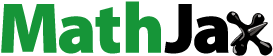
Abstract
Purpose
This study aimed to explore the accuracy of different imaging methods for lesion volume estimation pre- and post-microwave ablation (MWA) as compared with that of pathological examination.
Methods
We used the VX2 cell line to establish the VX2 lung tumor model in rabbits, followed by MWA of the tumor. The imaging features of the VX2 tumors were documented. The volume of the tumors and the ablated lesions were measured and compared across imaging methods, using the pathological examination as reference.
Results
Tumors were successfully developed in 11 rabbits (age, 13.91 ± 1.38 weeks; weight, 2.15 ± 0.56 kg). The mean volume of the tumors was 2.05 ± 1.88 cm3. CT showed the strongest correlation with the pathologic examination results (r = 0.998, p<.001). MWA created three-layered structures that were delineated on MRI. The mean volume of the post-ablation lesion was 10.39 ± 8.93 cm3, and the measurement of the post-ablation volume on 3D-VIBE-T1WI showed the strongest correlation with the pathologic examination results (r = 0.991, p<.001).
Conclusion
Both CT and MRI are capable of depicting lung tumors. In terms of post-ablation evaluation, MR images could provide more versatile information. The 3D-VIBE-T1WI sequence provides more precise lesion volume evaluation after ablation compared with other methods.
Introduction
Lung cancer has been acknowledged as one of the leading causes of cancer-related deaths; non-small-cell lung cancer (NSCLC) constitutes approximately 80% of primary lung cancer [Citation1]. Curative resection has been established as the standard therapy for clinical stage I NSCLC. Cautious preoperative assessment of patients’ overall condition, particularly the patient’s pulmonary reserve and comorbidities, is essential for evaluating surgical outcomes [Citation2]. Patients with early-stage NSCLC are candidates for local therapies, such as thermal ablation and stereotactic ablative radiotherapy (SABR), when they do not meet the criteria for resection. Kim et al. [Citation3] reported that radiofrequency ablation (RFA) yielded similar results as lobectomy in terms of overall survival (OS). In the 2016 National Comprehensive Cancer Network guidelines for NSCLC, SABR and thermal ablation were recommended as alternative options for inoperable stage I NSCLC patients.
Several studies have indicated that complications associated with pulmonary tumor ablation were minimal, and the local control rate was impressive when tumors were 30 mm or less in diameter [Citation4]. Microwave ablation (MWA) has emerged as an advanced thermal ablative method in the past decade [Citation5]. In a phase III randomized controlled trial, MWA obtained similar results as RFA in hepatocellular carcinoma patients but required a shorter ablation duration and fewer sessions [Citation6].
To the best of our knowledge, few studies have focused on the immediate evaluation of pulmonary tumors after ablation. In this process, a safety margin layer, which consists of ablated normal lung tissue, is created around the tumor. Thus, the area of the ablated lesion is larger than that of the original tumor mass and shrinks with time. On follow-up images, some radiologists may find it difficult to distinguish the scarred tissue from the residual tumor or recurrence when the size of the lesion remains the same on CT images, despite the use of contrast-enhancement. Accurate assessment of MWA outcome is of great importance, since the residues can then be treated as early as possible. We reasoned that, since magnetic resonance imaging (MRI) offered better soft tissue contrast than CT [Citation7], functional MRI could provide additional information about lesions, without concerns about radiation exposure, MRI may be an alternative for post-MWA assessment. The advantages of MRI on post-MWA assessment have been proven in a few studies [Citation8,Citation9], but most of these studies lacked pathologic verification.
Consequently, in this study, we documented the imaging features of pulmonary VX2 tumor pre- and post-MWA on CT and images obtained with two MRI sequences, and compared these features with those observed on pathological examination.
Materials and methods
This study was approved by the Laboratory Animal Ethics Review Committee of Fujian Medical University and was conducted on 15 male New Zealand white rabbits (Wu’s Animal Experimental Center, Fuzhou city, China) weighing 2.0‒2.5 kg. All procedures were performed while the rabbits were under general anesthesia induced by an intramuscular injection (1 ml/kg) of ketamine (50 mg/ml, Fujian Gutian Pharmaceutical Co., Ltd., Ningde, China).
VX2 cell preparation
The VX2 cell line used for tumor implantation was bought from Shanghai Aolu Biotechnology Co., Ltd. (Shanghai, China). Tumors previously created from a VX2 cell line and maintained in the thigh muscle of rabbits were stripped and minced to 1-mm pieces. These pieces were filtered through a metal mesh to obtain a tumor cell suspension. These cells were then centrifuged at 1000 r/s for 5 min and resuspended in a saline solution.
Establishment of the rabbit VX2 lung tumor model
Fifteen rabbits were fasted for 6 h before the procedure. They were positioned prone, under general anesthesia with ketamine as described above. Under a multidetector row CT scanner guidance (Somatom Emotion, Siemens, Munich, Germany), an 18-gauge needle was used to puncture the lung percutaneously and the tip was located in the left/right lower lobe. Then, we injected 0.4 ml of the VX2 cell suspensions through a 21-gauge needle placed coaxially to the target. To reduce the mortality and the infection rates of the procedure, all rabbits received implantation in only one lung. One week later, plain CT scans confirmed that tumors had developed in 11 rabbits (73.33%), which were assigned to the experimental group. The remaining four rabbits were assigned to the control group. Two to three weeks later, two rabbits died of pulmonary infection (one in the experimental group and one in the control group). The rabbits in the experimental group were scanned with CT and MRI to record tumorigenesis. One rabbit in the experimental group was sacrificed for gross specimen examination and tumor confirmation.
Microwave ablation
An MTC-3C microwave generator (VikingJiu Zhou Institute, Nanjing, China) that can produce a maximum of 100 W power at 2450 MHz was used for MWA. The microwave antenna (MTC-3CA-II25, VikingJiu Zhou Institute, Nanjing, China) used was 14 gauge, with a 5-mm-long active-tip. Twelve rabbits, including nine in the experimental group and three in the control group, received MWA under CT guidance. Parameters for the CT scanner were as follows: tube voltage, 100 kVp; tube current, 80 mA; slice thickness, 3.0 cm at 1.0 mm intervals; and field of view, 18 cm × 18 cm. After general anesthesia, the microwave antenna was used to puncture into the VX2 tumor in the left or right lower lobe, percutaneously. In the control group, the antenna targeted normal tissue in the basal segment of the left or the right lower lobe. When the correct position was confirmed, a microwave generator was connected to the antenna with a coaxial cable. The energy output was set at 40‒60 W, and ablation lasted about 3‒5 min, according to the tumor size. We defined the endpoint of the ablation as when the ablated area fully covered the tumor and extended over the edge by 5‒10 mm. After MWA, the rabbit was transferred to a 1.5-T MRI scanner (Magnet Espree, Siemens, Munich, Germany) for immediate imaging assessment. The rabbit was put in the prone position, and a lumbar corset was used to restrain the breath. To reduce the scan time and to acquire relatively high-resolution images, we chose the 3D-VIBE-T1WI sequence without breath hold and FS-TSE-T2WI sequence with gating technique. Parameters for the two sequences are listed in . The signal characteristics of the ablated lesion were recorded. Then, the rabbit was sacrificed for pathological examination.
Table 1. MRI sequences and parameters.
MRI sequences and parameters
MRI sequences and parameters used for lesion assessments are listed in .
Pathological examination
The rabbits’ lungs were sliced along the trajectory of the antenna, fixed with 10% formalin, embedded in paraffin, and stained with hematoxylin and eosin (HE) for pathological examination.
Volume evaluation
Both CT and MR images before and after MWA were transferred to the picture archiving and communication system and imaging reconstructions were produced by using an image viewing system (Radiant, MEDIXANT MACIEJ FRANKIEWICZ, Poznan, Poland). The area of every slice of the lesion was calculated using the region of interest (ROI) method. The lesion volume is determined by integrating the products of the slice area and the scan spacing.
We assumed the tumors and the ablated areas were ellipsoid in shape and calculated their volumes using the following equation [Citation10]:
where v is the volume, l is the maximal length, w is the width and h is the height, which can be calculated using the equation:
On gross tissues, we measured the longest diameter of the lesions and the longest perpendicular diameter on the same sections that were transaxially sliced along the trajectory of the antenna, and the height of the lesions was approximated using the equation mentioned above.
Percentage error calculation
The absolute percentage (%) errors of each imaging method on lesion volume evaluation relative to the pathological examination were defined by the following formula:
where Vi is the estimated volume of imaging and Vp is the volume of pathological examination.
Statistical analysis
Statistical analyses were performed using R language (version 3.6.2, The R Foundation, Vienna, Austria; https://www.r-project.org/foundation/). All continuous data are presented as mean ± standard deviation (SD). One-way analysis of variance (ANOVA) was performed to test the differences in the volume of the tumors and of the ablated lesions, and Tukey's honest significant difference (Tukey’s HSD) test was used for multiple pairwise-comparison. p<.05 was considered to indicate statistical significance.
Results
Pathological characteristics of the VX2 tumors
The VX2 tumors were successfully developed in 11 rabbits. General information of the rabbits included in this study is listed in . The gross specimen and the findings of the pathological examination of a VX2 tumor before MWA are presented in . The tumors were yellow-white in color on the section surface. Most tumors were lobulated, and the mean diameter was 1.83 ± 0.52 cm. Most tumors had clear boundaries and could be demarcated.
Figure 1. Gross specimen and pathological examination of a VX2 tumor after confirmation of successful tumor implantation. (A) The VX2 tumor located in the left lower lobe of the lung (arrow, scale bar = 1 cm). (B) Under the light microscope, the tumor cells occupied most of the lung parenchyma (T), and the tumor even invaded nearby vessels (V) and alveoli (L) (hematoxylin and eosin staining, ×10).
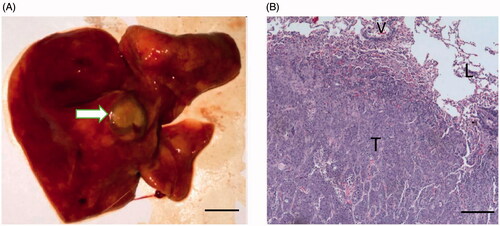
Table 2. General information of rabbits.
Normal lung microstructure was barely found at the region that was occupied by the tumor cells, using a low-power light microscope. The tumor cells were nested and some cells invaded the alveoli and nearby vessels. The adjacent lung parenchyma was filled with effusion.
Pathological changes after the ablation
After MWA, the tumors demonstrated three-layered structures, as presented in , including the following: a central necrotic area, which was mainly composed of cytoplasm that demonstrated acidophilic changes and concentrated nuclei, while no normal lung microstructure or tumor cell structure was distinguishable; an intermediate area, in which the pulmonary alveoli were filled with effusion; and the outermost layer, which was composed of congested lung tissue, in which ghost red blood cells caused by thermal injury were found.
Figure 2. Pathological examination of the ablated area and the peripheral lung tissue. (A) After hematoxylin and eosin staining (HE staining), the ablated area demonstrated three layers, including the central necrotic area (tagged with N, scale bar = 500 μm), the intermediate area (tagged with E) and the outermost layer (tagged with C), shown in B (scale bar = 500 μm). (C) At the outermost area, ghost red blood cells caused by thermal injury were observed (white arrow, HE staining, scale bar = 100 μm).
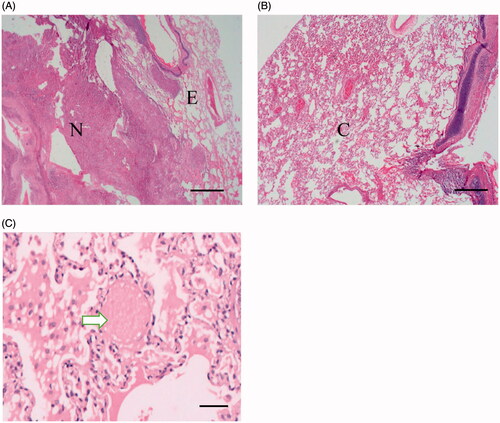
Imaging features of the VX2 tumors on CT and MRI
On CT images (), the tumors demonstrated a lobulated mass located in a single lower lobe of the lungs. The boundary between the tumors and lung tissues was clear. On 3D-VIBE-T1WI images (), the tumors demonstrated isointensity as compared with muscle, and even when the boundary was visible, the lung texture was nearly invisible. As with 3D-VIBE-T1WI, lung texture remained invisible on FS-TSE-T2WI images (). The tumors demonstrated high signal intensity, and the boundary was vague. Lung tissues that surrounded the tumors were affected, and they demonstrated a higher signal intensity, corresponding to the alveolar effusion () noted in the microscopic examination.
Figure 3. Comparison of imaging features of the VX2 tumor on CT and MRI pre- and post-MWA (scale bar = 1 cm). (A) The VX2 tumor was successfully implanted in the left lower lobe of the lung (arrow). (B) On 3D-VIBE-T1WI image, the tumor showed isointensity as compared with muscle (arrow). (C) On FS-TSE-T2WI image, the tumor showed hyperintensity as compared with muscle (arrow). Lung texture was barely visible on either 3D-VIBE-T1WI or on TS-FSE-T2WI image. (D) After the ablation, ground-glass opacity (arrow) was seen covering the tumor (triangle), and the border of the tumor was vague. (E) The tumor showed high signal intensity on 3D-VIBE-T1WI image after ablation (arrow) with a small area of centered isointensity (curve arrow). (F) The signal decreased on TS-FSE-T2WI image post-ablation (triangle). The ablation area demonstrated central low signal intensity, which corresponded to the thermally coagulated area in the pathological examination. The central area was surrounded by a high signal intensity rim (curve arrow) that could possibly correspond to the effusion area, while the outermost area showed higher signal intensity that could correspond to the congestion area (arrow).
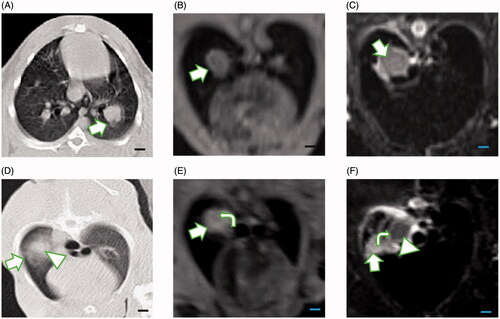
After MWA, on CT images, ground glass opacities formed, covering the tumors, and the boundary of the ablated lesion extended 5‒10 mm from the edge of the tumors. In some cases, it was difficult to differentiate between tumors and the thermally injured normal tissues (). On the 3D-VIBE-T1WI images, the tumors were fully covered by the ablated lesion, demonstrating a hyperintensity signal, which may represent the necrotic area, and a rim of isointensity signal area surrounding the ablated lesion, representing edema and congested areas (). Elimination of the tumor cells defined successful ablation that led to necrosis and was demonstrated as decreased signal intensity on FS-TSE-T2WI images (). The three-layered structures were clear on FS-TSE-T2WI images.
Pre- and post-ablation volume changes
The mean pre-MWA volume of the tumors was 2.05 ± 1.88 cm3 (0.49‒6.63 cm3) in the pathological examination that was used as the reference standard, and the volumes measured on different imaging methods were as follows: 2.10 ± 2.06 cm3 (0.61‒6.96 cm3) on CT images, 2.05 ± 1.61 cm3 (0.39‒5.55 cm3) on 3D-VIBE-T1WI images and 2.34 ± 1.96 cm3 (0.73‒6.88 cm3) on FS-TSE-T2WI images, respectively (). According to ANOVA, the volume was consistent across the different imaging methods (p=.98) (), and further analysis with Tukey’s HSD test for multiple pairwise-comparison showed no significant difference across all imaging methods. However, in correlation analysis, we found that the volumes measured on CT images had the strongest correlation with the volumes determined by pathological analysis (r = 0.977, p<.001), and the coefficients were 0.968 for 3D-VIBE-T1WI images and 0.961 for FS-TSE-T2WI images, respectively. The absolute mean percentage error of the volumes estimated on CT was the smallest across imaging methods relative to the pathological examination, which was 26.13% with uncertainty of 17%, and the value for 3D-VIBE-T1WI was 27.32%±27% and 40.25%±27% for FS-TSE-T2WI. We calculated error propagation to check the uncertainties of tumor volume calculated by the manual measurement of each slice area using various methods. The steadiest method for tumor volume evaluation was CT (measured volume: 1.47 ± 1.17 cm3), followed by 3D-VIBE-T1WI (measured volume: 1.59 ± 1.09 cm3), and finally, FS-TSE-T2WI (measured volume: 1.67 ± 1.17 cm3).
Figure 4. Boxplot showing the volumes of the VX2 tumors measured before microwave ablation, based on different imaging methods. Comparison of multiple-group means by ANOVA showed no statistically significant difference (p=.98). Note: We tagged the median with the blue rhombus. The upper boundary of the boxplot represents the third quartile of our data set, and the lower boundary represents the first quartile of our data set. The whiskers line indicates variability outside the upper and lower quartiles. The blue rhombus represents the median value of the data set.
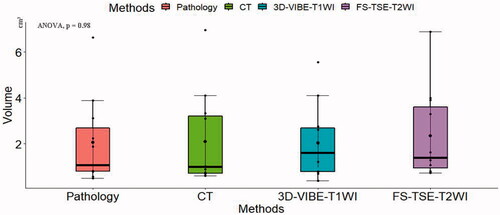
Table 3. The tumor volume on CT/MRI and pathological examinations.
The volume of the lesion was commonly larger after MWA than before ablation. The post-ablation volume was 10.39 ± 8.93 cm3 (1.20‒27.98 cm3) on pathological examination. The mean volume measured on FS-TSE-T2WI images was 11.38 ± 7.40 cm3 (3.24‒24.28 cm3), which was the largest as compared with the results of the other imaging methods. The mean volumes were 11.12 ± 10.28 cm3 (0.95‒29.38 cm3) on 3D-VIBE-T1WI images and 11.27 ± 7.85 cm3 (2.29‒26.16 cm3) on CT images. Nevertheless, there was no significant difference in the volume as measured with different imaging methods (p=.99) () and multiple pairwise-comparison also revealed no statistically significant differences. In correlation analysis, volumes determined on 3D-VIBE-T1WI images presented the strongest correlation (r = 0.991, p<.001) with that determined on pathological examination, while CT images presented the weakest correlation (r = 0.961, p<.001) (). The absolute mean percentage error of the volumes estimated on 3D-VIBE-T1WI was the smallest across imaging methods relative to the pathological examination, which was 21.29% with uncertainty of 10%, and the value for CT was 41.18%±55% and 45.01%±53% for FS-TSE-T2WI. Unlike the results before ablation, 3D-VIBE-T1WI provided the steadiest results for lesion volume evaluation (measured volume: 6.44 ± 6.18 cm3), followed by FS-TSE-T2WI (measured volume: 6.64 ± 4.85 cm3), and finally, CT (measured volume: 6.94 ± 5.79 cm3).
Figure 5. Graphs showing correlations of the volumes of the ablated lesion measured with different imaging methods. Using the pathological examination as reference, in (A), the volumes of the ablated lesion measured on CT showed a positive linear correlation with the volumes determined by pathological examination, but their correlation was slightly weaker (r = 0.92) than that of the volumes determined by FS-TSE-T2WI (r = 0.96) (C). (B) The correlation with the pathologically determined volumes was the strongest for 3D-VIBE-T1WI (r = 0.99). The matrix of their correlations is showed in (D).
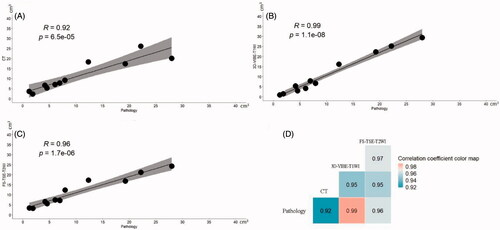
Table 4. The volume of ablated lesion on CT/MRI and pathological examinations.
Discussion
In aging societies, the incidence of pulmonary malignancies has been increasing. The number of patients that is unsuitable for curative resection is increasing proportionally due to inadequate pulmonary function or the fear of postoperative comorbidities. Further, pulmonary metastasis occurs in up to 40% of patients dying of malignancies, and can present even if only one lobe is affected [Citation11]. Thermal treatment of pulmonary tumors is an alternative to curative resection. Goldberg et al. proved the effectiveness of RFA on a VX2 tumor in a rabbit lung model [Citation12]. Consequently, Steinke et al. reported the first clinical research involving application of RFA on three patients with lung tumors, in 2000 [Citation13]. Since then, a number of clinical studies on the use of thermal ablation for lung malignancies have been reported [Citation8,Citation14,Citation15]. Accurate evaluation of the post-ablation response is a major concern regarding thermal ablation of lung malignancies. In most medical centers, CT is the main imaging method used for MWA planning and post-ablation evaluation. However, after ablation, it is difficult to distinguish the necrotic area from the thermally injured normal lung tissues, which is outlined as a uniform high-opacity area. In most cases, pulmonary tumors present as high attenuation regions on CT images and show a slight change in density after ablation. Some physicians may consider that the volume of tumors pre- and post-ablation remains unchanged. However, Yamamoto et al. showed that the volume of the necrotic area was smaller after ablation on pathological examination as compared with measurements on CT images [Citation16]. Thus, the imaging features of CT restrict its value for post-ablation evaluation in the lung. Hence, in this study, in order to find a better imaging method for post-ablation evaluation, we compared two MRI sequences with CT and with pathological evaluation. We show that both CT and MRI can be used to assess lesions pre-MWA, but that 3D-VIBE-T1WI is more precise for post-ablation evaluation compared with CT and FS-TSE-T2WI sequence.
MRI can provide detailed information on various lesions based on their signal intensity and high contrast soft-tissues images. MR images are susceptible to changes of tissue microstructure during thermal ablation. As shown in our study, MR images clearly depict the tumors and the areas of ablation. In our research, before ablation, the VX2 tumors demonstrated isointensity on 3D-VIBE-T1WI images and hyperintensity on FS-TSE-T2WI images. Then, after ablation, three-layered structures were observed on T2-weighted images. These layers included the central low signal intensity area, the intermediate high signal intensity, and the outermost area with higher signal intensity. However, there were only two layers noted on 3D-VIBE-T1WI images: the central high signal intensity area and the surrounding area that formed an isointense rim. Both MR sequences could provide versatile information. In terms of preventing local recurrence, under CT guidance, many reports recommend that the ground-glass opacity should extend 5‒10 mm beyond the tumor margin to ensure complete ablation [Citation17]. In contrast to CT, under MRI guidance, complete ablation refers to when the high signal intensity of the ablated area fully covers the tumor on 3D-VIBE-T1WI images, and when a hyperintense rim surrounds the tumor on FS-TSE-T2WI images. The existence of discrete areas of low signal intensity on 3D-VIBE-T1WI and an incomplete high signal intensity rim may indicate the possibility of residual tumor and that further treatment is required. While MRI could provide objective and direct information, the judgment of complete ablation on CT is sometimes difficult. Pathological changes like bleeding may obscure the ablated area.
CT is the imaging method conventionally used for screening and diagnosing lung disease. In this study, we measured the volumes change before and after ablation on CT and MRI, using the volumes determined by pathological examination as the reference standard. Before ablation, the difference in the volumes measured on CT, MRI and pathology specimens was not statistically significant. Due to the high-resolution of lung texture, the tumor volume estimated on CT showed the strongest correlation with that on pathological examination. However, after ablation, 3D-VIBE-T1WI showed the strongest correlation of volume estimation relative to pathological examination, and FS-TSE-T2WI showed the weakest correlation, although there was no statistically significant difference in the tumor volume estimated among the imaging methods. The main reason for the difference was that 3D-VIBE-T1WI images were sensitive to coagulative necrosis with lung tissue as the background, while changes of effusion and congestion in the lung tissue are more easily depicted on CT images and FS-TSE-T2WI images. Thus, the volumes we measured on 3D-VIBE-T1WI images were close to the real ablation-induced coagulative necrosis area and the volumes measured on CT and FS-TSE-T2WI images reflected a combination of necrosis area, effusion and hyperemia.
There are some limitations to this study. First, to balance the relation between the MRI scan time and image quality, we used only 3D-VIBE-T1WI sequence and FS-TSE-T2WI sequence. Other sequences like diffusion-weighted imaging will be applied in our future work. Second, only 12 rabbits received MWA in this study, which reduced the power of our conclusions. More rabbits should be included in future studies. Last, we did not perform a long-term follow-up CT or MRI or correlate them with pathological changes. This should be addressed in further studies.
Conclusion
Taken together, both CT and MRI are capable of depicting lung tumors. In terms of post-ablation evaluation, MR images could provide more versatile information. The 3D-VIBE-T1WI sequence allows more precise lesion volume evaluation after ablation as compared with other methods.
Disclosure statement
The authors report no conflicts of interest and are responsible for the content and writing of this article.
Data availability statement
The data that support the findings of this study are available on request from the corresponding author.
Correction Statement
This article has been republished with minor changes. These changes do not impact the academic content of the article.
Additional information
Funding
References
- Fidler MM, Bray F, Soerjomataram I. The global cancer burden and human development: a review. Scand J Public Health. 2018;46(1):27–36.
- Birim O, Kappetein AP, Goorden T, et al. Proper treatment selection may improve survival in patients with clinical early-stage nonsmall cell lung cancer. Ann Thorac Surg. 2005;80(3):1021–1026.
- Kim SR, Han HJ, Park SJ, et al. Comparison between surgery and radiofrequency ablation for stage I non-small cell lung cancer. Eur J Radiol. 2012;81(2):395–399.
- Simon CJ, Dupuy DE, DiPetrillo TA, et al. Pulmonary radiofrequency ablation: long-term safety and efficacy in 153 patients. Radiology. 2007;243(1):268–275.
- Murphy KP, Maher MM, O'Connor OJ. Abdominal ablation techniques. AJR Am J Roentgenol. 2015;204(5):W495–W502.
- Yu J, Yu XL, Han ZY, et al. Percutaneous cooled-probe microwave versus radiofrequency ablation in early-stage hepatocellular carcinoma: a phase III randomised controlled trial. Gut. 2017;66(6):1172–1173.
- Barile A, Zugaro L, Catalucci A, et al. Soft tissue liposarcoma: histological subtypes, MRI and CT findings. Radiol Med. 2002;104(3):140–149.
- Suh RD, Wallace AB, Sheehan RE, et al. Unresectable pulmonary malignancies: CT-guided percutaneous radiofrequency ablation-preliminary results. Radiology. 2003;229(3):821–829.
- Lin Z, Chen J, Yan Y, et al. Microwave ablation of hepatic malignant tumors using 1.5 T MRI guidance and monitoring: feasibility and preliminary clinical experience. Int J Hyperthermia. 2019;36(1):1216–1222.
- Sápi J, Kovács L, Drexler DA, et al. Tumor volume estimation and quasi-continuous administration for most effective bevacizumab therapy. PLoS One. 2015;10(11):e0142190.
- Davidson RS, Nwogu CE, Brentjens MJ, et al. The surgical management of pulmonary metastasis: current concepts. Surg Oncol. 2001;10(1–2):35–42.
- Goldberg SN, Gazelle GS, Compton CC, et al. Radio-frequency tissue ablation of VX2 tumor nodules in the rabbit lung. Acad Radiol. 1996;3(11):929–935.
- Steinke K, King J, Glenn DW, et al. Percutaneous radiofrequency ablation of lung tumors with expandable needle electrodes: tips from preliminary experience. AJR Am J Roentgenol. 2004;183(3):605–611.
- Herrera LJ, Fernando HC, Perry Y, et al. Radiofrequency ablation of pulmonary malignant tumors in nonsurgical candidates. J Thorac Cardiovasc Surg. 2003;125(4):929–937.
- Vogl TJ, Nour-Eldin NA, Albrecht MH, et al. Thermal ablation of lung tumors: focus on microwave ablation. Rofo. 2017;189(9):828–843.
- Yamamoto A, Nakamura K, Matsuoka T, et al. Radiofrequency ablation in a porcine lung model: correlation between CT and histopathologic findings. AJR Am J Roentgenol. 2005;185(5):1299–1306.
- Liu BD, Ye X, Fan WJ, et al. Expert consensus on image-guided radiofrequency ablation of pulmonary tumors: 2018 edition. Thorac Cancer. 2018;9(9):1194–1208.