Abstract
Purpose
While systemic tumor-stimulating effects can occur following ablation of normal liver linked to the IL-6/HGF/VEGF cytokinetic pathway, the potential for tumor cells themselves to produce these unwanted effects is currently unknown. Here, we study whether partially treated tumors induce increased tumor growth post-radiofrequency thermal ablation (RFA).
Methods
Tumor growth was measured in three immunocompetent, syngeneic tumor models following partial RFA of the target tumor (in subcutaneous CT26 and MC38 mouse colorectal adenocarcinoma, N = 14 each); and in a distant untreated tumor following partial RFA of target subcutaneous R3230 rat breast adenocarcinoma (N = 12). Tumor cell proliferation (ki-67) and microvascular density (CD34) was assessed. In R3230 tumors, in vivo mechanism of action was assessed following partial RFA by measuring IL-6, HGF, and VEGF expression (ELISA) and c-Met protein (Western blot). Finally, RFA was performed in R3230 tumors with adjuvant c-Met kinase inhibitor or VEGF receptor inhibitor (at 3 days post-RFA, N = 3/arm, total N = 12).
Results
RFA stimulated tumor growth in vivo in residual, incompletely treated surrounding CT26 and MC38 tumor at 3–6 days (p < 0.01). In R3230, RFA increased tumor growth in distant tumor 7 days post treatment compared to controls (p < 0.001). For all models, Ki-67 and CD34 were elevated (p < 0.01, all comparisons). IL-6, HGF, and VEGF were also upregulated post incomplete tumor RFA (p < 0.01). These markers were suppressed to baseline levels with adjuvant c-MET kinase or VEGF receptor inhibition.
Conclusion
Incomplete RFA of a target tumor can sufficiently stimulate residual tumor cells to induce accelerated growth of distant tumors via the IL-6/c-Met/HGF pathway and VEGF production.
Introduction
Thermal ablation using radiofrequency (i.e., RFA) and other methods for percutaneously destroying focal tumors are now commonly used to treat a wide range of focal primary and metastatic tumors in the liver, particularly of colorectal and breast origin [Citation1–3]. Although it has been asserted that thermal ablation is a local therapy, recent research suggests that it can occasionally induce systemic effects including potentially unwanted ‘off-target’ tumorigenesis in local and distant tumor [Citation4–8]. Indeed, several experimental and clinical studies of thermal ablation for focal liver tumors have clearly documented this phenomenon when the ablation has included non-lethal cellular injury of both tumor and normal tissue from non-lethal heating (i.e., incomplete treatment). Recently, additional studies have demonstrated that ablation of normal liver and kidney tissue (i.e., a simulation of complete ablation including the periablational margin) can stimulate growth of distant R3230 and MATIII mammary tumors [Citation4,Citation5]. Yet, it remains uncertain to what extent partial ablation of tumor alone stimulates local or distant tumor growth.
Several mechanisms have been linked to off-target stimulation of local and distant tumor growth following combined ablation of tumor and normal tissue, or normal tissue alone [Citation4]. Increased inflammatory cytokines, pro-angiogenic factors, hypoxia inducible factor, heat shock proteins, and several HIF-1a, VEGF, and heat shock proteins have all been linked to increased tumor growth following incomplete thermal ablation [Citation6,Citation8]. More recently, several studies have linked several key components of a mechanistic pathway – namely, IL-6, HGF, c-Met, STAT3, and VEGF produced in response to ablation of normal liver tissue, to off-target stimulation of angiogenesis and distant tumor growth [Citation5,Citation9–11]. However, the role of this pathway following partial incomplete ablation of tumor tissue has not been well characterized.
To mitigate this unwanted side-effect of an otherwise robust interventional oncologic therapy, the pharmacologic targeting of key mediators driving post-ablation tumor stimulation offers a potentially effective way to suppress pro-tumorigenic effects of thermal tumor ablation [Citation4,Citation5]. Yet, variable results have been reported based upon the mediator and drug selected [Citation11]. Accordingly, identifying precise mechanistic pathways that are both increased following thermal ablation and shared between both normal tissue and tumor cells will be critical for developing clinically relevant strategies to suppress post-ablation tumorigenic effects. Hence, the purposes of this study were: 1) to determine the in vivo effect of partial (i.e., incomplete) thermal ablation on local and distant tumor growth in the absence of underlying host organ effects, 2) to determine the role of the IL-6/HGF/c-Met pathway in distant tumor stimulation following partial tumor ablation, and 3) to determine whether targeting key mediators including c-Met receptor and VEGF can suppress in vivo tumor-driven distant tumor stimulation.
Materials and methods
Experimental overview
The study was performed in two phases. First, in vivo tumor growth studies were performed in three separate syngeneic species-specific tumors in immunocompetent animals. Tumor growth was measured following partial thermal ablation of subcutaneous CT26 and MC38 colorectal adenocarcinoma tumors (n = 7 per group, n = 14 total for each model). Next, growth of a second separate, distant subcutaneous R3230 tumor was assessed following partial thermal ablation of one of two implanted R3230 breast adenocarcinoma tumors (N = 12). For all three models, outcome measures included measurement of tumor growth, and immunohistochemical pathologic assessment for tumor proliferation (Ki-67) and microvascular density (CD34).
Next, the mechanism of distant tumor stimulation was assessed following partial thermal ablation of one of two implanted R3230 tumors based upon the kinetics of mechanisms previously demonstrated to be active following thermal ablation of normal liver [Citation11,Citation12]. This included measuring IL-6 at 6 h, and HGF and VEGF at 72 h expression in residual periablational tumor compared to sham controls with ELISA (n = 3 per group, n = 12 total) based upon known peak expression of these cytokines in prior animal studies [Citation4,Citation12]. c-Met protein in the residual periablational tumor was quantified with Western blot at 7 days (n = 6). Finally, thermal ablation was performed in one of two implanted R3230 tumors with either adjuvant c-Met kinase inhibitor (PHA-665752) or VEGF receptor inhibitor (SU5416) administered 3 days after ablation (n = 3 each arm, total = 12).
Animal models
All portions of our study were approved by the institutional animal care and use committee. All experiments were performed by individuals with experience in performing tumor implantation, RF thermal ablation, and surgery in these models. For all procedures, anesthesia was induced with intraperitoneal injection of a ketamine (50 mg/kg) and xylazine (5 mg/kg). Animals were sacrificed by carbon dioxide overdose (SMARTBOX CO2; EZ Systems). Initially, CT26 colon carcinoma (American Type Culture Collection (ATCC)) and MC38 colon carcinoma (Varol laboratory, Tel Aviv Medical Center) were implanted subcutaneously by slowly injecting 2 × 106 cells to 8 weeks male BALB/c mice and C57B6 mice (Harlan Laboratories, Jerusalem, Israel), respectively. Experiments to assess the effects on distant tumor were performed in a larger, well-characterized R3230 mammary adenocarcinoma model [Citation13]. Cells were implanted into of 14–16 weeks female Fisher 344 (Charles River, Wilmington, MA) rats weighing 150 ± 20 g by slowly injecting 0.3–0.4 ml of tumor suspension into the mammary fat pad of each animal as previously described [Citation14].
Thermal ablation with RF energy
Conventional monopolar RFA was applied by using a 500-kHz RFA generator (model 3E; Cosman Medical, Burlington, MA) [Citation14]. Briefly, the 1-cm tip of a 21-gauge electrically insulated electrode (SMK electrode; Cosman Medical) was placed in the center of the tumor. RFA was applied for 5 min with energy titrated to maintain 70 ± 2 °C as this provides reproducible 5-6 mm coagulation diameters which represents approximately half the diameter and one quarter of the volume of the target tumor at the time of the ablation [Citation14,Citation15]. Sham procedures were performed by inserting a needle into the tumor without activation of RFA for the specified ablation time.
Drug administration
PHA-665752, a c-Met inhibitor, was prepared in 0.9% NaCl to achieve a dose of 0.83 gm/kg with 1 ml administered IP 3 days post-RFA [Citation4]. SU5416, a VEGF receptor inhibitor, was mixed in dimethyl sulfoxide to achieve a dose of 8 mg/kg in 200 μl, administered via IP injection 3 days after RFA, based upon previous studies demonstrating both maximum peak serum HGF at this time and efficacy in blocking this cytokine and subsequent growth following RF ablation of the liver using this compound [Citation4].
Tumor measurements
Tumors were measured in both longitudinal and transverse diameters using mechanical calipers, and an average diameter was calculated. Tumors reaching 6–7 mm were measured for approximately 5 days to determine a temporal pretreatment growth rate. Once tumors reached target mean diameters of 10–11 mm they were randomly allocated to specified treatment arms. Mean initial tumor size was similar for all comparative treatment groups. After RFA or sham treatment, measurements were obtained for an additional 3, 6, and 7 days for the MC38, CT26, and R3230 models, respectively (based upon tumor growth rates and ethical considerations of maximum tolerated tumor burden).
Tissue harvesting and preparation
Animals were sacrificed at specified times. The primary site of tumor ablation was harvested and sliced perpendicularly to the direction of electrode insertion [Citation14,Citation16]. Distant tumors were harvested in a similar manner. Samples were fixed in 10% formalin, embedded in paraffin, and sliced to 5 µm thickness prior to staining with hematoxylin and eosin.
Immunohistochemical staining
Immunohistochemical staining was performed to quantify cell proliferation (Ki-67, rat: Ab16667, Abcam; mouse: GTX20833, GeneTex) and microvascular density (CD34, an endothelial cell marker, rat: Ab8158, Abcam; mouse: MCA1825 Bio-Rad) [Citation16]. Specimen slides were imaged and analyzed using a microscope (Micromaster I; Fisher Scientific) and software (Micron Imaging; Westover Scientific, Mill Creek, Wash). Five random high-power fields (HPF) were analyzed for a minimum of three specimens for each parameter and scored in a blinded fashion to remove observer bias. For Ki-67, the percentage of positive cells (a ratio of stained and unstained cells) was calculated for each field and averaged for each specimen. For c-Met (SC-162), intratumoral periablational rim thickness and percentage cell positivity were recorded using methods previously described for other proteins upregulated in the periablational rim [Citation16].
Quantification of c-Met, VEGF, and HGF
c-Met quantification was performed using Western blot analysis. Microdissection of excised tumors was performed to obtain the area of interest. Given the amounts of tissue obtained, a minimum of three primary or distant tumors tissues were pooled and homogenized together using cell lysis buffer. Briefly, protein was quantified using a bicinchoninic acid method (Sigma-Aldrich, St. Louis, Mo), with 60 µg total protein loaded on 10% sodium dodecyl sulfate–polyacrylamide gels and blotted onto nitrocellulose membranes. Nonspecific binding was blocked with 5% (wt/vol) skim milk powder in PBS with Tween-20 (Cell Signaling Technology, Danvers, MA) for 1 h followed by incubation with c-Met 1:100 (45 kDa [SC-162; Santa Cruz Biotechnology, Dallas, TX]) antibody overnight at 4 °C. The membrane was then incubated with appropriate anti-rabbit secondary antibodies followed by radiographic detection. Band intensities were quantified with densitometry using NIH ImageJ 1.3. Standardization of protein amount was performed using β-actin. Positive controls were also tested for all assays using A431 cells with known c-Met positivity. Serum and tissue levels of HGF (rat/MHG00, R&D Systems) and VEGF (rat/RRV00 Quantikine kit, R&D Systems) were determined using an ELISA kit according to manufacturer’s instructions. The homogenates of undiluted serum were centrifuged (14,000 rpm; 20 min; 4 °C), and total protein concentration was determined using bicinchoninic acid. HGF and VEGF values were normalized to protein concentration. All samples and standards were measured in duplicate [Citation17,Citation18].
Statistical analysis
SPSS 13.0 software (SPSS, Chicago, IL) was used for statistical analysis. All data are reported as a mean ± standard deviation. Mean tumor sizes at day 0 and time of sacrifice and immunohistochemical quantification were compared with analysis of variance (ANOVA), with testing including a post-treatment interaction term. Additional post-hoc analysis was performed with a two-sample, two-tailed Student T test if, and only if, the results achieved statistical significance (p < 0.05).
Results
Partial thermal ablation of local tumor accelerates local and distant tumor growth
Acceleration of both MC38 and CT26 colorectal tumor growth was noted following partial thermal ablation of the tumor (p < 0.01; , ). Partial ablation also increased residual tumor cell proliferation compared to sham in both cancer models (p < 0.001; ). Furthermore, thermal ablation significantly increased microvascular density in the residual tumor compared to sham procedure (p < 0.001; ).
Figure 1. RF ablation (RFA) of local tumor accelerates distant tumor growth. (A) Curves for MC38 tumors demonstrate an identical rate of growth for all groups before treatment After partial treatment with RFA (day 1), the tumor growth rate is significantly greater such that tumor diameter is significantly greater (p < 0.01) than that with sham treatment. (B) Curves for CT26 tumors demonstrate an identical rate of growth for all groups before treatment. After partial treatment with RFA (day 3), the tumor growth rate is significantly greater such that tumor diameter is significantly greater (p < 0.01) than that with sham treatment. (C,D) Immunohistochemical (IHC) staining for ki-67 demonstrating cell proliferation. RFA increased cell proliferation compared to sham treatment for MC38(C), and CT26 (D). (E,F) IHC staining for CD34 demonstrating microvascular density. RFA increased cell microvascular density compared to sham treatment for MC38 (E), and CT26 (F). G. Curves for R3230 tumors demonstrate identical growth rates for all groups before treatment. After tumor RFA (day 0), growth distant tumors is significantly greater such that tumor diameter is significantly greater than that with sham treatment.
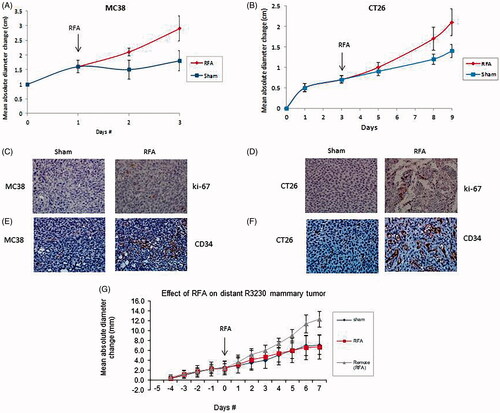
Table 1. Tumor growth and proliferative index for rodent tumors post RFA.
Over 7 days of post-ablation follow-up, coagulation zones in the primary tumor measured 6.5 + 0.4 mm in diameter, which is statistically identical to that observed immediately post-ablation [Citation14]. Nevertheless, distant R3230 mammary tumor growth rates accelerated to double compared to sham procedure after partial ablation of primary subcutaneous tumor (, ). Partial ablation of local tumor also significantly increased cell proliferation in both residual tumor cells in the target tumor as well as in the distant tumor compared to sham model (p < 0.001, both comparisons). Further, thermal ablation significantly increases microvascular density in both the residual untreated portions of the target tumor and in remote tumor compared to sham (p < 0.001, both comparisons).
Thermal tumor ablation increases IL6, c-Met, HGF and VEGF levels in peri-ablational and distant tumor
Compared with sham treatment, partial ablation increased IL-6 levels in the ablated (p = 0.004) and distant R3230 tumor (p < 0.001) at 6 h post RFA (). c-Met positive cells were observed 7 days post ablation particularly congregating in the untreated tumor most adjacent to the initial ablation zone (35.4 ± 0.1% positive cells/HPF) (). Increased levels of HGF were observed 72 h after ablation compared to sham treatment in treated (p < 0.001) and distant tumor (p < 0.01). VEGF levels were also increased in ablated tumor compared to sham procedure (p < 0.001) and in distant tumor (p = 0.01) 72 h post-treatment.
Figure 2. PHA reduces post-RFA c-Met expression in the periablational rim. (A) Immunohistochemical staining for c-Met receptor demonstrating c-Met expression in RFA periablational rim (arrowheads) vs. RFA with PHA 7 days post treatment. PHA addition to RFA reduces significantly c-Met expression (to minimal expression – arrowheads) compared to RFA alone. (B) Bar chart quantifying this infiltration of c-Met receptor + cells into the tumoral periablational rim 7 days post RFA. There is a significant reduction of c-Met positive cells in tumor tissue post RFA treated with PHA compared to RFA alone. (C,D) Western blot assays demonstrate increased c-Met receptor protein in the periablational tissue surrounding the ablation zone from pooled samples compared with sham treatment (seen as dense bands after gel electrophoresis at 50-kDa level, where the c-Met receptor a subunit is expected, after b-actin standardization). c-Met receptor protein levels are also increased in the distant untreated tumor after RFA compared with sham treatment. Addition of PHA reduces c-Met receptor protein levels in both local and distant tumor. (A431: Positive control; ST: Sham tumor; RFT: RFA tumor; RPT: RFA + PHA tumor; SRT: Sham remote tumor; RFRT: RFA remote tumor; RPRT: RFA + PHA remote tumor).
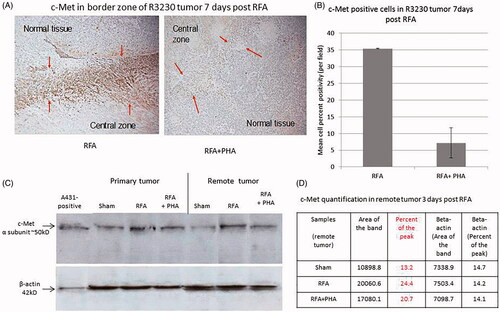
Table 2. Serologic cytokine expression for rodent tumors post RFA.
PHA reduces c-Met expression post-thermal ablation
c-Met positive cells were observed 7 days following partial RFA particularly congregating in the untreated tumor most adjacent to the initial ablation (35.4 ± 0.1% positive cells/HPF). Addition of PHA 3 days after RFA significantly reduced c-Met positive stained cells by 95% (7.2 ± 4.5% cells/HPF, p = 0.01) (). Western blot further confirmed that adding PHA 3 days post-RFA also reduced c-Met expression to sham level (9.9% peak densitometry) both in the residual viable portions of the partially ablated target tumor (10.6% vs RFA 21.1% of peak) as well as in distant tumor, (20.7% vs RFA 24.4% vs sham 13.2% of peak) ().
PHA suppresses distant tumor growth after local tumor ablation
Addition of PHA 3 days following incomplete RFA decreased distant tumor growth so that the tumor size at 7 days was equivalent to the sham group (). Similarly, a significant decrease in tumor cell proliferation to baseline levels was observed at 7 days between distant tumor receiving PHA 3 days following RFA compared to RFA alone (p < 0.001) (). Microvascular density was also reduced to baseline levels in both the residual viable target tumor and in distant tumor tissue. Control animals treated with PHA alone had similar tumor sizes and proliferative indices to the sham group (p = 0.25) (, ).
Figure 3. Inhibitors suppress distant tumor growth after local tumor RFA. (A) Graph of tumor growth of local and distant tumor with or without PHA treatment demonstrates the effect of tumor RFA on distant tumor growth in R3230 adenocarcinoma cell line in the presence of a c-Met kinase inhibitor. Graph shows that adjuvant PHA given 3 days after RFA decreases distant R3230 tumor growth rate back to baseline compared with RFA alone. PHA alone treatment arm has same growth rate as sham arm indicating that c-Met inhibitor does not have active effect on tumor growth by itself. B. Effect of tumor RFA on distant tumor growth in R3230 adenocarcinoma cell line with suppression of VEGF receptor inhibitor. Similar to PHA, anti-VEGF blocks R3230 tumor growth back to baseline compared to sham.
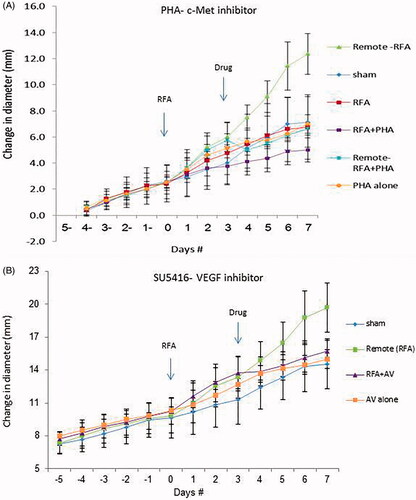
Figure 4. PHA reduces cell proliferation following RFA. Immunohistochemical staining for ki-67 demonstrating cell proliferation. Tumor RFA increases nuclear staining, with PHA treatment reducing cell proliferation to sham level 7 days post RFA.
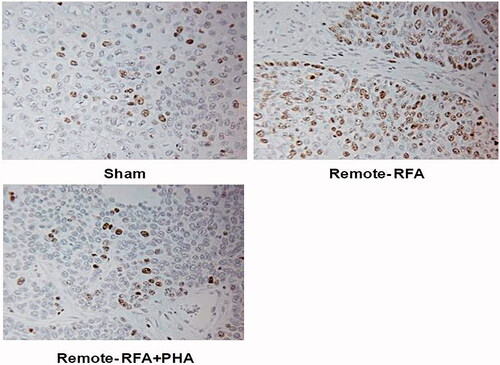
Figure 5. PHA reduces microvascular density levels following RFA. Microvascular density for RFA periablational rim vs RFA with PHA 7 days post treatment. Immunohistochemical staining for CD34 demonstrating increases in microvascular density post-RFA in local periablational rim and distant tumor. Addition of PHA to RFA reduced microvascular density levels to that similar to sham. ***Ablated zone adjacent to the periablational zone.
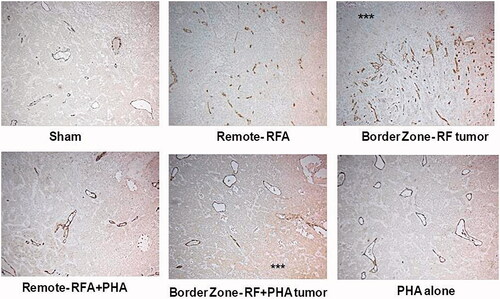
PHA effects IL6, HGF and VEGF levels in peri-ablational and distant tumor following thermal ablation
Addition of PHA at the time of RFA reduced IL-6 levels in both the treated (p = 0.02) and distant tumor (p = 0.008). PHA reduced HGF to control levels in ablated (p < 0.001) and distant tumor (p = 0.002). Addition of PHA to RF inhibited increases of VEGF in both treated and distant tumor (p < 0.01). In animals treated with PHA alone, factor levels were similar to the sham group ().
Anti-VEGF suppresses distant tumor growth after thermal ablation
Adding VEGF inhibitor (SU5416) 3 days post incomplete RFA also reduced tumor growth rate resulting in similar tumor size as sham treatment 7 days post-RFA (, ). Addition of VEGF inhibitor 3 days post-RFA also reduced distant tumor cell proliferation (p < 0.001) and microvascular density (p = 0.04), equivalent to sham and inhibitor alone (p = 0.51).
Discussion
Off-target tumor stimulatory effects following thermal ablation for intrahepatic tumor have been described in both experimental and clinical studies [Citation4,Citation5,Citation10]. However, by definition all clinical studies and many of prior experimental models have studied partial ablation of both tumor and hepatocytes which has precluded ascertaining the extent of the role played by incomplete tumor ablation on any potential systemic effects of treatment. Here, our study specifically demonstrates that RFA of tumor cells alone (without any additional ablation of normal non-tumor tissue) stimulates both growth of local untreated tumor and of distant untreated tumor separate from native hepatic cell populations. This is clinically relevant given studies that have demonstrated that viable partly injured tumor cells can be present in 13–27%% of cases, despite initial evidence of technical success and where complete ablation was anticipated [Citation18,Citation19]. Furthermore, we provide additional evidence that these phenomena can be seen in different tumor types, including colorectal and breast cancers – two types of metastatic hepatic tumors treated with RF and microwave ablation [Citation1,Citation2].
Our study also links tumor-driven post-ablation stimulation of distant tumor growth in a breast adenocarcinoma model to increased activation of the IL-6/HGF/c-Met pathway which is sufficiently activated locally within the intratumoral periablational rim to produce systemic downstream distant tumor angiogenesis and VEGF production [Citation4]. These growth factors also have well-established roles promoting tumor growth, metastatic invasion, and aggressive tumor biology [Citation20–26]. Regardless, our results provide additional evidence of the primary contribution of this particular mechanistic pathway in off-target systemic stimulation of distant extra-organ tumor. This builds upon several experimental studies that have already identified this mechanism as a significant driver of distant tumor growth following thermal ablation of normal liver or kidney tissue [Citation4]. Moreover, our results suggest a common protean pathway for both normal and malignant tissues with this pro-tumorigenic pathway retained even in relatively undifferentiated cell populations such as tumors [Citation4,Citation5,Citation11,Citation31,Citation32]. Regardless, our results clearly demonstrate that some tumors when only partially treated by RF are endowed with and retain enough basic machinery to produce sufficient quantities of the same factors as normal liver to induce tumorigenesis [Citation4,Citation5,Citation11,Citation31,Citation32].
The findings in this study also support the role of adjuvant inhibition of either c-Met (via PHA) or VEGFR (via SU5416) – two key targets of the IL-6/HGF/Stat-3/VEGF pathway – in suppressing these stimulatory effects from partially injured tumor cells following thermal ablation. Both c-Met and VEGFR blockade were equally effective in blocking off-target effects of ablation. Interestingly, studies using these agents following thermal ablation in normal liver demonstrated better efficacy with PHA compared to incomplete suppression of distant tumor stimulation with SU5416 [Citation4]. This suggests that some differences may exist between adjuvant drug efficacy based upon which types of tissue are driving post-ablation reactions, and should be factored into adjuvant drug strategies to suppress off-target effects.
The results of our study emphasize the need to determine which types of tumor will activate these and possibly other tumorigenic factors and increase tumor progression post partial ablation. Indeed, it must be noted that the inflammatory and wound-healing response that occurs within the periablational rim is complex, and associated with a host of secondary reactions [Citation12]. Indeed, we readily note that several other mediators and potential pathways have been linked to off-target tumor stimulation following thermal ablation of hepatic tumors, including hypoxia (HIF1a) [Citation8], activation of the PI3/Akt pathway, the erk pathway [Citation27], nerve inducible factor [Citation6], and heat stress responses (i.e., heat shock proteins) [Citation28]. While most of these pathways have been studied in models of partial tumor cell injury, our results combined with these studies suggest that different tumor populations can potentially express and upregulate a range of pro-tumorigenic pathways. Moreover, while it is probable that some tumor types will not display any post-ablation tumorigenic effects, it is further possible that some tumors may exhibit the activation of more than one pro-tumorigenic pathway, particularly given the heterogeneity of many commonly treated tumors that may be composed of multiple subtypes and a host of supporting cells comprising the microenvironment possessing various receptors or other oncogenic expressions. Accordingly, a future goal will be to determine the genetic profile of thermally stressed (i.e., partially ablated) human tumor cell types to determine genetic and protein upregulation with an ultimate goal of identifying potential biomarkers to determine which tumor ablation require adjuvant treatment to minimize tumorigenesis [Citation29].
In this study, we further noted that the secretion of pro-tumorigenic cytokines and the resultant tumorigenesis occur relatively rapidly (i.e., within hours to days) after treatment. Thus, the current results suggest that it may be warranted to attempt to identify and eliminate any visible residual tumor as soon as possible, ideally in the initial ablation session. This would support the contention of those promoting multiple methods of intra-procedural assessment including contrast enhanced ultrasound, cone bean CT, or MR thermal monitoring, and the timing of the first post-ablation imaging follow-up [Citation30]. Furthermore, our study raises concern that palliative treatment alone without additional adjuvant therapy may not be prudent, particularly in cases were residual tumors – visible or otherwise – is highly suspected. Moreover, any increase in tumorigenesis from residual tumor post ablation would call into question the potential appropriateness of using ablation as a palliative treatment or stimulator for immunotherapy.
We acknowledge that this study has several limitations. Specifically it is unknown which if any human tumor cells lines can produce pro-tumorigenic effects when partially ablated. Future study must also include elucidation of tumorigenesis in other relevant organs particularly for kidney tumors where increased IL-6 and/or HGF have been detected post-RFA [Citation4]. Likewise, further study beyond subcutaneous tumors particularly by expanding to the study of orthotopic models may shed additional light on this phenomenon by including the tumor microenvironment tumorigenenic effect post-RFA. Similarly, this study demonstrates differences in tumor growth curves following RF ablation between the colorectal and breast adenocarcinoma models, which likely suggests differences in tumor response to ablation-induced tumorigenic effects, underscoring the need for further expanded study across multiple tumor types commonly ablated in clinical practice [Citation31]. Even though our data are clearly relevant to all methods of thermal ablation including the ever more used microwave ablation, assessing the extent of variability in tumorigenesis when using other ablation modalities (including microwave and IRE) that have been shown to have tumorigenic effects is warranted [Citation31,Citation32]. We further acknowledge that only a limited number of standardized RF and thermal doses were studied, and thus ultimately more complete modeling of these responses may be required.
In conclusion, incomplete RFA of a tumor can stimulate tumor growth of local residual and distant tumors, with this process stimulated partially by the cellular response of the periablational rim including the IL-6/c-Met/HGF pathway and VEGF. By blocking c-Met or VEGF with inhibitors after RFA this phenomena is suppressed offering a potential strategy to deal with this issue in clinical practice.
Disclosure statement
S. Nahum Goldberg – Unrelated consulting and sponsored research support from Angiodynamics and Cosman Company. Muneeb Ahmed – Unrelated consulting support from Canon Medical, Inc, Agile Devices, Inc, and RevOps Health, Inc; Scientific Advisory Board for Boston Scientific. All other authors have no conflicting interests to report.
Additional information
Funding
References
- Solbiati L, Ahmed M, Cova L, et al. Small liver colorectal metastases treated with percutaneous radiofrequency ablation: local response rate and long-term survival with up to 10-year follow-up. Radiology. 2012;265:958–968.
- Meloni MF, Andreano A, Laeseke PF, et al. Breast cancer liver metastases: US-guided percutaneous radiofrequency ablation-intermediate and long-term survival rates . Radiology. 2009;253:861–869.
- EASL-EORTC. EASL-EORTC clinical practice guidelines: management of hepatocellular carcinoma. J Hepatol. 2012;56:908–943.
- Ahmed M, Kumar G, Moussa M, et al. Hepatic radiofrequency ablation-induced stimulation of distant tumor growth is suppressed by c-Met inhibition. Radiology. 2016;279:103–117.
- Rozenblum N, Zeira E, Scaiewicz V, et al. Oncogenesis: an "off-target" effect of radiofrequency ablation. Radiology. 2015;276:426–432.
- Thompson SM, Jondal DE, Butters KA, et al. Heat stress and thermal ablation induce local expression of nerve growth factor inducible (VGF) in hepatocytes and hepatocellular carcinoma: pre-clinical and clinical studies. Gene Expr. 2018;19:37–47.
- Nijkamp MW, Borren A, Govaert KM, et al. Radiofrequency ablation of colorectal liver metastases induces an inflammatory response in distant hepatic metastases but not in local accelerated outgrowth. J Surg Oncol. 2010;101:551–556.
- Nijkamp MW, van der Bilt JD, de Bruijn MT, et al. Accelerated perinecrotic outgrowth of colorectal liver metastases following radiofrequency ablation is a hypoxia-driven phenomenon. Ann Surg. 2009;249:814–823.
- Erinjeri JP, Thomas CT, Samoilia A, et al. Image-guided thermal ablation of tumors increases the plasma level of interleukin-6 and interleukin-10. J Vasc Interv Radiol. 2013;24:1105–1112.
- Hinz S, Tepel J, Roder C, et al. Profile of serum factors and disseminated tumor cells before and after radiofrequency ablation compared to resection of colorectal liver metastases – a pilot study. Anticancer Res. 2015;35:2961–2967.
- Ahmed M, Kumar G, Navarro G, et al. Systemic siRNA nanoparticle-based drugs combined with radiofrequency ablation for cancer therapy. Plos One. 2015;10:e0128910.
- Rozenblum N, Zeira E, Bulvik B, et al. Radiofrequency ablation: inflammatory changes in the periablative zone can induce global organ effects, including liver regeneration. Radiology. 2015;276:416–425.
- Yang W, Ahmed M, Tasawwar B, et al. Combination radiofrequency (RF) ablation and IV liposomal heat shock protein suppression: reduced tumor growth and increased animal endpoint survival in a small animal tumor model. J Control Release. 2012;160:239–244.
- Ahmed M, Monsky WE, Girnun G, et al. Radiofrequency thermal ablation sharply increases intratumoral liposomal doxorubicin accumulation and tumor coagulation. Cancer Res. 2003;63:6327–6333.
- Monsky WL, Kruskal JB, Lukyanov AN, et al. Radio-frequency ablation increases intratumoral liposomal doxorubicin accumulation in a rat breast tumor model. Radiology. 2002;224:823–829.
- Solazzo SA, Ahmed M, Schor-Bardach R, et al. Liposomal doxorubicin increases radiofrequency ablation-induced tumor destruction by increasing cellular oxidative and nitrative stress and accelerating apoptotic pathways. Radiology. 2010;255:62–74.
- Abarbanell AM, Wang Y, Herrmann JL, et al. Toll-like receptor 2 mediates mesenchymal stem cell-associated myocardial recovery and VEGF production following acute ischemia-reperfusion injury. Am J Physiol Heart Circ Physiol. 2010;298:H1529–H1536.
- Sotirchos VS, Petrovic LM, Gonen M, et al. Colorectal cancer liver metastases: biopsy of the ablation zone and margins can be used to predict oncologic outcome. Radiology. 2016;280:949–959.
- Snoeren N, Huiskens J, Rijken AM, et al. Viable tumor tissue adherent to needle applicators after local ablation: a risk factor for local tumor progression. Ann Surg Oncol. 2011;18:3702–3710.
- Ang CS, Sun MY, Huitzil-Melendez DF, et al. c-MET and HGF mRNA expression in hepatocellular carcinoma: correlation with clinicopathological features and survival. Anticancer Res. 2013;33:3241–3245.
- Dankbar B, Padro T, Leo R, et al. Vascular endothelial growth factor and interleukin-6 in paracrine tumor-stromal cell interactions in multiple myeloma. Blood. 2000;95:2630–2636.
- Gherardi E, Birchmeier W, Birchmeier C, et al. Targeting MET in cancer: rationale and progress. Nat Rev Cancer. 2012;12:89–103.
- Goyal L, Muzumdar MD, Zhu AX. Targeting the HGF/c-MET pathway in hepatocellular carcinoma. Clin Cancer Res. 2013;19:2310–2318.
- Middleton K, Jones J, Lwin Z, et al. Interleukin-6: an angiogenic target in solid tumours. Crit Rev Oncol Hematol. 2014;89:129–139.
- Mittal K, Ebos J, Rini B. Angiogenesis and the tumor microenvironment: vascular endothelial growth factor and beyond. Semin Oncol. 2014;41:235–251.
- Sun R, Jaruga B, Kulkarni S, et al. IL-6 modulates hepatocyte proliferation via induction of HGF/p21cip1: regulation by SOCS3. Biochem Biophys Res Commun. 2005;338:1943–1949.
- Thompson SM, Callstrom MR, Jondal DE, et al. Heat stress-induced PI3K/mTORC2-dependent AKT signaling is a central mediator of hepatocellular carcinoma survival to thermal ablation induced heat stress. PLoS One. 2016;11:e0162634.
- Yang W, Ahmed M, Tasawwar B, et al. Radiofrequency ablation combined with liposomal quercetin to increase tumour destruction by modulation of heat shock protein production in a small animal model. Int J Hyperthermia. 2011;27:527–538.
- Shady W, Petre EN, Vakiani E, et al. Kras mutation is a marker of worse oncologic outcomes after percutaneous radiofrequency ablation of colorectal liver metastases. Oncotarget. 2017;8:66117–66127.
- Bruenn K, Beyer L, Haimerl M, et al. Comparison of computed tomography (CT) and contrast-enhanced ultrasound (CEUS) for the quantitative evaluation of an ablation defect following radiofrequency ablation of malignant liver lesions. Clin Hemorheol Microcirc. 2017;67:445–451.
- Bulvik BE, Rozenblum N, Gourevich S, et al. Irreversible electroporation versus radiofrequency ablation: a comparison of local and systemic effects in a small-animal model. Radiology. 2016;280:413–424.
- Velez E, Goldberg SN, Kumar G, et al. Hepatic thermal ablation: effect of device and heating parameters on local tissue reactions and distant tumor growth. Radiology. 2016;281:782–792.