Abstract
More than 130-year ago, Sir Victor Horsley delivered a landmark address to the British Medical Association, in which he described successful localization and resection of an epileptogenic focus resulting in seizure freedom for the patient. Several important steps in epilepsy surgery have been achieved since, including resection techniques such as anterior temporal lobectomy and selective amygdalohippocampectomy, both resulting in 70–80% seizure freedom and distinct differences in neuropsychological outcomes. The most recent addition to techniques for epilepsy surgery is minimally invasive thermal therapy. Significant advances in imaging technology and thermal ablation have opened a novel avenue for epilepsy treatment, permitting surgical intervention with seizure-freedom rates approaching the success of traditional methods but with reduced invasiveness, blood loss and duration of postoperative hospital stay. Here, we review recent advances on stereotactic ablation techniques focused on epilepsy surgery. Finally, we present emerging navigation techniques, which allow a higher degree of freedom. The described technologies render precise navigation of the ablation probe to avoid critical structures along the trajectory path and open novel pathways to further minimize invasiveness and improve safety and efficacy. Improve safety and efficacy.
Introduction
Epilepsy surgery has been advanced over a long history of several foundational developments. In 1886, Sir Victor Horsley delivered a landmark address to the British Medical Association on ‘Advances in the Surgery of the Central Nervous System’, in which he described successful seizure localization in three patients, who suffered from post-traumatic epilepsy as a result of depressed skull fractures following a traffic accident. Drawing on the pioneering work of John Hughlings Jackson, Horsley resected the epileptogenic focus resulting in seizure-freedom for the patients. This report represents the first description of epilepsy surgery and was the foundation upon which subsequent work can be considered. A seminal study on localization of temporal lobe epilepsy (TLE) was presented by William Feindel and Wilder Penfield in 1954, critically advancing the field and allowing precise targeting of structures identified for surgical resection. Once the efficacy of temporal lobectomy was established, techniques for optimizing this operation fell into two schools of practice: The practice of tailored resection by Ojemann postulated that the extent of the epileptogenic zone and the localization of eloquent areas vary in each patient. Therefore, surgery was conformed individually based on results of electrocorticography and functional mapping in the respective case [Citation1]. The anteromedial temporal technique developed by Spencer accessed mesial temporal structures through the temporal pole corridor to preserve lateral temporal neocortex function [Citation2]. The latter ultimately led to the so called selective amygdalohippocampectomy (SAH) technique described in detail below. Recently, stereotactic thermal ablation has emerged as a novel treatment technique to these traditional methods with seizure-freedom statistics approaching those of the open resections but with significantly less invasiveness, neuropsychiatric deficits and hospital duration. In this review, we discuss the recent advances and challenges in thermal ablation for epilepsy surgery.
Physiological and anatomical basis of epileptic seizures
In epilepsy, nerve cells of a seizure focus undergo sudden depolarization that triggers an uncontrolled chain-reaction of action-potential activation. The resulting discharge of neuronal ensembles together with dysfunctional inhibitory inputs manifests as a seizure. This spread may continue along axonal pathways intra-hemispherically on the same side of the brain or inter-hemispherically to contralateral regions. Causally, genetic, structural, metabolic, idiopathic or cryptogenic features lead to altered cellular properties and/or synaptic connections resulting in the above-mentioned imbalance between increased excitability and decreased inhibition. Anatomically, most seizures arise in the mesial aspect of the temporal lobe including the hippocampus, parahippocampal gyrus (PHG), amygdala and the entorhinal cortex. TLE is frequently associated with hippocampal sclerosis. As such, mesial temporal lobe epilepsy with hippocampal sclerosis (MTLE-HS) is a specific epilepsy constellation in the 2017 International League Against Epilepsy (ILAE) classification system portraying its critical role in seizures.
Medical therapy vs surgery for epilepsy treatment
In an antiepileptic-naïve population with newly diagnosed seizures, the first antiepileptic drug (AED) controls seizures in 50% of the patients, a second AED adds 15%, but only 4% achieve seizure-freedom with a third AED trial [Citation3]. Over the past several decades, the introduction of novel anticonvulsant agents has increased the tolerability of these drugs but not significantly improved their efficacy [Citation4]. According to the ILAE, drug resistant epilepsy is defined as ‘failure of adequate trials of two tolerated, appropriately chosen and used AED schedules to achieve sustained seizure freedom’. MTLE-HS is the most common cause of pharmaco-resistant epilepsy. The first randomized, controlled trial demonstrated that surgery is superior to medical therapy in TLE [Citation5], as 58% of patients who received surgery remained seizure-free at 1 year compared with 8% in the medical arm. Based on the evidence, the guidelines of the American Academy of Neurology in association with the American Epilepsy Society and the Association of Neurological Surgeons began recommending surgery as the treatment of choice for pharmaco-resistant TLE. The Early Randomized Surgical Epilepsy Trial revealed that medical therapy with early surgery provides better seizure control than continued medical management alone in newly diagnosed TLE [Citation6]. Although this study was prematurely terminated due to slow enrollment, nearly 75% (11/15) of surgically treated patients remained seizure-free at 2 years, while none of the 23 patients in the medical arm became seizure-free. Several additional studies show that seizure-freedom probability after surgery for TLE was between 50 and 75% for up to 10 years [Citation7]. This evidence urges the need for advanced and targeted surgical therapies for TLE.
Traditional surgical treatment options in pharmaco-resistant epilepsy
The most common surgical procedures for TLE are anterior temporal lobectomy (ATL) and SAH. The ATL procedure can be conceptualized as two stages, in which the lateral and mesial parts of the temporal lobe are resected. Typically, the extent of resection is 3–4 cm in the language-dominant hemisphere and 5–6 cm in the non-dominant side to avoid postoperative visual field deficits attributed to injury of the geniculocalcarine tract (Meyer’s Loop), which results in a specific visual field deficit (contralateral superior quadrantanopsia). SAH minimizes resection compared to ATL and can be performed through varying trajectories: Transcortical-transventricular [Citation8], transsylvian [Citation9] and subtemporal [Citation10]. Postoperative seizure outcomes are usually reported according to the Engel classification ranging from Engel I (seizure-freedom) to IV (no worthwhile improvement) [Citation11]. More recent adaptations include (i) the modified Engel classification, which assesses postoperative seizure outcomes annually and in seizures days instead of an absolute seizure number to allow better comparison between individual patients, different centers and AEDs [Citation12] and (ii) the ILAE classification, where the terminology was re-defined and seizures were classified based on their onset, specification of motor symptoms and non-motor symptoms [Citation13]. Seizure outcomes for ATL and SAH were reported equivalent, regardless of surgery or approach [Citation14]: A 71% seizure-freedom rate after >10 year follow-up was shown with the transsylvian approach [Citation15]; similar rates were described for the transcortical and subtemporal approaches [Citation16–18]. This notion has however been recently challenged by several meta-analyses favoring higher seizure freedom with ATL, although rates of seizure freedom were only subtlety different [Citation19,Citation20]. Notably, neurocognitive outcomes are superior with SAH [Citation21], in particular verbal IQ and verbal memory [Citation22], implying that less invasive approaches preserve more normal anatomy leading to less postoperative deficits.
Laser ablation in neurosurgery
The first applications of lasers in the brain began in 1965 demonstrating the destructive effect of pulse laser technology in animal tissues [Citation23–26]. Next, laser technology was used in the human brain for tumor ablation [Citation27]. The aimed tumor necrosis, however, was incomplete insinuating that, although tissue destruction was possible, further work was needed to provide controlled and targeted lesion ablation. This was achieved shortly after, using a high-power continuous wave CO2 laser [Citation28]. However, this process was not applicable in daily practice as it was highly time-consuming. The technology was then modified to provide a practical version of the CO2 laser. Using this principle, a large series of 250 central nervous system (CNS) ablations for extra-axial tumors and intra-axial vascular lesions was completed [Citation29]. In addition to the CO2 laser, neodymium-doped yttrium aluminum garnet (Nd:YAG) lasers attracted attention for medical applications. Nd:YAG lasers initially lacked the precision needed for neurosurgical operations due to reduced laser absorption by CNS tissue, which resulted in increased collateral damage [Citation30]. This laser differs in two main aspects from the CO2 laser: (i) Nd:YAG laser energy is selectively absorbed by blood and blood vessels allowing targeted blood vessel occlusion and (ii) the laser penetrates deeper than the CO2 laser providing more predictable laser penetration [Citation30,Citation31]. Due to these advantages, Nd:YAG lasers had a more pronounced effect on vascularized tissue than the CO2 laser. Nd:YAG lasers were eventually used to induce focal laser coagulation in 1983, which led to the development of laser interstitial thermal therapy (LITT) [Citation32,Citation33] culminating in the treatment of five patients with brain tumors under CT guidance [Citation34].
The next critical advance was the development and integration of magnetic resonance imaging (MRI). The first use of MRI to monitor thermal ablation involved preoperative target localization and postoperative distinction and identification between temporary and irreversible tissue destruction [Citation35]. Importantly, the technology was unable to estimate temperature alteration online (i.e., in real-time during the ablation procedure). Research over the past three decades has significantly improved this shortcoming, permitting accurate peri-procedural temperature control in today’s use of LITT. This addition expanded laser and thermal technology to emerge as an innovative treatment avenue for intracranial pathologies including epilepsy, neoplasms, radiation necrosis, movement disorders and chronic pain [Citation36,Citation37].
LITT for epilepsy treatment
MRI-guided laser ablation is a promising minimal-invasive alternative to the above-mentioned classical surgical approaches of ATL and SAH for pharmaco-resistant TLE () [Citation38]. Memory deficits and particularly verbal memory deficits are feared complications after TLE surgery. Given that SAH results in reduced neuropsychiatric deficits compared to ATL (see above), it is intriguing to speculate that a smaller extent of potentially functional tissue resection can spare function and reduce de novo post-surgical side effects. Drane et al. compared LITT with open resection and reported superior cognitive outcome for object recognition and naming in both, the dominant and non-dominant hemisphere [Citation39]. These findings have been confirmed by two other reports [Citation40,Citation41]. On the contrary, another study found laser ablation in the dominant hemisphere to result in a decline in verbal and narrative memory, also here no change was seen in naming [Citation42]. Contradicting results are reported for laser ablation and memory function, these differences may stem from small sample sizes, procedural differences with LITT and variability in test selection, patient cohorts and assessment [Citation43]. Studies investigating broader cognitive outcome are yet lacking. Prospective randomized-controlled trials are needed to investigate seizure-freedom and cognitive outcomes in resective and ablative procedures. The ongoing prospective, single-arm SLATE (Stereotactic Laser Ablation for TLE) trial is underway and hopefully brings more detailed answers to these questions. Advantages of minimal-invasive procedures such as LITT are reduced hospital stay and thus decreased risk of general medical complications such as infections, deep venous thrombosis and pulmonary embolism. Brachytherapy was the first modality used to induce stereotactic amygdalohippocampal lesioning [Citation44,Citation45]. However, it harbored the risk of radiation injury to adjacent blood vessels. An improved method with a string electrode was then used to induce segmental coagulations in 38 patients. Seizure-freedom was reported in 50% of the cases, 32% showed significant improvement and 18% remained unchanged [Citation46]. These results were compared to open surgery outcomes in the same institution: 52% seizure-freedom, 19% significant improvement and 29% unchanged. This represents the first major comparison between stereotactic radiofrequency ablation and open surgical outcomes after amygdalohippocampectomy for pharmaco-resistant TLE, showing that stereotactic ablation showed comparable and, in some outcomes, improved results to open surgery. With the development of MRI, an increasing number of centers began to use thermal coagulation. Most importantly, it was confirmed early on that seizure-free outcomes were comparable to open surgery [Citation47,Citation48]. An important step was the identification of the PHG as a critical structure to achieve seizure-freedom; which was significantly lower when the PHG was not coagulated [Citation49,Citation50]. In a sophisticated trial from the Czech Republic with 61 patients, an occipital insertion point was used to calculate a trajectory avoiding critical structures, vessels and the ventricles. Segmental lesioning of the hippocampus, amygdala and PHG was induced under MRI guided stereotaxis. Seizure-freedom (Engel I) was reported in 70% of the cases, concluding that stereotactic thermal ablation provides a safe and less-invasive modality resulting in comparable seizure-freedom to conventional surgical methods and improved cognitive outcomes [Citation51].
Figure 1. LITT Procedure. (A) Probe insertion through the StarFix frame (FHC Inc.) via an occipital entry. (B) Extent of laser ablation of the right amygdala, hippocampus, PHG and entorhinal cortex. Unpublished property of Dr. Joseph Neimat.
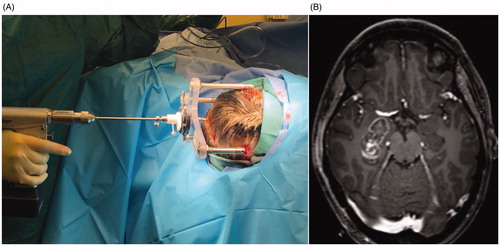
With the advent of MR thermography and the coupling of laser ablation systems to a calculation of presumed tissue damage, a more controlled ablation became possible. Two independent research efforts to create such a system arose independently and were ultimately commercialized as similar but distinct systems; Monteris Inc. and Visualase Inc. (the latter was later acquired by Medtronic Inc). The application of such real-time MRI guided ablation was first published as a small series of 13 patients in 2014. Seizure-freedom rates beyond 6 months were 67% in patients with preoperative mesial temporal sclerosis (MTS), whereas significantly lower Engel I outcomes (25%) were prevalent in non-MTS patients, suggesting the presence of MTS as a critical factor for seizure freedom after LITT [Citation52]. Other authors have reported >50% Engel I outcomes with LITT, which was lower than ATL; however, advantages for LITT over ATL were demonstrated for surgical morbidity, lower postoperative pain, shorter hospital stay and recovery period [Citation53]. A clearer picture is seen with neuropsychiatric outcomes: Several studies have demonstrated superior outcomes with LITT over conventional surgery [Citation39,Citation53,Citation54]. Object recognition and naming were tested postoperatively with the Boston Naming and Iowa Famous Faces Test in 19 LITT patients and 39 open temporal resections. Strikingly, while no patient with LITT experienced a decline, 32/39 patients with open resection revealed inferior outcomes in the two categories [Citation39]. Another important aspect is the extent of ablation with LITT. In open surgery, it was traditionally accepted that greater hippocampal resection results in improved seizure-freedom. However, various case series have disputed this notion identifying possible factors being difficulty in measuring the amount of resection precisely on MRI and poor standardization of the approach within and across studies [Citation55]. LITT on the other hand allows more standardization of the surgical technique and likely more precise measurement of the coagulated area offering a novel opportunity to assess critical regions of ablation. A large, multi-center, retrospective cohort study including 234 patients used an image-based methodology to normalize patient anatomy and quantify the extent of ablation on seizure outcomes in LITT for MTLE. The study revealed that coagulation of the amygdala, the hippocampal head, PHG and rhinal cortices maximizes the chance of seizure-freedom (). While greater amygdala ablation volume correlated with a higher Engel I outcome, a greater overall extent of resection was not favorable but possibly lead to inferior results when the resection was biased posteriorly [Citation56]. The large variance in seizure-free rates, however, suggests that research still needs to be done to elucidate key factors for seizure-freedom with LITT.
Continued challenges and future directions
The development of thermal ablation technologies opens new avenues and possibilities to treat neurosurgical pathologies. The variability of these pathologies and the long trajectories often required to reach deep targets has prompted the need for greater safety and control of the thermal ablation. An early innovation was to create a directional laser (side-fire) that could be rotated along the axis of trajectory to shape ablations. Thermal spread of the ablation ultimately limited this technique’s ability to tailor an ablation. In , this technique is compared to a traditional radial ablation in treating the amygdala.
Figure 2. Flexible catheters and curved trajectories. (A) Third-generation variable stiffness catheter allowing high degree of flexibility due to multiple magnetically steerable segments. (B) Animation of a transforaminal curved trajectory allowing ablation of the left amygdala, hippocampus, PHG and entorhinal cortex through a minimal-invasive corridor preserving normal anatomy. (C) Postoperative MRI demonstrating difference between diffuse and directed ablation. (A) Adapted with permission from Chautems et al. [Citation63]; (B, C) Unpublished property of Dr. Joseph Neimat.
![Figure 2. Flexible catheters and curved trajectories. (A) Third-generation variable stiffness catheter allowing high degree of flexibility due to multiple magnetically steerable segments. (B) Animation of a transforaminal curved trajectory allowing ablation of the left amygdala, hippocampus, PHG and entorhinal cortex through a minimal-invasive corridor preserving normal anatomy. (C) Postoperative MRI demonstrating difference between diffuse and directed ablation. (A) Adapted with permission from Chautems et al. [Citation63]; (B, C) Unpublished property of Dr. Joseph Neimat.](/cms/asset/e4b80ea6-45e2-4b50-9016-cf7f9d9678b7/ihyt_a_1788175_f0002_c.jpg)
A more capable strategy could involve the use of steerable cannulas. To date, at least two techniques have been used to create steerable surgical probes that could be utilized to deliver thermal ablation. These techniques have dual advantages, (i) improved safety in avoiding blood vessels, ventricles and other key structures along the planned trajectory, and (ii) more complete ablation of structures that are linked with seizure freedom (amygdala, PHG, entorhinal cortex, hippocampus), if these key structures do not lend themselves to linear targeting (as is the case in many patients). Curved trajectories allow for more varied approaches to bypass important structures along the path and reach targets ‘around the corner’ or in other adjacent areas that are not reached by straight path navigation. Another advantage is multiple-targeting through a single insertion point. Recent research has focused on designing different systems for curved trajectories.
One mechanism for curved trajectories is magnetic steering in which a magnetic navigation system (MNS) external to the patient is used to steer probes with magnetic tips. This technology was originally used to navigate cardiac catheters in the 1980s [Citation57], soon followed by neurosurgical application [Citation58–60]. Early challenges included the need for a large MNS requiring considerable space, moving components impeding the integration of the device into clinical workflow and stress-relaxation of the needle tip. Advances in the manipulation of magnetic fields over the past three decades have enabled the development of new systems that have overcome many of these challenges. One system included development of a system with static electromagnets, thus avoiding the need for moving magnets [Citation61,Citation62]. Newly developed smaller mobile electromagnetic navigation systems eliminate the need for larger spaces or moving components, resulting in a seamless integration into clinical routines. Another focus has been on flexible catheters. To maximize the degrees of steering freedom, magnetically steerable catheters have been developed that consist of multiple segments with independent stiffness control () [Citation63]. Further, this technology permits the surgeon to carry out the operation remotely, which can protect the surgeon from radiation and in light of the current Covid-19 pandemic mitigate pathogen spread through reduced contact with the patient. Proof-of-concept studies have been completed for this technology, animal trials are underway. Together, these innovative systems permit accurate navigation of curved paths and can be remotely controlled to reduce radiation exposure and risk of infection to the surgeon and patient.
An alternate approach to the creation of curved, steerable cannulas is an established technique of using concentric flexible tubes with precise planar or helical curvatures. It has been demonstrated that such cannulas can be deployed with a ‘follow the leader’ technique that does not shear the surrounding tissue and achieves exceptional accuracy at deployments of up to 80 mm [Citation64,Citation65]. These strategies have the advantage that they can be driven by pneumatic actuators and adapted for use in an MRI environment.
These novel technologies open windows for new approaches, e.g., through transvascular [Citation66] or transforaminal approaches that can be used to reach the target for thermal ablation. Curved trajectory steering of the probe through natural cranial access points opens another level of minimal-invasiveness, preserving normal anatomy to a larger degree than the current methods (). Further work is needed to integrate these technologies into existing systems and test their translation into clinical surgery. The incorporation of such technologies may improve the control and accuracy of ablative therapies beyond the techniques of traditional surgery and result in more accurate ablation ().
Disclosure statement
JN consults for Monteris Inc., No other conflicts of interest are reported by the author(s).
Additional information
Funding
References
- Ojemann GA. Treatment of temporal lobe epilepsy. Annu Rev Med. 1997;48(1):317–328.
- Spencer DD, Spencer SS, Mattson RH, et al. Access to the posterior medial temporal lobe structures in the surgical treatment of temporal lobe epilepsy. Neurosurgery. 1984;15(5):667–671.
- Kwan P, Brodie MJ. Early identification of refractory epilepsy. N Engl J Med. 2000;342(5):314–319.
- Kanner AM, Ashman E, Gloss D, et al. Practice guideline update summary: efficacy and tolerability of the new antiepileptic drugs I: treatment of new-onset epilepsy. Epilepsy Curr. 2018;18(4):260–268.
- Wiebe S, Blume WT, Girvin JP, et al. A randomized, controlled trial of surgery for temporal-lobe epilepsy. N Engl J Med. 2001;345(5):311–318.
- Engel J, McDermott MP, Wiebe S, et al. Early surgical therapy for drug-resistant temporal lobe epilepsy: a randomized trial. JAMA – J Am Med Assoc. 2012;307(9):922.
- Rugg-Gunn F, Miserocchi A, McEvoy A. Epilepsy surgery. Pract Neurol. 2020;20(1):4–14.
- Niemeyer P, The transventricular amygdala-hippocampectomy in the temporal lobe epilepsy. In: Baldwin M, Bailey P, editors. Temporal lobe epilepsy. Springfield (IL): Charles C Thomas; 1958. p. 461–482.
- Yaşargil MG, Krayenbühl N, Roth P, et al. The selective amygdalohippocampectomy for intractable temporal limbic seizures: historical vignette. J Neurosurg. 2009;112(1):168–185.
- Hori T, Tabuchi S, Kurosaki M, et al. Subtemporal amygdalohippocampectomy for treating medically intractable temporal lobe epilepsy. Neurosurgery. 1993;33(1):50–56.
- Engel J. Update on surgical treatment of the epilepsies: summary of the second international palm desert conference on the surgical treatment of the epilepsies (1992). Neurology. 1993;43(8):1612–1617.
- Wieser HG, Blume WT, Fish D, Commission on Neurosurgery of the International League Against Epilepsy (ILAE) 1997–2001, et al. Proposal for a new classification of outcome with respect to epileptic seizures following epilepsy surgery. Epilepsia. 2008;42(2):282–286.
- Berg AT, Berkovic SF, Brodie MJ, et al. Revised terminology and concepts for organization of seizures and epilepsies: report of the ILAE Commission on Classification and Terminology, 2005-2009. Epilepsia. 2010;51(4):676–685.
- Jain P, Tomlinson G, Snead C, et al. Systematic review and network meta-analysis of resective surgery for mesial temporal lobe epilepsy. J Neurol Neurosurg Psychiatry. 2018;89(11):1138–1144.
- Wieser HG, Ortega M, Friedman A, et al. Long-term seizure outcomes following amygdalohippocampectomy. J Neurosurg. 2003;98(4):751–763.
- Lutz MT, Clusmann H, Elger CE, et al. Neuropsychological outcome after selective amygdalohippocampectomy with transsylvian versus transcortical approach: a randomized prospective clinical trial of surgery for temporal lobe epilepsy. Epilepsia. 2004;45(7):809–816.
- Hori T, Yamane F, Ochiai T, et al. Selective subtemporal amygdalohippocampectomy for refractory temporal lobe epilepsy: operative and neuropsychological outcomes. J Neurosurg. 2007;106(1):134–141.
- Wieser HG, Häne A. Antiepileptic drug treatment before and after selective amygdalohippocampectomy. Epilepsy Res. 2003;55(3):211–223.
- Josephson CB, Dykeman J, Fiest KM, et al. Systematic review and meta-analysis of standard vs selective temporal lobe epilepsy surgery. Neurology. 2013;80(18):1669–1676.
- Hu WH, Zhang C, Zhang K, et al. Selective amygdalohippocampectomy versus anterior temporal lobectomy in the management of mesial temporal lobe epilepsy: a meta-analysis of comparative studies A systematic review. J. Neurosurg. 2013;119(5):1089–1097.
- Schramm J. Temporal lobe epilepsy surgery and the quest for optimal extent of resection: a review. Epilepsia. 2008;49(8):1296–1307.
- Chengxiong W, Dingyang L, Zhiquan Y, et al. Clinical outcomes after medial temporal lobe epilepsy surgery: anterior temporal lobectomy versus selective amygdalohippocampectomy. J Cent South Univ (Medical Sci). 2018;43(6):638–645.
- Klein E, Fine S, Laor Y, et al. Aaronson, interaction of laser radiation with biologic systems. II. Experimental. Fed Proc. 1965;24(Suppl. 14):143–149.
- Fox JL, Hayes JR, Stein MN, et al. Experimental cranial and vascular studies of the effects of pulsed and continuous wave laser radiation. J Neurosurg. 2009;27:126–137.
- Krishnamurthy S, Powers SK. Lasers in neurosurgery. Lasers Surg Med. 1994;15(2):126–167.
- Brown TE, True C, McLaurin RL, et al. Laser radiation. II. Long-term effects of laser radiation on certain intracranial structures. Neurology. 1967;17(8 Pt 1):789–796.
- Rosomoff HL, Carroll F. Reaction of neoplasm and brain to laser. Arch Neurol. 1966;14(2):143–148.
- Stellar S, Polanyi TG. Lasers in neurosurgery: a historical overview. J Clin Laser Med Surg. 1992;10(6):399–411.
- Ascher PW, Heppner F. CO2-Laser in neurosurgery. Neurosurg Rev. 1984;7(2–3):123–133.
- Ognev BV, Vishnevskii AA, Troitskii RA, et al. Changes in the brain and eyes produced by lasers. Bull Exp Biol Med. 1972;73:205–207.
- Beck OJ, Wilske J, Schönberger JL, et al. Tissue changes following application of lasers to the rabbit brain - results with CO2 and neodymium-YAG lasers. Neurosurg Rev. 1979;2(1):31–36.
- Bown SG. Phototherapy of tumors. World J Surg. 1983;7(6):700–709.
- Brown SG. The future of lasers in cancer therapy. Br J Hosp Med. 1988;40(3):161.
- Sugiyama K, Sakai T, Fujishima I, et al. Stereotactic interstitial laser-hyperthermia using Nd-YAG laser. Stereotact Funct Neurosurg. 1990;54–55:501–505.
- Jolesz FA, Bleier AR, Jakab P, et al. MR imaging of laser-tissue interactions. Radiology. 1988;168(1):249–253.
- Patel P, Patel NV, Danish SF. Intracranial MR-guided laser-induced thermal therapy: single-center experience with the Visualase thermal therapy system. J Neurosurg. 2016;125(4):853–860.
- Harris M, Steele J, Williams R, et al. MRI-guided laser interstitial thermal thalamotomy for medically intractable tremor disorders. Mov Disord. 2019;34(1):124–129.
- Salem U, Kumar VA, Madewell JE, et al. Neurosurgical applications of MRI guided laser interstitial thermal therapy (LITT). Cancer Imaging. 2019;19(1):65.
- Drane DL, Loring DW, Voets NL, et al. Better object recognition and naming outcome with MRI-guided stereotactic laser amygdalohippocampotomy for temporal lobe epilepsy. Epilepsia. 2015;56(1):101–113.
- Dredla BK, Lucas JA, Wharen RE, et al. Neurocognitive outcome following stereotactic laser ablation in two patients with MRI-/PET + mTLE. Epilepsy Behav. 2016;56:44–47.
- Jermakowicz WJ, Kanner AM, Sur S, et al. Laser thermal ablation for mesiotemporal epilepsy: analysis of ablation volumes and trajectories. Epilepsia. 2017;58(5):801–810.
- Donos C, Breier J, Friedman E, et al. Laser ablation for mesial temporal lobe epilepsy: surgical and cognitive outcomes with and without mesial temporal sclerosis. Epilepsia. 2018;59(7):1421–1432.
- Drane DL. MRI-Guided stereotactic laser ablation for epilepsy surgery: promising preliminary results for cognitive outcome. Epilepsy Res. 2018;142:170–175.
- Talairach J, Bancaud J, Bonis A, et al. Functional stereotaxic exploration of epilepsy. Confin Neurol. 1962;22:328–331.
- Talairach J, Szikla G. Application of stereotactic concepts to the surgery of epilepsy. Acta Neurochir Suppl. 1980;30:35–54.
- Vladyka V. Taktika chirurgického lécení epilepsií a jejǐ uplatnění u temporálních epilepsií. Cesk Neurol Neurochir. 1978;41(2):95–106.
- Kratimenos GP, Pell MF, Thomas DGT, et al. Open stereotactic selective amygdalo-hippocampectomy for drug resistant epilepsy. Acta Neurochir (Wien). 1992;116(2–4):150–154.
- Patil AA, Andrews R, Torkelson R. Stereotactic volumetric radiofrequency lesioning of intracranial structures for control of intractable seizures. Stereotact Funct Neurosurg. 1995;64(3):123–133.
- Blume WT, Parrent AG, Kaibara M. Stereotactic amygdalohippocampotomy and mesial temporal spikes. Epilepsia. 1997;38(8):930–936.
- Parrent AG, Blume WT. Stereotactic amygdalohippocampotomy for the treatment of medial temporal lobe epilepsy. Epilepsia. 1999;40(10):1408–1416.
- Vojtěch Z, Malíková H, Krámská L, et al. MRI-guided stereotactic amygdalohippocampectomy: a single center experience. Neuropsychiatr Dis Treat. 2015;11:359–374.
- Willie JT, Laxpati NG, Drane DL, et al. Real-time magnetic resonance-guided stereotactic laser amygdalohippocampotomy for mesial temporal lobe epilepsy. Neurosurgery. 2014;74(6):569–585.
- Kang JY, Wu C, Tracy J, et al. Laser interstitial thermal therapy for medically intractable mesial temporal lobe epilepsy. Epilepsia. 2016;57(2):325–334.
- Shukla ND, Ho AL, Pendharkar AV, et al. Laser interstitial thermal therapy for the treatment of epilepsy: evidence to date. Neuropsychiatr Dis Treat. 2017;13:2469–2475.
- Wyler AR, Hermann BP, Somes G. Extent of medial temporal resection on outcome from anterior temporal lobectomy: a randomized prospective study. Neurosurgery. 1995;37(5):982–989.
- Wu C, Jermakowicz WJ, Chakravorti S, et al. Effects of surgical targeting in laser interstitial thermal therapy for mesial temporal lobe epilepsy: a multicenter study of 234 patients. Epilepsia. 2019;60(6):1171–1183.
- Yuan S, Holmqvist F, Kongstad O, et al. Long-term outcomes of the current remote magnetic catheter navigation technique for ablation of atrial fibrillation. Scand Cardiovasc J. 2017;51(6):308–315.
- McNeil RG, Ritter RC, Wang B, et al. Functional design features and initial performance characteristics of a magnetic-implant guidance system for stereotactic neurosurgery. IEEE Trans Biomed Eng. 1995;42(8):793–801.
- Grady MS, Howard MA, Broaddus WC, et al. Magnetic stereotaxis: a technique to deliver stereotactic hyperthermia. Neurosurgery. 1990;27(6):1010–1016.
- Grady MS, Howard MA, Molloy JA, et al. Nonlinear magnetic stereotaxis: three-dimensional, in vivo remote magnetic manipulation of a small object in canine brain. Med Phys. 1990;17(3):405–415.
- Petruska AJ, Ruetz F, Hong A, et al. “Magnetic needle guidance for neurosurgery: Initial design and proof of concept,” 2016 IEEE International Conference on Robotics and Automation (ICRA), Stockholm, 2016, 4392–4397. DOI:10.1109/ICRA.2016.7487638.
- Hong A, Boehler Q, Moser R, et al. 3D path planning for flexible needle steering in neurosurgery. Int J Med Robot Comput Assist Surg. 2019;15(4):e1998.
- Chautems C, Tonazzini A, Boehler Q, et al. Magnetic continuum device with variable stiffness for minimally invasive surgery. Adv Intell Syst. 2020;2(6):1900086.
- Webster RJ, Kim JS, Cowan NJ, et al. Nonholonomic Modeling of Needle Steering. The International Journal of Robotics Research. 2006;25(5–6): 509–525. DOI:10.1177/0278364906065388
- Gilbert HB, Neimat J, Webster RJ. Concentric tube robots as steerable needles: achieving follow-the-leader deployment. IEEE Trans Robot. 2015;31(2):246–258.
- Watanabe H, Takahashi H, Nakao M, et al. Intravascular neural interface with nanowire electrode. Electron Commun Jpn. 2009;92(7):29–37.