Abstract
Objective
To study the efficacy of LITT for BM patients experiencing in-field recurrence following SRS.
Methods
A literature search was conducted to identify studies investigating local control (LC) rate and overall survival (OS) of LITT for BMs with IFR following SRS.
Results
Analysis included 14 studies (470 patients with 542 lesions). The 6-month (LC-6) and 12-month (LC-12) local control rates were 78.5% (95% CI: 70.6–84.8%) and 69.0% (95% CI: 60.0–76.7%) separately. Pooled median OS was 17.15 months (95% CI: 13.27–24.8). The overall OS-6 and OS-12 rates were 76.0% (95% CI: 71.4–80.0%) and 63.4% (95% CI: 52.9–72.7%) separately. LITT provided more favorable local control efficacy in RN than BM recurrence (LC-6: 87.4% vs. 67.9%, p = 0.009; LC-12: 76.3% vs. 59.9%, p = 0.041).
Conclusions
LITT is an effective treatment for BM patients experiencing IFR following SRS. For different pathological entities, LITT showed more satisfactory local control efficacy on RN than BM recurrence.
Introduction
An estimated 20% of cancer patients will develop brain metastases (BMs), with the majority occurring in those with lung, breast cancers and melanoma [Citation1]. Stereotactic radiosurgery (SRS) alone or postoperatively to surgical cavity is now well-established as a standard first-line treatment for patients with a limited number of BMs [Citation2,Citation3]. However, recurrence within the previously irradiated field (also known as in-field recurrence, IFR) could be as high as 40% for radiation resistant cancers in radiological follow up [Citation4,Citation5], which may represent true tumor recurrence or radiation necrosis (RN) or a combination of both. Both BM recurrence and RN could be irreversibly progressive, leading to neurological deterioration and shortened lifespan [Citation6].
Currently no consensus on the treatment modality for IFR has been established. Surgical resection may be considered as the preferred salvage option [Citation7–9], but some patients are not amenable for resection due to deep-seated lesions or cannot tolerate the modality due to poor clinical performance status. Effective medical therapy in this setting remains elusive as significant heterogeneity exists with regard to pathology and drug related variables.
MRI-guided laser interstitial thermal therapy (LITT, also known as laser thermal ablation, SLA) is an emerging option which offers a minimally invasive approach for IFR following prior SRS [Citation10–34]. Although an increasing usage of LITT has been reported in the setting of IFR over the past decade [Citation10–34], they are limited by small sample sizes generally and lack of differentiation between BM recurrence and RN in some studies, which possibly resulted in wide variation on efficacy among different studies.
Here, we performed a systemic review and meta-analysis of the literature to determine the overall efficacy of LITT for IFR and to elucidate underlying causes of the variation.
Materials and methods
Data source
The literature search was conducted in PubMed, EMBASE and Cochrane Library (until October, 2020). Our search strategy included the terms ‘LITT’, ‘laser interstitial’, ‘thermal therapy’, ‘stereotactic laser ablation’ AND ‘brain’, ‘cerebral’, ‘intracranial’ AND ‘metastases’, ‘metastasis’, ‘metastatic’ and was restricted to human studies published in English. The following search strategy was used to search PubMed: (((LITT) OR (laser interstitial) OR (thermal therapy) OR (stereotactic laser ablation)) AND (brain)) AND ((tumor) OR (neoplasms) OR (metastasis) OR (radiation necrosis)) AND ((humans[Filter]) AND (english[Filter])). Articles were screened by title and abstract, and those passed screening were read in full. Additionally, we manually searched the reference lists of all accepted articles to screen for articles missed in the initial search.
Selection criteria and process
Studies that met the following criteria were chosen for the analysis: (i) clinical studies designed to evaluate the efficacy of LITT for IFR; (ii) using laser ablation, not cryotherapy or radiofrequency ablation; (iii) including intracranial BM recurrence or RN after SRS, not spinal lesions; (iv) more than five patients enrolled; (v) data for local control rate or overall survival (OS) was available. All the potentially relevant papers were reviewed independently by two investigators (CC and GYB) and disagreements were resolved by discussion and consensus. Details on study design, patient characteristics, local control rates and follow-up of survival were extracted by the two investigators. When data were solely provided in graphical form, figures were digitized to extract the numerical value using Engauge Digitizer version 11.1 (http://digitizer.sourceforge.net/).
Statistical analysis
The pooled median OS and corresponding 95% CI were pooled by R software 4.0.1 with ‘metamedian’ package [Citation35]. The pooled LC-6, LC-12, OS-6 and OS-12 rates were estimated with Comprehensive Meta-Analysis program version 3 (Biostat, Englewood, NJ, USA). For each meta-analysis, the Cochrane’s Q and I2 statistic were estimated to assess the heterogeneity of the included studies. For I2 > 50%, the assumption of homogeneity was deemed invalid, and the random-effects model was used. The fixed-effects model was chosen when I2≤50%. A two-tailed p value of <0.05 was deemed statistically significant.
Results
Identification of relevant studies
The flow diagram of the selection process for relevant studies was shown in . Our search yielded a total of 576 articles. 539 articles were excluded for not being related to the topic after reviewing the titles and abstracts, and the remaining 37 articles were reviewed further [Citation10,Citation11,Citation13–34,Citation36–48]. Twenty one studies were considered to be ineligible for inclusion for the following reasons: (i) five studies enrolled no more than five patients [Citation36–40]; (ii) nine studies did not describe the clinical endpoints of interest [Citation11,Citation14,Citation16,Citation18,Citation20,Citation26,Citation28,Citation33,Citation41]; (iii) one study enrolled patients who were included in another larger study [Citation13]; (iv) one study mixed brain metastases with primary brain tumor [Citation22]; (v) seven articles were reviews [Citation42–48]. Finally, 14 studies were included in the meta-analysis [Citation10,Citation15,Citation17,Citation19,Citation21,Citation23–25,Citation27,Citation29–32,Citation34]. It should be noted that patients enrolled in the study conducted by Hernandez et al. [Citation24]were included in the study conducted by Kaye et al. [Citation29], but the former provided local control rate which was not provided by the latter study, so the study conducted by Hernandez et al. was only included for local control analysis. Although enrolling both brain metastases and primary brain tumors, the study conducted by Smith et al. was included because the clinical endpoints of BMs could be calculated from raw data [Citation17].
Characteristics of included studies and patients
Clinical and treatment related characteristics for all included studies were summarized in and . The 14 studies were comprised of nine retrospective case series, two retrospective case-control studies, one phase I clinical trial, one phase II clinical trial and one prospective registry study. The two case-control studies compared LITT with surgical resection [Citation25] and bevacizumab [Citation34] on RN separately.
Table 1. Summary of characteristics and clinical features of included studies.
Table 2. Summary of local control efficacy, overall survival benefit and predictive factors of included studies.
A total of 470 patients with 542 lesions were included in the final analysis. Median age was 59.6 years (range 23–90). A female predominance (female: 281/429, 65.5% vs. male: 148/429, 34.5%) was noted. Median Karnofsky Performance Status (KPS) was 85 (range 50–100). The most common primary tumor type was lung cancer (127/299, 42.5%), following by breast cancer (65/299, 21.7%) and melanoma (44/299, 14.7%). Median pre-operative lesion volume was 4.6 cm3 (range 0.2–38.9).
Local control
Local control rate was available for analysis from a total of 342 lesions enrolled in ten studies. The 6-month local control rate (LC-6) ranged between 66.7% and 96.0% and the pooled effect was 78.5% (95% CI: 70.6–84.8%) as determined by the random-effects model (heterogeneity analysis: I2 = 50.350%, p = 0.034) (). The 12-month local control rate (LC-12) ranged between 56.0% and 84.7% and the pooled effect was 69.0% (95% CI: 60.0–76.7%) as determined by the random-effects model (heterogeneity analysis: I2 = 50.584%, p = 0.048) ().
Figure 2. Local control efficacy of LITT for BMs with IFR following SRS, including 6-month local control rate (a) and 12-month local control rate (b).
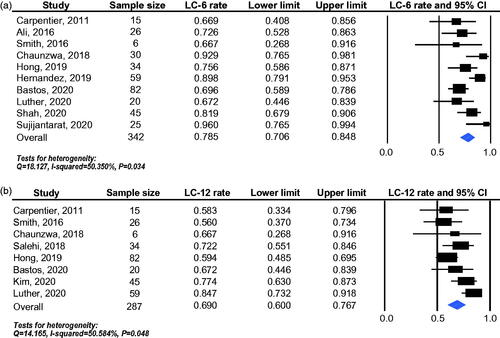
To explore the heterogeneity of the included studies, different pathological entities were further analyzed. In RN subgroup, 149 lesions enrolled in seven studies were available for LC-6 analysis and 75 lesions enrolled in four studies for LC-12 analysis. The pooled effects were 87.4% (95% CI: 75.6–93.9%) for LC-6 () and 76.3% (95% CI: 65.0–84.8%) for LC-12 () separately. In BM recurrence subgroup, 176 lesions enrolled in six studies were available for LC-6 analysis and 153 lesions enrolled in five studies for LC-12 analysis. The pooled effects were 67.9% (95% CI: 59.0–75.6%) for LC-6 () and 59.9% (95% CI: 47.9–70.9%) for LC-12 () separately. LITT provided more favorable local control efficacy in RN than BM recurrence (LC-6: 87.4% vs. 67.9%, p = 0.009; LC-12: 76.3% vs. 59.9%, p = 0.041) ().
Figure 3. Local control efficacy of LITT for different pathological entities, including 6-month local control rate (a) and 12-month local control rate (b). RN: radiation necrosis; BM recurrence: brain metastasis recurrence.
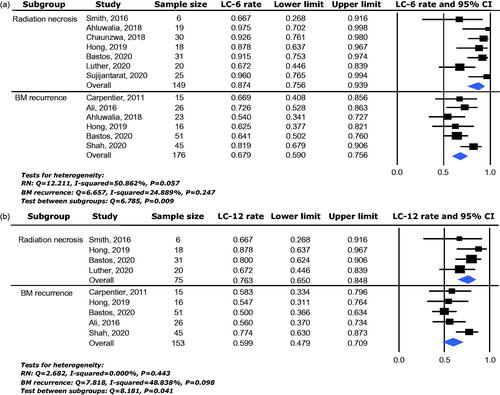
With the median pre-operative lesion volume 4.6 cm3 as the cutoff point, a subgroup analysis regarding pre-operative lesion volume was carried out from 321 lesions enrolled in eight studies. And a trend was noted favoring studies with lower median pre-operative lesion volume on LC-6 without reaching statistical significance (81.8% vs 78.3%, p = 0.709) (Supplementary Figure S1).
Overall survival
Overall survival (OS) was available for analysis from a total of 410 patients enrolled in 11 studies. The pooled median OS was 17.15 months (95% CI: 13.27–24.8). The 6-month overall survival rate (OS-6) ranged between 52.3% and 96.0% and the pooled effect was 76.0% (95% CI: 71.4–80.0%) as determined by the fixed-effects model (heterogeneity analysis: I2 = 46.10%, p = 0.059) (). The 12-month overall survival rate (OS-12) ranged between 26.1% and 84% and the pooled effect was 63.4% (95% CI: 52.9–72.7%) as determined by the random-effects model (heterogeneity analysis: I2 = 68.20%, p = 0.001) ().
Figure 4. Overall survival benefit of LITT for BMs with IFR following SRS, including 6-month overall survival rate (a) and 12-month overall survival rate (b).
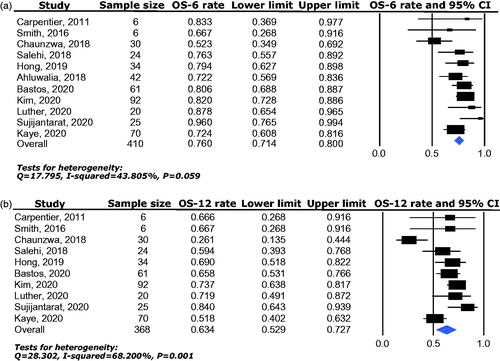
Different pathological entities were further analyzed. In RN subgroup, 141 patients enrolled in seven studies were available for OS-6 analysis and 122 patients enrolled in six studies for OS-12 analysis. The pooled effects were 83.1% (95% CI: 68.4–91.8%) for OS-6 () and 66.8% (95% CI: 49.1–80.8%) for OS-12 () separately. In BM recurrence subgroup, 85 patients enrolled in four studies were available for OS-6 analysis and 65 patients enrolled in three studies for OS-12 analysis. The pooled effects were 69.2% (95% CI: 58.5–78.2%) for OS-6 () and 66.5% (95% CI: 54.3–76.9%) for OS-12 () separately. No significant difference between RN and BM recurrence (66.8% vs. 66.5%, p = 0.978) was detected in OS-12 (); however, a trend favoring RN subgroup was noted in OS-6 (83.1% vs. 69.2%, p = 0.104) ().
Discussion
Due to advances in systemic treatments which allow better control of extracerebral disease and prolong patients overall survival, practitioners are encountering a growing number of patients with BMs failed first-line treatment, particularly those relapse after surgical resection and SRS [Citation45]. Limited salvage local control options are available, especially when lesions are not amenable for resection or patients cannot tolerate surgery. LITT is an emerging minimally invasive procedure that consists of a laser probe inserted under stereotactic guidance and destroys tissue through generation of heat. Real-time intraoperative magnetic resonance thermal imaging (MRTI) qualitatively allows the technique to create thermal ablation safely in deep-seated and high eloquent regions [Citation49].
In this study, we showed that LITT is a viable salvage option for IFR of brain metastases following SRS with 12-month local control rate of 69.0% (95% CI: 60.0–76.7%) and median OS of 17.15 months (95% CI: 13.27–24.8). Comparing with surgical resection with 12-month local control rate ranging from 62% to 93% and median OS of 8.7 months after surgery [Citation7–9], LITT shows comparable local control rate but more satisfactory overall survival benefit.
In field recurrence following SRS can be attributable to BM recurrence or RN or a combination of both. Our study showed that LITT provided more satisfactory local control efficacy on RN than BM recurrence with higher LC-6 (87.4% vs. 67.9%, p = 0.009) and LC-12 (76.3% vs. 59.9%, p = 0.041). Tumor recurrence is greatly contributed to tumor stem cell, which is resistant to thermal damage and overexpression of heat shock protein family might involve in the mechanism of thermoresistance [Citation50,Citation51]. RN has been described as a radiation dose-dependent central area necrosis surrounded by vascular hyalinization [Citation52]. As heat diffuses rapidly in necrotic tissue with higher water content, higher temperature is achieved insider the RN lesion and in the perilesional area [Citation53], which may contribute to higher local control efficacy in RN.
It is quite challenging to distinguish BM recurrence from RN. No standard has been established on radiographic modalities for differentiation and histopathology study is still considered as the gold standard. But pre-ablation biopsy during LITT was not performed routinely in some studies, which took MRI as the differentiation modality or didn’t distinguish the two pathological entities. Some researchers argued that sampling bias in needle biopsy specimen may result in an inaccurate diagnosis while possible bleeding or air entry during biopsy could impair the visualization of ablation extension during MRTI [Citation45]. Since effectiveness of LITT on RN was proven to be better than BM recurrence in several studies [Citation19,Citation25,Citation27] and biopsy result is paramount for guiding post-treatment decision-making, it is recommended to preform pre-ablation biopsy routinely [Citation32].
Although LITT showed more satisfactory local control efficacy on RN than BM recurrence, the overall survival benefits of the two pathological entities did not present the same pattern. The higher overall survival rate on RN compared with BM recurrence at 6 months follow up (83.1% vs. 69.2%, p = 0.104) did not extend to 12 months follow up (66.8% vs. 66.5%, p = 0.978). Although LITT provided good local control of intracranial diseases, control of extracerebral disease was the main determinator of BM patients overall survival time.
Besides pathological type, pre-operative lesion volume was reported as predictive factor correlated with local control efficiency in two studies [Citation23,Citation27] and extent of ablation was reported as predictive factor in six studies [Citation10,Citation15,Citation23,Citation27,Citation31,Citation32]. In our study, sub-group analysis showed higher 6-month local control rate in studies with mean pre-operative lesion volume < 4.6 cm3 for LC-6 (81.8% vs 74.3%). Beechar et al. showed that pre-operative lesion volume played a significant role in determining response to LITT with smaller lesions responding better to LITT than lesions with larger volumes [Citation20]. Although extent of ablation was not analyzed due to wide variability of data, several studies showed that higher extent of ablation was associated with improved local control and OS [Citation10,Citation15,Citation23,Citation27,Citation31,Citation32]. The correlation between lesion volume and local control/survival was partially caused by higher extent of ablation in small lesions [Citation27]. Because a single laser trajectory has a maximal diameter of 4 cm, smaller lesions have more favorable thermal damage threshold coverage compared with their larger counterparts [Citation33]. Currently, the ideal lesion to be treated with LITT is a circumscribed lesion with a diameter less than 3 cm [Citation32,Citation45]. For larger lesions, multiple fibers could be used to cover the entire target, but this must be balanced against the increased risk for malignant cerebral edema and neurological deficits, particularly for lesions located near critical structure [Citation45].
For larger lesions or lesions with complex geometry that may not be ablated completely, there may be a role for adjuvant SRS for the residual and for systemic therapy in the post-LITT period. Ali et al. [Citation15] showed that BM recurrence with extent of ablation <80% had higher risk of recurrence, but adjuvant hypofractionated SRS 1 months later could enhance the efficacy of LITT. Bastos et al. [Citation27] showed that patients receiving systemic therapy within 3 months after LITT had a longer freedom of local recurrence. Radiological study with dynamic contrast-enhancement MRI showed that hyperthermia caused by LITT might be able to induce disruption of the BBB and the peak of permeability occurred in one to two weeks and resolved in four to six weeks after LITT [Citation54]. Validation of the synergistic effect between LITT and adjuvant therapy in future studies is warranted.
This study must be interpreted in the context of certain limitations. Firstly, most of the included studies are retrospective and enrolled small case series. Secondly, significant heterogeneity exists in the studies enrolled. Substantial efforts were made to explore the possible causes for heterogeneity and found that different pathological entities (RN vs. BM recurrence) could explain the heterogeneity adequately. Random-effects model was used when heterogeneity exists within a group to minimize the bias. Thirdly, a lack of standardized definition on local control across different series represents a potential source of bias and should be addressed by consensual use of objective criteria such as the RANO-BM in future studies.
Conclusion
For brain metastasis patients experiencing IFR following SRS, LITT is an effective salvage treatment option with 6-month local control rate of 78.5% and 12-month local control rate of 69.0%. For different pathological entities, LITT showed more satisfactory local control efficacy on RN than BM recurrence.
Supplemental Material
Download EPS Image (1.1 MB)Disclosure statement
No potential conflict of interest was reported by the author(s).
Additional information
Funding
Reference
- Achrol AS, Rennert RC, Anders C, et al. Brain metastases. Nat Rev Dis Primers. 2019;5(1):5.
- Brown PD, Jaeckle K, Ballman KV, et al. Effect of radiosurgery alone vs radiosurgery with whole brain radiation therapy on cognitive function in patients with 1 to 3 brain metastases: a randomized clinical trial. JAMA. 2016;316(4):401–409.
- Mahajan A, Ahmed S, McAleer MF, et al. Post-operative stereotactic radiosurgery versus observation for completely resected brain metastases: a single-centre, randomised, controlled, phase 3 trial. Lancet Oncol. 2017;18(8):1040–1048.
- Higuchi Y, Yamamoto M, Serizawa T, et al. Modern management for brain metastasis patients using stereotactic radiosurgery: literature review and the authors’ gamma knife treatment experiences. Cancer Manag Res. 2018;10:1889–1899.
- Christ SM, Mahadevan A, Floyd SR, et al. Stereotactic radiosurgery for brain metastases from malignant melanoma. Surg Neurol Int. 2015;6(13):355–365.
- Telera S, Fabi A, Pace A, et al. Radionecrosis induced by stereotactic radiosurgery of brain metastases: results of surgery and outcome of disease. J Neurooncol. 2013;113(2):313–325.
- Vecil GG, Suki D, Maldaun MV, et al. Resection of brain metastases previously treated with stereotactic radiosurgery. J Neurosurg. 2005;102(2):209–215.
- Truong MT, St Clair EG, Donahue BR, et al. Results of surgical resection for progression of brain metastases previously treated by gamma knife radiosurgery. Neurosurgery. 2006;59(1):86–97;discussion 86-97.
- Kano H, Kondziolka D, Zorro O, et al. The results of resection after stereotactic radiosurgery for brain metastases. J Neurosurg. 2009;111(4):825–831.
- Carpentier A, McNichols RJ, Stafford RJ, et al. Laser thermal therapy: real-time MRI-guided and computer-controlled procedures for metastatic brain tumors. Lasers Surg Med. 2011;43(10):943–950.
- Torres-Reveron J, Tomasiewicz HC, Shetty A, et al. Stereotactic laser induced thermotherapy (LITT): a novel treatment for brain lesions regrowing after radiosurgery. J Neurooncol. 2013;113(3):495–503.
- Fabiano AJ, Alberico RA. Laser-interstitial thermal therapy for refractory cerebral edema from post-radiosurgery metastasis. World Neurosurg. 2014;81(3–4):652.e1–4.
- Rao MS, Hargreaves EL, Khan AJ, et al. Magnetic resonance-guided laser ablation improves local control for postradiosurgery recurrence and/or radiation necrosis. Neurosurgery. 2014;74(6):658–667; discussion 67.
- Sun XR, Patel NV, Danish SF. Tissue ablation dynamics during magnetic resonance-guided, laser-induced thermal therapy. Neurosurgery. 2015;77(1):51–58. discussion 8.
- Ali MA, Carroll KT, Rennert RC, et al. Stereotactic laser ablation as treatment for brain metastases that recur after stereotactic radiosurgery: a multiinstitutional experience. FOC. 2016;41(4):E11.
- Patel P, Patel NV, Danish SF. Intracranial MR-guided laser-induced thermal therapy: single-center experience with the Visualase thermal therapy system. J Neurosurg. 2016;125(4):853–860.
- Smith CJ, Myers CS, Chapple KM, et al. Long-term follow-up of 25 cases of biopsy-proven radiation necrosis or post-radiation treatment effect treated with magnetic resonance-guided laser interstitial thermal therapy. Neurosurgery. 2016;79 Suppl 1:S59–s72.
- Kamath AA, Friedman DD, Hacker CD, et al. MRI-guided interstitial laser ablation for intracranial lesions: a large single-institution experience of 133 cases. Stereotact Funct Neurosurg. 2017;95(6):417–428.
- Ahluwalia M, Barnett GH, Deng D, et al. Laser ablation after stereotactic radiosurgery: a multicenter prospective study in patients with metastatic brain tumors and radiation necrosis. J Neurosurg. 2018;130(3):804–811.
- Beechar VB, Prabhu SS, Bastos D, et al. Volumetric response of progressing post-SRS lesions treated with laser interstitial thermal therapy. J Neurooncol. 2018;137(1):57–65.
- Chaunzwa TL, Deng D, Leuthardt EC, et al. Laser thermal ablation for metastases failing radiosurgery: a multicentered retrospective study. Neurosurgery. 2018;82(1):56–63.
- Rammo R, Asmaro K, Schultz L, et al. The safety of magnetic resonance imaging-guided laser interstitial thermal therapy for cerebral radiation necrosis. J Neurooncol. 2018;138(3):609–617.
- Salehi A, Kamath AA, Leuthardt EC, et al. Management of intracranial metastatic disease with laser interstitial thermal therapy. Front Oncol. 2018;8:499.
- Hernandez RN, Carminucci A, Patel P, et al. Magnetic resonance-guided laser-induced thermal therapy for the treatment of progressive enhancing inflammatory reactions following stereotactic radiosurgery, or PEIRs, for metastatic brain disease. Neurosurgery. 2019;85(1):84–90.
- Hong CS, Deng D, Vera A, et al. Laser-interstitial thermal therapy compared to craniotomy for treatment of radiation necrosis or recurrent tumor in brain metastases failing radiosurgery. J Neurooncol. 2019;142(2):309–317.
- Swartz LK, Holste KG, Kim MM, Morikawa A, et al. Outcomes in patients treated with laser interstitial thermal therapy for primary brain cancer and brain metastases. Oncologist. 2019;24(12):e1467–e1470.
- Bastos DCA, Rao G, Oliva ICG, et al. Predictors of local control of brain metastasis treated with laser interstitial thermal therapy. Neurosurgery. 2020;87(1):112–122.
- Ginalis EE, Danish SF. Magnetic resonance-guided laser interstitial thermal therapy for brain tumors in geriatric patients. Neurosurg Focus. 2020;49(4):E12.
- Kaye J, Patel NV, Danish SF. Laser interstitial thermal therapy for in-field recurrence of brain metastasis after stereotactic radiosurgery: does treatment with LITT prevent a neurologic death? Clin Exp Metastasis. 2020;37(3):435–444.
- Kim AH, Tatter S, Rao G, et al. Laser Ablation of Abnormal Neurological Tissue Using Robotic NeuroBlate System (LAANTERN): 12-month outcomes and quality of life after brain tumor ablation. Neurosurgery. 2020;87(3):E338–E346.
- Luther E, McCarthy D, Shah A, et al. Radical laser interstitial thermal therapy ablation volumes increase progression-free survival in biopsy-proven radiation necrosis. World Neurosurg. 2020;136:e646–e659.
- Shah AH, Semonche A, Eichberg DG, et al. The role of laser interstitial thermal therapy in surgical neuro-oncology: series of 100 consecutive patients. Neurosurgery. 2020;87(2):266–275.
- Shao J, Radakovich NR, Grabowski M, et al. Lessons learned in using laser interstitial thermal therapy for treatment of brain tumors: a case series of 238 patients from a single institution. World Neurosurg. 2020;139:e345–e354.
- Sujijantarat N, Hong CS, Owusu KA, et al. Laser interstitial thermal therapy (LITT) vs. bevacizumab for radiation necrosis in previously irradiated brain metastases. J Neurooncol. 2020;148(3):641–649.
- McGrath S, Zhao X, Qin ZZ, et al. One-sample aggregate data meta-analysis of medians. Stat Med. 2019;38(6):969–984.
- Carpentier A, McNichols RJ, Stafford RJ, et al. Real-time magnetic resonance-guided laser thermal therapy for focal metastatic brain tumors. Neurosurgery. 2008;63(1 Suppl 1):ONS21–ONS28; discussion ONS8-9.
- Hawasli AH, Ray WZ, Murphy RK, et al. Magnetic resonance imaging-guided focused laser interstitial thermal therapy for subinsular metastatic adenocarcinoma: technical case report. Neurosurgery. 2012;70(2 Suppl Operative):332–337. discussion 8.
- Jethwa PR, Barrese JC, Gowda A, et al. Magnetic resonance thermometry-guided laser-induced thermal therapy for intracranial neoplasms: initial experience. Neurosurgery. 2012;71(1 Suppl Operative):133–144;144–145.
- Rahmathulla G, Recinos PF, Valerio JE, et al. Laser interstitial thermal therapy for focal cerebral radiation necrosis: a case report and literature review. Stereotact Funct Neurosurg. 2012;90(3):192–200.
- Hawasli AH, Bagade S, Shimony JS, et al. Magnetic resonance imaging-guided focused laser interstitial thermal therapy for intracranial lesions: single-institution series. Neurosurgery. 2013;73(6):1007–1017.
- Patel NV, Jethwa PR, Barrese JC, et al. Volumetric trends associated with MRI-guided laser-induced thermal therapy (LITT) for intracranial tumors. Lasers Surg Med. 2013;45(6):362–369.
- Sharma M, Balasubramanian S, Silva D, et al. Laser interstitial thermal therapy in the management of brain metastasis and radiation necrosis after radiosurgery: an overview. Expert Rev Neurother. 2016;16(2):223–232.
- Alattar AA, Bartek J, Jr., Chiang VL, et al. Stereotactic laser ablation as treatment of brain metastases recurring after stereotactic radiosurgery: a systematic literature review. World Neurosurg. 2019;128:134–142.
- Salem U, Kumar VA, Madewell JE, et al. Neurosurgical applications of MRI guided laser interstitial thermal therapy (LITT). Cancer Imaging. 2019;19(1):65.
- Bastos DCA, Weinberg J, Kumar VA, et al. Laser interstitial thermal therapy in the treatment of brain metastases and radiation necrosis. Cancer Lett. 2020;489:9–18.
- Holste KG, Orringer DA. Laser interstitial thermal therapy. Neurooncol Adv. 2020;2(1):vdz035.
- Hong CS, Beckta JM, Kundishora AJ, et al. Laser interstitial thermal therapy for treatment of cerebral radiation necrosis. Int J Hyperthermia. 2020;37(2):68–76.
- Hong CS, Kundishora AJ, Elsamadicy AA, et al. Laser interstitial thermal therapy in neuro-oncology applications. Surg Neurol Int. 2020;11:231.
- Kahn T, Harth T, Kiwit JC, et al. In vivo MRI thermometry using a phase-sensitive sequence: preliminary experience during MRI-guided laser-induced interstitial thermotherapy of brain tumors. J Magn Reson Imaging. 1998;8(1):160–164.
- Rylander MN, Feng Y, Bass J, et al. Thermally induced injury and heat-shock protein expression in cells and tissues. Ann N Y Acad Sci. 2005;1066:222–242.
- Burke AR, Singh RN, Carroll DL, et al. The resistance of breast cancer stem cells to conventional hyperthermia and their sensitivity to nanoparticle-mediated photothermal therapy. Biomaterials. 2012;33(10):2961–2970.
- Yoshii Y. Pathological review of late cerebral radionecrosis. Brain Tumor Pathol. 2008;25(2):51–58.
- Ansari MA, Erfanzadeh M, Mohajerani E. Mechanisms of laser-tissue interaction: II. Tissue thermal properties. J Lasers Med Sci. 2013;4(3):99–106.
- Leuthardt EC, Duan C, Kim MJ, et al. Hyperthermic laser ablation of recurrent glioblastoma leads to temporary disruption of the peritumoral blood brain barrier. PloS One. 2016;11(2):e0148613.