Specifically, the in situ level of HMGB1 was increased at 48 h after treatment, and thus, it occurred after the best time to induce M1 macrophage polarization. In addition, HSP70 and HMGB1 act through common receptors [Citation40]. Because HSP70 and HMGB1 competitively bind to receptors on APCs and the release of HSP70 after cryo-thermal therapy occurs much earlier than the release of HMGB1, the function of HMGB1 binding to APC receptors is replaced by HSP70 binding. We found that HSP70 could upregulate the expression of TNF-α, CD206 and CCL2 but downregulate the level of iNOS, while CRT could downregulate the expression of CD206. CD206 can mediate glycosylated antigen phagocytosis in macrophages [Citation41]. Naïve APCs increase antigen uptake after activation, but mature APCs downregulate the function of phagocytosis and overexpress MHC molecules [Citation42]. Hence, HSP70 and CRT competitively adjust the antigen uptake activity of macrophages. The upregulation of CD206 expression promoted by released HSP70 after cryo-thermal treatment would directly mediate the phagocytosis of tumor antigens and released CRT would impair the phagocytosis of tumor antigens through downregulation of CD206 expression. In addition, released HSP70 can also be recognized by TLR 4 [Citation43], which further promotes MHC molecule expression and facilitates M1 macrophage polarization. M1 macrophages express high levels of TNF-α, which can directly kill tumor cells [Citation44], and trigger innate and adaptive immune responses [Citation45]. Moreover, TNF-α can upregulate the expression of CCL2, which functions in monocyte attraction [Citation46]. Monocytes further differentiate into M1 macrophages or mature DCs in an immunostimulatory environment and facilitate antitumor responses.
In contrast to their roles in M1 macrophage polarization, the roles of the three DAMPs in DC maturation were similar but less dramatic. After neutralization of the three DAMPs, there were fewer changes in cytokine and immune-related molecule expression in DCs than in macrophages, which indicated that the DAMPs had a smaller effect on DC maturation than on monocyte maturation and that DC maturation was mediated by other factors in the serum. The expression level of IDO2 in DCs was downregulated after the addition of antibodies specific for the three DAMPs. IDO2 is a homolog of IDO1, which is generally considered to help tumor cell immune escape and promote immune tolerance [Citation47]. However, more evidence has shown that IDO2 operates as a proinflammatory mediator by initializing cross-talk between B and T cells and stimulating the expression of IL-4, IL-6 and IL-21 in T cells [Citation48]. Furthermore, released HMGB1 could upregulate the expression of IL-15 in DCs, which can stimulate the generation of NK cells, NK-T cells, γδ T cells, ILC1 and memory CD8+ T cells [Citation49].
Considering that the three released DAMPs, especially released HSP70, could enhance M1 macrophage polarization and DC maturation and, moreover, that only HSP70 released after cryo-thermal therapy could decrease the MDSC frequency and impair their immunosuppressive signature, we suggested that released HSP70 was the most important DAMP in promoting innate and adaptive immune responses. The survival rate of cryo-thermal-treated mice after HSP70 neutralization was much lower than that after neutralization of the other DAMPs, which further demonstrated the evident role of HSP70 in the induction of the systemic antitumor response.
In this study, we did not distinguish the population of MDSCs. The different populations of MDSCs utilize different mechanisms of immune suppression, but both of M-MDSC and PMN-MDSC suppress the antitumor immune response [Citation50]. In this study, we were focused on the percentage of total MDSCs, and the population of MDSCs affected by cryo-thermal therapy will be studied in near future. In this study, we found cryo-thermal therapy promoted DCs maturation, but how DCs maturation was induced after cryo-thermal therapy was not involved. Now, we are carrying out another study on how APCs capture and present tumor specific antigens after cryo-thermal therapy. The results will be reported in near future.
In summary, cryo-thermal therapy could significantly induce the release of tumor-related DAMPs in situ and in the peripheral circulation. The high levels of DAMPs, especially HSP70, impaired the level and suppressive signature of MDSCs and promoted M1 macrophage polarization and DC maturation, leading to the enhanced presentation of tumor-derived antigens and creation of an inflammatory environment for the stimulation of T cell activation and differentiation. This study suggested that local cryo-thermal therapy could induce the release of a large amount of DAMPs to effectively activate a systemic and durable antitumor response, which could open up a broader field of thermal-physical tumor immunotherapy.
Disclosure statement
No potential conflict of interest was reported by the author(s).
Additional information
Funding
Reference
- Li X, Yang J, Peng L, et al. Triple-negative breast cancer has worse overall survival and cause-specific survival than non-triple-negative breast cancer. Breast Cancer Res Treat. 2017;161(2):279–287.
- Dunn GP, Bruce AT, Ikeda H, et al. Cancer immunoediting: from immunosurveillance to tumor escape. Nat Immunol. 2002;3(11):991–998.
- Garg AD, Dudek AM, Agostinis P. Cancer immunogenicity, danger signals, and DAMPs: what, when, and how? Biofactors. 2013;39(4):355–367.
- Zitvogel L, Tesniere A, Kroemer G. Cancer despite immunosurveillance: immunoselection and immunosubversion. Nat Rev Immunol. 2006;6(10):715–727.
- Galluzzi L, Vacchelli E, Bravo-San Pedro JM, et al. Classification of current anticancer immunotherapies. Oncotarget. 2014;5(24):12472–12508.
- Blankenstein T, Coulie PG, Gilboa E, et al. The determinants of tumour immunogenicity. Nat Rev Cancer. 2012;12(4):307–313.
- Matzinger P. Tolerance, danger, and the extended family. Annu Rev Immunol. 1994;12:991–1045.
- Rock KL, Hearn A, Chen CJ, et al. Natural endogenous adjuvants. Springer Semin Immunopathol. 2005;26(3):231–246.
- Garg AD, Krysko DV, Vandenabeele P, et al. DAMPs and PDT-mediated photo-oxidative stress: exploring the unknown. Photochem Photobiol Sci. 2011;10(5):670–680.
- Garg AD, Nowis D, Golab J, et al. Immunogenic cell death, DAMPs and anticancer therapeutics: an emerging amalgamation. Biochim Biophys Acta. 2010;1805(1):53–71.
- Asea A, Kraeft SK, Kurt-Jones EA, et al. HSP70 stimulates cytokine production through a CD14-dependant pathway, demonstrating its dual role as a chaperone and cytokine. Nat Med. 2000;6(4):435–442.
- Gold LI, Eggleton P, Sweetwyne MT, et al. Calreticulin: non-endoplasmic reticulum functions in physiology and disease. FASEB J. 2010;24(3):665–683.
- Inoue H, Tani K. Multimodal immunogenic cancer cell death as a consequence of anticancer cytotoxic treatments. Cell Death Differ. 2014;21(1):39–49.
- Obeid M, Tesniere A, Ghiringhelli F, et al. Calreticulin exposure dictates the immunogenicity of cancer cell death. Nat Med. 2007;13(1):54–61.
- Müller S, Ronfani L, Bianchi ME. Regulated expression and subcellular localization of HMGB1, a chromatin protein with a cytokine function. J Intern Med. 2004;255(3):332–343.
- Kroemer G, Galluzzi L, Kepp O, et al. Immunogenic cell death in cancer therapy. Annu Rev Immunol. 2013;31:51–72.
- Zitvogel L, Kepp O, Kroemer G. Decoding cell death signals in inflammation and immunity. Cell. 2010;140(6):798–804.
- Garg AD, Martin S, Golab J, et al. Danger signalling during cancer cell death: origins, plasticity and regulation. Cell Death Differ. 2014;21(1):26–38.
- Chen G, Ward MF, Sama AE, et al. Extracellular HMGB1 as a proinflammatory cytokine. J Interferon Cytokine Res. 2004;24(6):329–333.
- Tang D, Kang R, Coyne CB, et al. PAMPs and DAMPs: signal 0s that spur autophagy and immunity. Immunol Rev. 2012;249(1):158–175.
- Chen L, Flies DB. Molecular mechanisms of T cell co-stimulation and co-inhibition. Nat Rev Immunol. 2013;13(4):227–242.
- Yatim N, Cullen S, Albert ML. Dying cells actively regulate adaptive immune responses. Nat Rev Immunol. 2017;17(4):262–275.
- Zhang K, Zou J, He K, et al. Study of enhanced radiofrequency heating by pre-freezing tissue. Int J Hyperthermia. 2018;35(1):79–89.
- Zhu J, Zhang Y, Zhang A, et al. Cryo-thermal therapy elicits potent anti-tumor immunity by inducing extracellular Hsp70-dependent MDSC differentiation. Sci Rep. 2016;6:27136.
- He K, Liu P, Xu LX. The cryo-thermal therapy eradicated melanoma in mice by eliciting CD4+ T-cell-mediated antitumor memory immune response. Cell Death Dis. 2017;8(3):e2703.
- He K, Jia S, Lou Y, et al. Cryo-thermal therapy induces macrophage polarization for durable anti-tumor immunity. Cell Death Dis. 2019;10(3):216.
- Shen Y, Liu P, Zhang A, et al. Study on tumor microvasculature damage induced by alternate cooling and heating. Ann Biomed Eng. 2008;36(8):1409–1419.
- Hernandez C, Huebener P, Schwabe RF. Damage-associated molecular patterns in cancer: a double-edged sword. Oncogene. 2016;35(46):5931–5941.
- Gabrilovich DI, Ostrand-Rosenberg S, Bronte V. Coordinated regulation of myeloid cells by tumours. Nat Rev Immunol. 2012;12(4):253–268.
- Mantovani A, Marchesi F, Malesci A, et al. Tumour-associated macrophages as treatment targets in oncology. Nat Rev Clin Oncol. 2017;14(7):399–416.
- Chen C, Zhang A, Cai Z, et al. Design of microprobe for accurate thermal treatment of tumor. Cryo Lett. 2011;32(3):275–286.
- Cai Z, Song M, Sun J, et al. Design of a new probe for tumor treatment in the alternate thermal system based on numerical simulation. Conf Proc IEEE Eng Med Biol Soc. 2011;2011:6874–6877.
- Qian BZ, Pollard JW. Macrophage diversity enhances tumor progression and metastasis. Cell. 2010;141(1):39–51.
- Bellora F, Castriconi R, Dondero A, et al. TLR activation of tumor-associated macrophages from ovarian cancer patients triggers cytolytic activity of NK cells. Eur J Immunol. 2014;44(6):1814–1822.
- Vatner RE, Janssen EM. STING, DCs and the link between innate and adaptive tumor immunity. Mol Immunol. 2019;110:13–23.
- Moynihan KD, Opel CF, Szeto GL, et al. Eradication of large established tumors in mice by combination immunotherapy that engages innate and adaptive immune responses. Nat Med. 2016;22(12):1402–1410.
- Ellmark P, Mangsbo SM, Furebring C, et al. Tumor-directed immunotherapy can generate tumor-specific T cell responses through localized co-stimulation. Cancer Immunol Immunother. 2017;66(1):1–7.
- Patidar A, Selvaraj S, Sarode A, et al. DAMP-TLR-cytokine axis dictates the fate of tumor. Cytokine. 2018;104:114–123.
- Garg AD, Agostinis P. Cell death and immunity in cancer: from danger signals to mimicry of pathogen defense responses. Immunol Rev. 2017;280(1):126–148.
- Krombach J, Hennel R, Brix N, et al. Priming anti-tumor immunity by radiotherapy: dying tumor cell-derived DAMPs trigger endothelial cell activation and recruitment of myeloid cells. Oncoimmunology. 2019;8(1):e1523097.
- Dasgupta S, Bayry J, Lacroix-Desmazes S, et al. Human mannose receptor (CD206) in immune response: novel insights into vaccination strategies using a humanized mouse model. Expert Rev Clin Immunol. 2007;3(5):677–681.
- Watts C, West MA, Zaru R. TLR signalling regulated antigen presentation in dendritic cells. Curr Opin Immunol. 2010;22(1):124–130.
- Płóciennikowska A, Hromada-Judycka A, Borzęcka K, et al. Co-operation of TLR4 and raft proteins in LPS-induced pro-inflammatory signaling. Cell Mol Life Sci. 2015;72(3):557–581.
- Josephs SF, Ichim TE, Prince SM, et al. Unleashing endogenous TNF-alpha as a cancer immunotherapeutic. J Transl Med. 2018;16(1):242.
- de Matos LG, Cândido EB, Vidigal PV, et al. Association between Toll-like receptor and tumor necrosis factor immunological pathways in uterine cervical neoplasms. Tumori. 2017;103(1):81–86.
- Shi L, Wang J, Ding N, et al. Inflammation induced by incomplete radiofrequency ablation accelerates tumor progression and hinders PD-1 immunotherapy. Nat Commun. 2019;10(1):5421.
- Merlo LM, Mandik-Nayak L. IDO2: a pathogenic mediator of inflammatory autoimmunity. Clin Med Insights Pathol. 2016;9(Suppl 1):21–28.
- Merlo LMF, Pigott E, DuHadaway JB, et al. IDO2 is a critical mediator of autoantibody production and inflammatory pathogenesis in a mouse model of autoimmune arthritis. J Immunol. 2014;192(5):2082–2090.
- Waldmann TA, Miljkovic MD, Conlon KC. Interleukin-15 (dys)regulation of lymphoid homeostasis: implications for therapy of autoimmunity and cancer. J Exp Med. 2020;217(1):e20191062.
- Gabrilovich DI. Myeloid-derived suppressor cells. Cancer Immunol Res. 2017;5(1):3–8.