Abstract
Background & aims
To explore the ability of gadolinium-ethoxybenzyl-diethylenetriamine pentaacetic acid magnetic resonance imaging (EOB-MRI)/ultrasound (US) fusion imaging (FI) to improve the prognosis of radiofrequency ablation (RFA) by ablating the characteristic findings of hepatocellular carcinoma (HCC) in hepatobiliary phase (HBP) imaging.
Methods
We retrospectively recruited 115 solitary HCC lesions with size of (15.9 ± 4.6) mm. They were all treated by RFA and preoperative EOB-MRI. According to the modalities guiding RFA performance, the lesions were grouped into contrast enhanced US (CEUS)/US guidance group and EOB-MRI/US FI guidance group. For the latter group, the ablation scope was set to cover the HBP findings (peritumoral hypointensity and irregular protruding margin). The presence of HBP findings, the modalities guided RFA, the recurrence rate were observed.
Results
After an average follow-up of 377 days, local tumor progression (LTP) and intrahepatic distant recurrence (IDR) were 14.8% and 38.4%, respectively. The lesions having HBP findings exhibited a higher recurrence rate (73.7%) than the lesions without HBP findings (42.9%) (p = 0.002) and a low overall recurrence-free curve using the Kaplan-Meier method (p = 0.038). Using EOB-MRI/US FI as guidance, there was no difference in the recurrence rate between the groups with and without HBP findings (p = 0.799). In lesions with HBP findings, RFA guided by EOB-MRI/US FI (53.8%) produced a lower recurrence rate than CEUS/US (84.0%) (p = 0.045).
Conclusions
The intraprocedurally application of EOB-MRI/US FI to determine ablation scope according to HBP findings is feasible and beneficial for prognosis of RFA.
Introduction
Surgical resection and liver transplant are recommended in recent guideline as curative first line therapies for hepatocellular carcinoma (HCC) [Citation1,Citation2]. However, image-guided percutaneous radiofrequency ablation (RFA) is becoming a preferable treatment option for a much wider HCC population [Citation3] due to the harsh conditions required for the therapy of surgical resection (solitary small lesion with good liver function) and liver transplant (transplant donors). Encouragingly, thanks to its potential advantages of a relatively low level of invasiveness, ease of use, and effectiveness [Citation4], the value of image-guided percutaneous RFA has recently become more widely recognized by many researchers. A recent randomized clinical trial showed that total post-operative complications, infections, and hospital stays of the RFA group were significantly lower than those of the liver resection group (p < 0.001). In addition, their recurrence and overall survival rates were comparable to the liver resection group [Citation5]. Nevertheless, the reported overall recurrence within 5 years after RFA and local recurrence at RFA sites of HCC patients was as high as 70% and 36%, respectively, which is alarming [Citation6,Citation7]. It is believed that this high recurrence rate is closely related to micro-vascular invasion (MVI). MVI indicates the presence of tumor emboli in the microscopic vessels in close proximity to the primary tumor, and is an early expression of tumor expansion [Citation8]. HCC has a tendency to invade the portal vein by microscopic tumor emboli, leading to an intrahepatic diffuse spread [Citation8]. The diagnosis of MVI is based on histopathology. It is difficult to preoperatively detect [Citation9], and it is also rare to treat it successfully [Citation8].
Compared with other imaging modalities, Gadolinium-ethoxybenzyl-diethylenetriamine pentaacetic acid magnetic resonance imaging (EOB-MRI) has an inherently high image resolution of MRI and unique hepatobiliary phase (HBP) imaging, which increase the high lesion-to-liver contrast and give additional diagnostic information through the hepatocyte uptake contrast agent [Citation10]. Recently, EOB-MRI has been recommended by several guidelines as the primary or first-line diagnostic imaging modality for the noninvasive diagnosis of HCC [Citation10,Citation11]. It is of utmost importance that, when using the pathology findings as the reference standard, the findings of peritumoral hypointensity and the irregular protruding margin in the HBP of EOB-MRI (in the following, we abbreviate them to ‘HBP findings’) are used to preoperatively predict the MVI of HCC; the HBP findings are supposed to be associated with an early recurrence of surgical resection and RFA [Citation12–15].
US related technology has been the most widely used imaging modality for the guidance of RFA of hepatic tumors [Citation16], mainly making use of its merits as a real-time display the operation, thus improving the safety and efficiency of RFA treatment. Intraprocedurally contrast-enhanced MRI and US fusion imaging (FI) can simultaneously display contrast-enhanced MRI and US, making comprehensive use of the advantage of real-time of US and a high resolution of MRI. Some studies have described the pros and cons of MRI/US FI and CEUS/US in guiding RFA [Citation17–19]. To date, however, no research has been done to comparatively evaluate which modality is better for guidance during RFA. More importantly, although EOB-MRI/US FI has been reported by some articles to have the ability to guide interventional operations for HCC, especially for small or atypical HCC lesions [Citation20,Citation21], regrettably, to the best of our knowledge, no study has focused on ablating the valuable prognosis-related HBP findings.
Inspired by the reported positive correlation between HBP findings and the prognosis of HCC treatment, we naturally wondered the feasibility of ablating HBP findings and its impact on recurrence. The purpose of our study was to further confirm the prognostic value of HBP findings on the basis of previous HBP studies, and most importantly, to investigate the feasibility and effectiveness of intraprocedurally EOB-MRI/US FI-guided ablation of HBP findings. We expected to make a step forward in improving the poor outcomes of HCC after RFA.
Materials and methods
Patient enrollment
This retrospective study was approved by the institutional review board of our hospital, and informed consent was obtained from all enrolled patients. From January 2015 to December 2019, 115 patients with pathologically diagnosed HCC were enrolled. The diagnosis of HCC were obtained by biopsy specimen, and was based on the criteria of the International Working Party [Citation22] and International Consensus Group for Hepatocellular Neoplasia (ICGHN) [Citation23]. The histological grade classification was made according to the research of Wang et al. [Citation24]. For the patients who participated in this study, the choice of method to guide RFA treatment was left to the senior hepatologists (K.N., K.O. and H.F.) who performed the RFA operation. They were fully informed of the advantages and disadvantages of EOB-MRI/US FI and CEUS/US, such as the real-time characteristic of CEUS, the high resolution of EOB-MRI, and the cost of CEUS at our institution.
All patients/lesions enrolled met the following criteria and intended to receive RFA in our department. (I) EOB-MRI examination of the liver was undertaken within two weeks before the RFA performance; (II) A percutaneous RFA treatment guided by US related imaging could be performed if feasible and safe; (III) Underlying hepatitis or cirrhosis was classified as Child-Pugh grade A or B; (IV) A 5 mm distance from the ablation margin to the lesion could be obtained; (V) Solitary tumor, maximum diameter ≤5 cm, and untreated. Patients/lesions with the following characteristics were excluded from this study: (I) Patients who were unable to undergo EOBMRI due to contraindication for the use of intravenous contrast agents (allergic reaction or impaired renal function); (II) Patients who had previous treatment histories of transcatheter arterial chemoembolization (TACE), percutaneous ethanol injection therapy (PEIT), stereotactic body radiotherapy (SBRT), or hepatic resection for HCC; (III) The ablation scope could not reach 5 mm because of difficult-to-treat HCC (close to the diaphragm or large blood vessels, much too superficially or deeply located); (IV) Multiple HCC lesions; (V) Lesions undetectable on conventional US; (VI) Child-Pugh score system classified as grade C; and (VII) LTP and IDR were detected simultaneously during follow-up.
The baseline clinical data of patients (gender, age, associated liver disease, Child-Pugh grade, and alpha-fetoprotein (AFP)), baseline imaging data of lesions (segmental location and size), and pathohistological diagnosis were retrospectively collected from the electronic medical record system, the radiology database, and pathology reports, respectively, of our hospital.
Preoperative evaluation of the HBP findings by EOB-MRI
For all the patients, within two weeks before RFA, EOB-MRI was performed and the HBP findings were determined according to the axial HBP images of EOB-MRI.
All patients were examined using a 1.5 T MR scanner (Avant; Siemens Medical System, Erlangen, Germany) with an 18-channel phased-array body coil and an integrated spine coil with 12 channels. Patients were asked to fast for 6–8 h before MR examination. The routine MR imaging sequences included in the standardized scanning protocol were high-spatial-resolution transverse T2-weighted imaging, coronal T2-weighted imaging, and T1-weighted in and opposed phase imaging. After pre-enhanced scanning with liver acquisition with volume acceleration (LAVA) with fat saturation, an EOB agent (Primovist®, Bayer Schering Pharma, Berlin, Germany) at a dose of 0.1 mmol/kg was injected at a rate of 1 mL/s through a catheter placed in the antecubital vein, followed by flushing with 20 mL of sterile saline flush. Arterial phase (AP), portal phase (PP), and late phase scanning were performed at 25–30, 70–85, and 180 s after initiation of the contrast injection, respectively. After initiation of the contrast injection, HBP scanning was performed at 20 min post injection. Unenhanced and HBP images were obtained using a T1-weighted high-resolution sequence of LAVA (TR/TE = 6.2/3.15 ms; flip angle, 20°; bandwidth, 260 Hz/pix; matrix, 166 × 320) in a single breath hold (18–20 s).
The HBP findings in this study were defined as the abnormal appearance of peritumoral hypointensity and irregular protruding margin in HBP of EOB-MRI (). Peritumoral hypointensity was defined as a relatively hypointense area compared with the surrounding liver parenchyma, located outside the tumor margin, on the hepatobiliary images [Citation23]. As the signal intensity of this area was slightly different from the tumor and the surrounding liver parenchyma, it could be distinguished from the surrounding tissue and tumor. Irregular protruding margins indicated a local (not the entire) non-smooth margin at the periphery of the tumor, protruding like a minute budding portion [Citation14]. In view of the relative subjectivity of the interpretation of HBP findings, taking images of other phases (plain scan, AP, and diffusion weighted imaging) as reference, two radiologists with more than five years of experience (M.C. and S.M.) in imaging diagnosis of the liver read the hepatobiliary images by consensus discussion. Any discrepancies in imaging features were resolved by a third reviewer (M.O., with 20 years of experience in liver imaging). It is crucial to note that the ablation margin in our routine RFA practice is not less than 5 mm. In that case, if the length of the HBP finding is less than 5 mm, regardless of using MRI or not, we can still ablate the HBP findings. With this in mind, we determined that only HBP findings with a maximum measurable diameter of more than 5 mm were meaningful, and were therefore included in the subsequent analysis.
Figure 1. Characteristic findings of hepatocellular carcinoma (HCC) in a hepatobiliary phase (HBP) image using gadolinium-ethoxybenzyl-diethylenetriamine pentaacetic acid magnetic resonance imaging (EOB-MRI). (a) Fusion imaging combining a grayscale ultrasound (left side) and HBP image of EOB-MRI (right side). In the transverse HBP image, the HCC lesion has local irregular protruding margins, which seem to be budding portions at the periphery of the lesions. (b) Fusion imaging combining a grayscale ultrasound (left side) and HBP image of EOB-MRI (right side). HCC lesions with the appearance of peritumoral hypointensity in the HBP image, which show as a hypointense area of hepatic parenchyma located outside of the tumor margin. They usually appear in the plane where the lesion is not at the maximum diameter, and in various shapes, such as wedge-shaped or flame-like. We can see that the ultrasound image may not clearly show the hypointense area, while HBP images do. The arrows and arrowheads shown in all the images indicate the HBP findings and the border of the lesions, respectively. These lesions are not adjacent to the portal vein or hepatic vein. Lesion size and segmental location are recorded as follows: (a) S3, 22×20 mm; (b) S4, 15×13 mm.
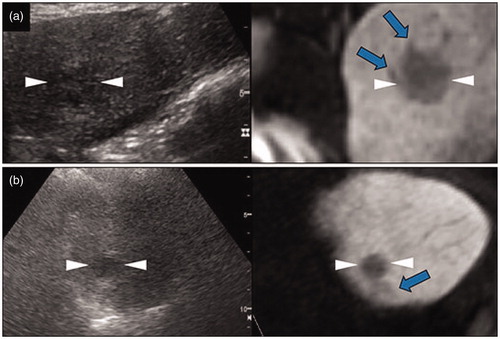
US imaging examination
For all the enrolled patients, the imaging characteristics of the HCC lesions (size, shape, echogenicity, location, margin, and blood flow) were assessed by conventional US using the LOGIQ E9 US system (GE Healthcare, Ltd. Chicago, IL). A convex probe with a frequency of 1–6 MHz and a micro-convex probe with a frequency of 2–5 MHz were used.
The operation steps of contrast enhanced ultrasound (CEUS) examination were in accordance with the guidelines of clinical practice of CEUS using Sonazoid [Citation25]. In short, for patients assigned to use CEUS for RFA guidance, intraprocedurally CEUS with Sonazoid was performed. A 0.2 mL dose of perfluorobutane microbubbles (Sonazoid®, Daiichi Sankyo, Tokyo, Japan) was injected into an antecubital vein at 0.2 mL/s via a 24-gauge cannula, followed by 2 mL of 5% glucose. CEUS images were acquired and evaluated during three contrast phases, consisting of AP (10–50 s after initiation of the injection), PP (80–120 s after initiation of the injection), and post-vascular phase (PVP) (10 min after initiation of the injection).
Intraprocedurally application of FI
After confirming the HBP findings with the radiologist (M.C. or S.M., randomly), EOB-MRI/US FI was performed by one of the three senior hepatologists (K.N., K.O. and H.F.). As previously reported [Citation18], the FI system is composed of a position-sensing unit mounted on a LOGIQ E9 or E10 unit (GE Healthcare, Ltd. Chicago, IL), a position sensor internally equipped with a US probe, and a magnetic field transmitter which is located 20–30 cm from the patient so that the area being scanned is within the range of the transmitter. When the position sensor is moved into the magnetic field, an induced electric current is generated, allowing the system to recognize its 3 D spatial position and orientation. The image fusion procedure began with the importation of DICOM data from a previous MR examination. Next was the planning phase, registering the US image with the pre-acquired volumetric EOB-MRI DICOM data. Registration was achieved by manually defining common anatomic planes. First, the transducer was placed parallel to the MR volume data axial slices; second, certain common anatomic landmarks, such as the bifurcation of vessels, cysts, and calcifications, were identified and marked in the real-time US image and the HBP image of the EOB-MRI. Using the overlay function of the FI technology for regulation and verification, the displacement between images of lesions was required to be within 2 mm. When accurate image matching was obtained, real-time US and fused HBP images of EOB-MRI appeared side-by-side or overlaid on the US monitor. Therefore, the fused EOB-MRI showed the same plane and moved synchronously with the real-time US. Furthermore, we used the global positioning system (GPS) tool, a subset of the VNav System, to make marks. The marks confirmed the location of the HCC and identified the margin of the treated HCC.
RFA procedure
All patients received local anesthesia and analgesia before the procedure. RFA was performed with the guidance of real-time CEUS/US or EOB-MRI/US FI by one of the three senior hepatologists (K.N., K.O. and H.F.), each with more than 20 years of experience in interventional techniques. In this study, ‘CEUS/US’ refers to the operating RFA in the process of CEUS examination (especially in PVP), with CEUS and US appearing side-by-side on the US monitor in real time. The operation steps of RFA were taken from Nishigori S’s study [Citation21]. RFA was performed using a 480 kHz generator (VIVA RF generator; STARmed, Gyeonggi, Korea), capable of producing a maximum power of 200 W and a specific size of 17-gauge internally cooled, adjustable RF electrode (Proteus; STARmed, Gyeonggi, Korea). The lengths of the active tip of the electrodes were 5 mm, 1.0 cm, 1.5 cm, 2.0 cm or 3.0 cm. Each electrode was selected based on tumor size, tumor location, and operator preference. Briefly, for patients with a tumor diameter of 2 cm or smaller, an electrode with a 2 cm tip was inserted into the tumor and ablated at 20 W. For patients with a tumor diameter larger than 2 cm, an electrode with a 3-cm tip and ablation at 40 W was selected. For nodules with a relatively larger size (3–5 cm), the electrode was inserted at different sites. In general, the first puncture of RFA targeted the center of the largest section of the lesion shown on the US or radiological images. Notably, based on our experience in exploring HBP findings in this study, we found that the HBP findings did not usually appear on the plane with the largest lesion size. In view of this, for the lesions both with HBP findings and treated by EOB-MRI/US FI guided RFA, the purpose of the first puncture of RFA was to ablate the HBP findings. Therefore, the target was aimed at the plane where the HBP findings could be displayed clearly, rather than the largest section of the lesion. The mean duration of one ablation was 12 min, and the temperature of the ablated tissue was maintained above 60 °C. Immediately after ablation, the therapeutic response to RFA was evaluated by CEUS or FI to determine the adequacy of ablation, and whether additional ablation was needed. For RFA performed under the guidance of CEUS/US, post-operative CEUS examination was undertaken to see if the edge of the ablation area showed hypervascularity in AP. A complete ablation could be confirmed by no perfusion of contrast agent into the tumor as a whole, showing a ‘cavity’ appearance with a distinct boundary. For RFA treatment guided by EOB-MRI/US, the overlap function of FI was applied to observe whether the ablative area completely covered the lesion area shown on pre-operative EOB-MRI images. After ablation, the needle was retracted, maintaining its tip hot in order to prevent, by thermal coagulation, seeding or hemorrhage along the electrode track. Every procedure was aimed at obtaining a no less than 5 mm safety margin around the treated lesions.
Follow-Up
The disease recurrence patterns were classified by local tumor progression (LTP) () and intrahepatic distant recurrence (IDR) (). LTP is defined as the development of a tumor contiguous to or within the initial ablative treated area. IDR is defined as the emergence of new lesions in other segments, or in the same segment more than 2 cm away from the treated area [Citation26]. To detect recurrence, all patients were followed every two months by CEUS, EOB-MRI, or CECT examination in the first year after RFA. Then, the follow-up interval was prolonged to every three months after the first year. Recurrent tumors were typically diagnosed by the following imaging features: arterial hypervascularity and wash-out in the early or delayed venous phase in CEUS, EOB-MRI, or CECT (LTP or IDR), and/or irregular thickening at the periphery of the ablation cavity in CT or MRI (LTP). When LTP or IDR was detected, the follow-up of the lesion was terminated.
Figure 2. The local tumor progression (LTP) of a HCC lesion after radiofrequency ablation (RFA). (a) A hepatobiliary phase image of EOB-MRI shows the peritumoral hypointensity appearance (arrow). (b, c) Contrast enhanced ultrasound examination using a Sonazoid agent. The arterial phase image (b) exhibits a washed in appearance, while the post-vascular phase image (c) shows a washed out appearance. (d) Pre-operative fusion imaging combining a grayscale ultrasound (left side) and the hepatobiliary phase image of EOB-MRI as a reference (right side) on a single screen. Arrowheads in (a–d) indicate the margin of the lesion. (e) The grayscale ultrasound image shows the ablated area (arrowheads). (f) Portal phase of the contrast-enhanced CT image obtained four months after RFA shows the ablate area (arrowheads) and adjacent low density area (arrow), which suggests LTP. This lesion is from a 78 year-old male patient and was pathologically confirmed as poorly differentiated HCC (maximum diameter 23 mm) in segment VII.
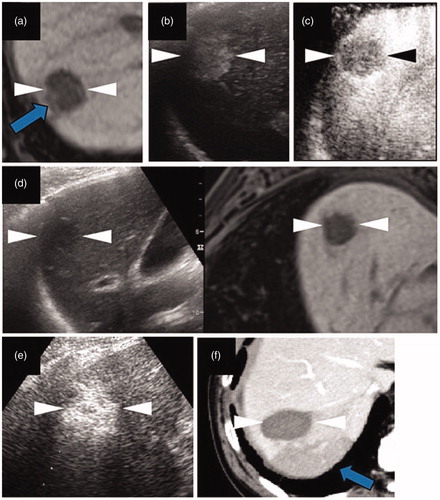
Figure 3. Intrahepatic distant recurrence (IDR) of a HCC lesion after radiofrequency ablation (RFA). (a–c) Axial T1-weighted image on a plain scan, the arterial phase, and the hepatobiliary phase (HBP) of EOB-MRI, respectively. The lesion is 27 mm in segment VI/VII. Arrows (a–c) indicate the local irregular protruding margins. (d, e) Contrast enhanced ultrasound examination using a Sonazoid agent. The arterial phase image (d) exhibits a washed in appearance, while the post-vascular phase image (e) shows a distinct washed out appearance. (f) An HBP image of EOB-MRI obtained 3 months after RFA shows adequate necrosis (arrowheads). (g) Fusion imaging combining a grayscale ultrasound (left side) and contrast-enhanced CT image (right side) on a single screen, showing an IDR lesion at the half-year follow-up examination. Both the grayscale ultrasound and contrast-enhanced CT images show an IDR lesion of about 13 mm in size in segment VII. Arrowheads (a–e) and (g) indicate the margin of the original lesion and IDR lesion, respectively. This case comes from a 76 year-old female patient with chronic C hepatitis. The original lesion and the IDR lesion were pathologically confirmed to be poorly and moderately differentiated HCCs, respectively.
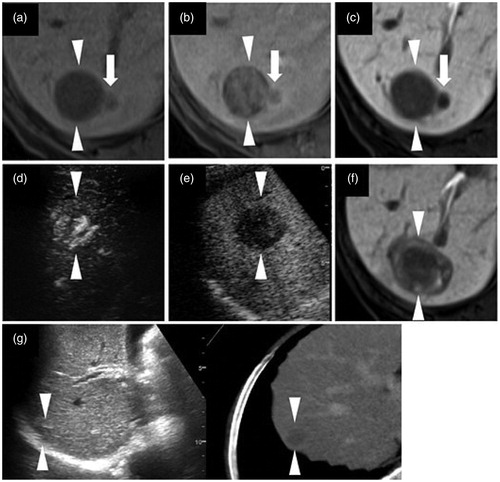
Statistical analysis
Statistical analysis was performed using SPSS software (version 26.0; SPSS Inc., Chicago, IL, USA). Continuous variables (age, follow-up period, lesion size, and serum AFP levels) were presented as the median and standard deviation. Descriptive statistics, including the comparison among the LTP, IDR and no recurrence groups, were analyzed by one-way analysis of variance (ANOVA). Categorical variables (the distribution frequencies of gender, etiology, location of the lesion, Child-Pugh classification, histological grade, ways to guide RFA, and HBP findings), were tested by the chi-square test or Fisher exact test. Overall non-recurrence rates were computed using the Kaplan-Meier method. Comparisons were made between the groups with and without HBP findings using the log-rank test. A two-tailed P value of less than 0.05 was considered statistically significant.
Results
Baseline characteristics
elucidates the baseline characteristics of the study population with LTP, IDR, and no recurrence. For all the lesions, the mean and range of size, which was defined by the maximum diameter measured in the US images, was (15.9 ± 4.6) and (6, 33) mm, respectively. The mean follow-up time was 376.7(21–1329) days. Of the 115 HCC patients, 17 and 44 patients encountered LTP and IDR, respectively, whereas 54 patients had no recurrence. There were no significant differences in the baseline characteristics of the patients (age, sex, etiology of HCC, Child-Pugh classification, AFP levels) and lesions (location, size) among the three groups (p > 0.05). According to the histological classification of the included lesions, it was found that there was a significant difference in the incidence of LTP, IDR and no recurrence between histologically early HCC lesions and advanced ones (p = 0.012, ).
Table 1. Baseline characteristics of patients and HCC lesions.
Comparison of recurrence in terms of HBP findings and guidance of RFA
Of the 115 patients, 64 and 51 were successfully treated by RFA under CEUS/US guidance and EOB-MRI/US FI guidance, respectively. The HBP findings were statistically different based on prognosis regarding LTP, IDR and no recurrence (p = 0.002), and methods of guiding RFA (p = 0.049) (). The overall recurrence rate for groups with and without HBP findings was 73.7% (28/38) and 42.9% (33/77), respectively; for the groups using EOB-MRI/US FI and CEUS/US for guidance, it was 56.9% (29/51) and 50.0% (32/64), respectively ().
Table 2. The impact of HBP findings and methods of guiding RFA on the type of recurrence.
Table 3. The impact of the HBP findings of HCC and method of guiding RFA on recurrence.
We further undertook a detailed subgroup analysis. In the group of RFA guided by EOB-MRI/US FI, the recurrence rate revealed no difference between the lesions with and without HBP findings (p = 0.799), which suggested that with the help of EOB-MRI, the adverse effects of HBP findings on recurrence could be improved or even offset. In the group of RFA guided by CEUS/US, the lesions without HBP findings have a better prognosis, which was suggested by the findings that the non-recurrence rate of patients without HBP findings (28/39, 71.7%) was much higher than those with HBP findings (4/25, 16.0%) (p = 0.002, ). Regardless of whether RFA is guided by EOB-MRI/US FI or not, HBP findings have an effect on the prognosis, which was demonstrated by different recurrence rates between groups with and without HBP findings (p = 0.002) (). This overall statistical significance may indicate the HBP findings of CEUS/US guided group has too great influence on the prognosis (p < 0.001). When CEUS/US and EOB-MRI/US groups were taken together into statistics, the adverse effect still existed.
As eHCCs and adHCCs showed differences in terms of recurrence (, p=0.012), we conducted a subgroup analysis according to histological grade. In adHCCs, the lesions with HBP findings had a higher recurrence rate (25/30, 83.3%) than those without HBP findings (24/49, 49.0%) (p = 0.002, ).
Prediction of overall recurrence risk
depicts the recurrence-free survival rate of groups with and without HBP findings using Kaplan-Meier curves. A statistical difference (p = 0.039) was observed between the two groups. Lesions with HBP findings have a worse recurrence progression.
Discussion
In the present study, the indifference of some baseline character analysis ensured that the possible risk factors of recurrence after RFA (large lesion size, tumor multifocality, elevated serum AFP level, young patient age and high Child-Pugh class) [Citation3,Citation27] would not affect our final prognosis. Our study revealed that eHCCs have a lower rate of overall recurrence (33.3%) than adHCCs (62.0%). The difference lies in both the LTP (eHCC: 5.6%, adHCC: 19.0%) and IDR (eHCC: 27.8%, adHCC: 43.0%). We conducted a subgroup analysis according to histopathology to compare the prognosis. As expected, we found that for adHCCs, the overall recurrence rate of lesions with HBP findings was significantly different from those without. Interestingly, however, for eHCCs, there was no significant difference in the overall recurrence rate between lesions with (37.5%) and without (32.1%) HBP findings (p = 0.777). This divergence may be due to the fact that, compared with adHCC, eHCC is characterized by a vague margin and relatively small size [Citation28]. Therefore, the HBP findings are not easy to detect in eHCCs. In addition to the relatively larger size and distinct fibrous capsule of adHCCs, which are advantageous to the detection of HBP findings, the changes in biological behavior of adHCCs also play a role. As eHCCs spread, they do not displace or destroy the surrounding vascular structures, but replace the surrounding parenchyma [Citation29]. On the other hand, adHCCs are thought to progress through vascular invasion and intrahepatic metastasis [Citation29]. As histological differentiation advances, the incidence of MVIs increases [Citation30]. In this regard, HBP findings may be both easy-to-observe and effective imaging indicators to predict the prognosis of adHCCs, rather than eHCCs.
As expected, the overall recurrence rate of lesions with HBP findings (73.7%) was much higher than those without (42.9%), confirming the reported view that the presence of HBP findings is harmful to the prognosis of HCC [Citation12,Citation14]. Specifically, in our study, both LTP and IDR were higher in lesions with HBP findings (LTP: 23.6%, IDR: 50.0%) than those without (LTP: 10.4%, IDR: 32.5%). The reported LTP rates varied from 2% to 36% [Citation31]. Consistent with this, our study exhibited an LTP rate of 14.8% for all enrolled patients, and 5.6% and 19.9% for eHCC and adHCC patients, respectively. To note, LTP and IDR, as two recurrence patterns of HCC were reported to have different pathogenesis. IDR either results from a new tumor developed from cirrhosis or tumor metastases via the intrahepatic portal venous system [Citation32]. According to the General Rules for the Clinical and Pathological Study of Primary Liver Cancer, IDR lesions have the components of portal vein tumor thrombi, or the tumors are growing in apparent contiguity with these thrombi [Citation33]. In short, the occurrence of IDR is closely related to MVI. Quite differently, LTP is caused by untreated viable tumor tissues [Citation32]. We therefore speculate that LTP lesions may occur not only in the MVI in the blood vessels around the tumor, but also in the peritumoral microsatellite foci that have not been treated during RFA surgery. Microsatellite foci are not easy to find on imaging, and have the risk of a poor prognosis. They are distributed around the larger primary tumors and have the same tumor clonality as the primary lesions [Citation33]. Obviously, the results of LTP and IDR shown in this study have further confirmed the relationship between HBP findings and MVI, as the previous literature described [Citation12,Citation14]. More importantly, due to the mechanism of LTP occurrence and our findings, we put forward a new hypothesis of the potential relationship between HBP findings and microsatellite foci. The HBP findings may suggest the presence of microsatellite foci, thus leading to poor LTP.
At present, FI technology has been evidenced to be effective for RFA of HCCs through three widely recognized functions. Firstly, in locating the lesions that are poorly defined on conventional US and CEUS because of a poor acoustic window [Citation34]. Our included lesions were all visible by conventional US. In this setting, the FI in this study will not exhibit this effect for RFA. Secondly, in helping intra-operatively evaluate the large blood vessels proximate to the lesions. Heat from ablation may result in possible damage to adjacent large vessels. What is worse, the ‘heat sink’ effect caused by adjacent large vessels absorbing heat from ablation may lead to an incomplete ablation, and thus a poor recurrence outcome [Citation35]. Based on this consideration, we made a careful pre-operative plan for the safety and feasibility of the RFA treatment, and recruited lesions for RFA treatment on the premise of a 5 mm ablation margin free of peritumoral large blood vessels. Hence, compared with CEUS/US guidance, FI did not show much advantage in intra-operative displaying of peritumoral large blood vessels. Last but not the least, the most critical role of FI is in determining the ablation scope before, during, and immediately after the operation, to achieve complete ablation. In this study, for lesions with HBP findings, using EOB-MRI/US FI to guide RFA leads to lower overall recurrence (53.8%) compared to using CEUS/US (84.0%). In line with this, when using EOB-MRI/US FI to guide RFA, there is no difference in the overall recurrence rate between lesions with (53.8%) and without (57.9%) HBP findings (p > 0.05). These results in concert suggest that intraprocedurally ablating of the HBP findings by EOB-MRI/US FI can offset the adverse prognostic effect cause by MVI, and even microsatellite foci. For lesions with HBP findings, the positive effect of EOB-MRI/US FI guidance much outperformed US/CEUS guidance (p = 0.045). A few recent studies undertook primary investigations of the relationship between tumoral irregular defects in PVP image of CEUS and MVI, and further predicted the impact of PVP images on the recurrence of HCC after surgical resection and RFA [Citation36]. Unfortunately, although they used the same log-rank test of survival curve as our study did, no significant difference in overall recurrence rate was detected between the irregular-defect and non-irregular-defect groups (surgical resection: p = 0.427, RFA: p = 0.293) [Citation36,Citation37]. The poor prognosis results of US/CUES in our study and literature may possibly due to the fact that CEUS is not as sensitive as EOB-MRI in detecting MVI-related images. Although the unique PVP of Sonazoid CEUS and the novel technology of re-injection in PVP play an important role in improving the diagnosis of HCC, PVP of Sonazoid CEUS still cannot avoid the shortcomings of US. That is, the parenchyma background of cirrhosis, intestinal gas, abundant fatty tissue, and depth, which can adversely affect the recognition of image details [Citation25]. With respect to these findings, we concluded that when MVI and microsatellite foci exist, the real tumor scope in RFA performance might be underestimated by US/CEUS because a lack of sensitivity to detect the margin and peritumoral imaging. Nevertheless, a comparative study between CEUS and EOB-MRI based on homogeneous large samples is needed to further verify this point of view.
As we described, in patients using CEUS/US to guide RFA, the post-operation overall recurrence rate is markedly higher (84.0%) in lesions with HBP findings than those without (28.2%). From this perspective, CEUS/US-guided RFA appears to be useless in improving the poor recurrence caused by MVI and/or microsatellite foci. However, it is obviously wrong to conclude that the role of US-related technology in guiding RFA is inferior to that of EOB-MRI/US. Interestingly, when no HBP findings are presented, the overall recurrence rate of US/CEUS guided RFA was lower (28.2%) than that of EOB-MRI/US FI (57.9%). This result confirms the multiple benefits of CEUS in monitoring the RFA procedure. CEUS can reflect the real-time micro-vascular blood perfusion of the ablation zone [Citation38], detect the residual enhancing tumor immediately after ablation [Citation39], and enjoys perfect image matching between US and CEUS. In contrast, FIs of radiologic and US modalities, including EOB-MRI/US, have a slight but unavoidable registration error that may decrease the accuracy of ablation [Citation18].
Certain limitations must be considered when interpreting the results of our study. Firstly, due to the short follow-up time, almost all the patients were still alive at follow up. Therefore, we did not calculate the survival rate, which is as important as the recurrence rate in understanding prognosis. Secondly, there was a statistical difference in the follow-up period between the recurrent group and the non-recurrent group (p = 0.001). Because the follow-up terminated once the recurrence was detected, the follow-up period of the recurrence group was shorter. Thirdly, as mentioned above, there may be registration errors in the FI technique, which unavoidably exert adverse influence on the accuracy of the ablation scope and outcome of RFA. Registration errors are possibly produced from the different patient status between the reference MRI (obtain during breath-hold) and real-time working US images (obtain when free-breathing) [Citation18].
Conclusion
In summary, the findings in current research were in line with previous ones suggesting a role for HBP findings in the prognosis of HCC. The application of EOB-MRI/US FI in guiding ablation of solitary HCC lesions less than 4 cm seems to be feasible, effective and improves the outcome of the lesions with HBP imaging findings compared to using CEUS only.
Disclosure statement
No potential conflict of interest was reported by the author(s).
References
- Omata M, Cheng A, Kokudo N, et al. Asia-Pacific clinical practice guidelines on the management of hepatocellular carcinoma: a 2017 update. Hepatol Int. 2017;11(4):317–370.
- Liver EAFT. EASL clinical practice guidelines: management of hepatocellular carcinoma. J Hepatol. 2018;69(1):182–236.
- Kang TW, Lim HK, Cha DI. Aggressive tumor recurrence after radiofrequency ablation for hepatocellular carcinoma. Clin Mol Hepatol. 2017;23(1):95–101.
- Hirooka M, Ochi H, Koizumi Y, et al. Local recurrence of hepatocellular carcinoma in the tumor blood drainage area following radiofrequency ablation. Mol Clin Oncol. 2014;2(2):182–186.
- Gong Y, Tang Y, Geng Y, et al. Comparative safety and effectiveness of ultrasound-guided radiofrequency ablation combined with preoperative three-dimensional reconstruction versus surgical resection for solitary hepatocellular carcinoma of 3–5 cm. J Cancer. 2019;10(22):5568–5574.
- Ganne-Carrie N, Nault JC, Ziol M, et al. Predicting recurrence following radiofrequency percutaneous ablation for hepatocellular carcinoma. Hepat Oncol. 2014;1(4):395–408.
- Harrison LE, Koneru B, Baramipour P, et al. Locoregional recurrences are frequent after radiofrequency ablation for hepatocellular carcinoma. J Am Coll Surg. 2003;197(5):759–764.
- Reginelli A, Vacca G, Segreto T, et al. Can microvascular invasion in hepatocellular carcinoma be predicted by diagnostic imaging? A critical review. Future Oncol. 2018;14(28):2985–2994.
- Kawaguchi T, Shimose S, Torimura T. Challenges and prospects in prediction and treatment for hepatocellular carcinoma with microvascular invasion. Hepatobiliary Surg Nutr. 2019;8(6):651–654.
- Joo I, Lee JM. Recent advances in the imaging diagnosis of hepatocellular carcinoma: value of gadoxetic acid-enhanced MRI. Liver Cancer. 2016;5(1):67–87.
- Kudo M, Matsui O, Izumi N. et al. JSH consensus-based clinical practice guidelines for the management of hepatocellular carcinoma: 2014 update by the liver cancer study group of Japan. Liver Cancer. 2014;3(3–4):458–468.
- Lee S, Kang TW, Song KD, et al. Effect of microvascular invasion risk on early recurrence of hepatocellular carcinoma after surgery and radiofrequency ablation. Annals Surgery. 2019. Doi: 10.1097/SLA.0000000000003268
- Lee S, Kim SH, Lee JE, et al. Preoperative gadoxetic acid-enhanced MRI for predicting microvascular invasion in patients with single hepatocellular carcinoma. J Hepatol. 2017;67(3):526–534.
- Ariizumi S, Kitagawa K, Kotera Y, et al. A non-smooth tumor margin in the hepatobiliary phase of gadoxetic acid disodium (Gd-EOB-DTPA)-enhanced magnetic resonance imaging predicts microscopic portal vein invasion, intrahepatic metastasis, and early recurrence after hepatectomy in patients with hepatocellular carcinoma. J Hepatobiliary Pancreat Sci. 2011;18(4):575–585.
- Ahn SY, Lee JM, Joo I, et al. Prediction of microvascular invasion of hepatocellular carcinoma using gadoxetic acid-enhanced MR and (18)F-FDG PET/CT. Abdom Imaging. 2015;40(4):843–851.
- Lee LH, Hwang JI, Cheng YC, et al. Comparable outcomes of ultrasound versus computed tomography in the guidance of radiofrequency ablation for hepatocellular carcinoma. PLoS One. 2017;12(1):e169655.
- Song KD, Lee MW, Rhim H, et al. Percutaneous US/MRI fusion-guided radiofrequency ablation for recurrent subcentimeter hepatocellular carcinoma: technical feasibility and therapeutic outcomes. Radiology. 2018;288(3):878–886.
- Sanga K, Numata K, Nihonmatsu H, et al. Use of intra-procedural fusion imaging combining contrast-enhanced ultrasound using a perflubutane-based contrast agent and auto sweep three-dimensional ultrasound for guiding radiofrequency ablation and evaluating its efficacy in patients with hepatocellular carcinoma. Int J Hyperthermia. 2020;37(1):202–211.
- Toshikuni N, Takuma Y, Tomokuni J, et al. Planning sonography using real-time virtual sonography and contrast-enhanced sonography for radiofrequency ablation of inconspicuous hepatocellular carcinoma nodules. Hepatogastroenterol. 2015;62(139):661–666.
- Kunishi Y, Numata K, Morimoto M, et al. Efficacy of fusion imaging combining sonography and hepatobiliary phase MRI with Gd-EOB-DTPA to detect small hepatocellular carcinoma. Am J Roentgenol. 2012;198(1):106–114.
- Nishigori S, Numata K, Irie K, et al. Fusion imaging with contrast-enhanced ultrasonography for evaluating the early therapeutic efficacy of radiofrequency ablation for small hypervascular hepatocellular carcinomas with iso-echoic or unclear margins on conventional ultrasonography. J Med Ultrason. 2018;45(3):405–415.
- International Working Party. Terminology of nodular hepatocellular lesions. Hepatology. 1995;22(3):983–993.
- International-Consensus-Group-for-Hepatocellular-Neoplasia. Pathologic diagnosis of early hepatocellular carcinoma: a report of the international consensus group for hepatocellular neoplasia. Hepatology. 2009;49(2):658–664.
- Wang F, Numata K, Nakano M, et al. Diagnostic value of imaging methods in the histological four grading of hepatocellular carcinoma. Diagnostics. 2020;10(5):321.
- Lee JY, Minami Y, Choi BI, et al. The AFSUMB consensus statements and recommendations for the clinical practice of contrast-enhanced ultrasound using Sonazoid. Ultrasonography. 2020;39(3):191–220.
- Facciorusso A, Del PV, Antonino M, et al. Post-recurrence survival in hepatocellular carcinoma after percutaneous radiofrequency ablation. Dig Liver Dis. 2014;46(11):1014–1019.
- Kim SH, Lim HK, Choi D, et al. Percutaneous radiofrequency ablation of hepatocellular carcinoma: effect of histologic grade on therapeutic results. AJR Am J Roentgenol. 2006;186(5_supplement):S327–S333.
- Kondo F. Histological features of early hepatocellular carcinomas and their developmental process: for daily practical clinical application: hepatocellular carcinoma. Hepatol Int. 2009;3(1):283–293.
- Cho ES, Choi JY. MRI features of hepatocellular carcinoma related to biologic behavior. Korean J Radiol. 2015;16(3):449–464.
- Fukuda S, Itamoto T, Nakahara H, et al. Clinicopathologic features and prognostic factors of resected solitary small-sized hepatocellular carcinoma. Hepatogastroenterology. 2005;52(64):1163–1167.
- Lam VW, Ng KK, Chok KS, et al. Risk factors and prognostic factors of local recurrence after radiofrequency ablation of hepatocellular carcinoma. J Am Coll Surg. 2008;207(1):20–29.
- Ng KK, Poon RT, Lo CM, et al. Analysis of recurrence pattern and its influence on survival outcome after radiofrequency ablation of hepatocellular carcinoma. J Gastrointest Surg. 2008;12(1):183–191.
- Sakuraoka Y, Kubota K, Imura J, et al. Microsatellite analysis of recurrent lesions confirms merit of anatomical liver resection for hepatocellular carcinoma. Anticancer Res. 2019;39(8):4315–4324.
- Minami Y, Kudo M. Ultrasound fusion imaging technologies for guidance in ablation therapy for liver cancer. J Med Ultrasonics. 2020;47(2):257–263.
- Huang HW. Influence of blood vessel on the thermal lesion formation during radiofrequency ablation for liver tumors. Med Phys. 2013;40(7):73303.
- Nuta J, Shingaki N, Ida Y, et al. Irregular defects in hepatocellular carcinomas during the kupffer phase of contrast-enhanced ultrasonography with perfluorobutane microbubbles: pathological features and metastatic recurrence after surgical resection. Ultrasound Med Biol. 2017;43(9):1829–1836.
- Nuta J, Tamai H, Mori Y, et al. Kupffer imaging by contrast-enhanced sonography with perfluorobutane microbubbles is associated with outcomes after radiofrequency ablation of hepatocellular carcinoma. J Ultrasound Med. 2016;35(2):359–371.
- Xu E, Long Y, Li K, et al. Comparison of CT/MRI-CEUS and US-CEUS fusion imaging techniques in the assessment of the thermal ablation of liver tumors. Int J Hyperthermia. 2018;35(1):159–167.
- Roccarina D, Garcovich M, Ainora ME, et al. Usefulness of contrast enhanced ultrasound in monitoring therapeutic response after hepatocellular carcinoma treatment. World J Hepatol. 2015;7(14):1866–1874.