Abstract
Objectives
To evaluate acute changes in serum brain-derived neurotrophic factor (BDNF) concentration following combined endurance exercise and heat stress through head-out water immersion (HOI).
Setting
Observational study with crossover design.
Methods
Ten healthy young male participants performed HOI at 40 °C (40 °C HOI) or continuous cycling at 60% of maximal oxygen uptake while immersed in 40 °C (40 °C HOI-ex) or 23 °C water (23 °C HOI-ex) for 15 min. Serum BDNF, cortisol and lactate concentrations, and core temperature (Tcore) were measured pre, immediately post, and 15 and 30 min post-immersion.
Results
BDNF concentration increased immediately and 15 min after 40 °C HOI-ex, but not after 40 °C or 23 °C HOI-ex. No changes in Tcore concentration were observed during 23 °C HOI-ex (Pre; 37.3 °C ± 0.3 °C, Post; 37.8 °C ± 0.2 °C, Post 15; 37.4 °C ± 0.3 °C, Post 30; 37.2 °C ± 0.2 °C). Tcore increased significantly post, post 15, and post 30 min of 40 °C HOI (Pre; 37.1 °C ± 0.4 °C, Post; 38.8 °C ± 0.5 °C, Post 15; 37.9 °C ± 0.4 °C, Post 30; 37.9 °C ± 0.2 °C) and 40 °C HOI-ex (Pre; 37.2 °C ± 0.2 °C, Post; 40.2 °C ± 0.7 °C, Post 15; 38.9 °C ± 0.5 °C, Post 30; 38.3 °C ± 0.5 °C). Tcore was higher in 40 °C HOI-ex compared with 40 °C HOI and 23 °C HOI-ex immediately post and post 15 min. Plasma lactate and cortisol were significantly higher in 40 °C HOI-ex compared with 40 °C HOI and 23 °C HOI-ex after immersion (p = 0.001).
Conclusion
While 15 min HOI alone or thermoneutral exercise do not increase BDNF concentration, both combined may form a time-efficient strategy to acutely elevate BDNF.
Introduction
Regular exercise provides beneficial effects such as improvement in glucose and lipid metabolism [Citation1–4], brain health [Citation5–7], and prevention of neurologic disorders, including Alzheimer`s disease [Citation8], Parkinson disease [Citation9], and multiple sclerosis [Citation10]. One of the mechanisms by which exercise can improve glucose and lipid metabolism, as well as brain health, is by promoting the expression of neurotrophic factors, particularly brain-derived neurotrophic factor (BDNF) [Citation11,Citation12]. BDNF is a key protein that promotes neurogenesis, neuroprotection, neuro-regeneration, cell survival, development. and maintenance of synaptic connections between neurons, and plays a regulatory role in glucose and energy metabolism [Citation13–16]. Furthermore, it is suggested that elevating BDNF concentration, either pharmacologically or through endurance exercise, may aid in tissue repair and restoring the motor function in individuals with stroke or spinal cord injury [Citation17,Citation18].
Since Neeper et al. [Citation19] discovered that BDNF mRNA can be elevated through exercise, the effects of different exercise components on BDNF concentration, such as modality, intensity, and duration, have been investigated. In humans, regular resistance and endurance exercise elevates resting serum BDNF concentration [Citation20–24]. Moreover, a single bout of exercise for at least 30 min at an intensity higher than 60% of maximal oxygen uptake (VO2 max) can acutely elevate serum BDNF concentration [Citation10,Citation25]. However, notwithstanding the apparent existence of an exercise intensity and duration threshold to acutely elevate BDNF concentration, the influence of additional factors related to the exercise bout, such as the environment in which it is performed or the participant’s characteristics, remains unclear [Citation26,Citation27].
It has been suggested that environmental factors can increase serum BDNF concentration, independent of exercise. Goekint et al. [Citation28], for instance, reported that exercising in an ambient temperature of 30 °C increased serum BDNF levels to a larger extent compared with the same exercise in 18 °C. Furthermore, Kojima et al. [Citation29] recently reported that serum BDNF concentration can be increased by head-out water immersion (HOI) in water set at 42 °C for 20 min. Based on these results, the combined use of a thermal stressor and endurance exercise may elicit a synergistic effect. By combining both stressors, serum BDNF concentration may be elevated by shorter duration interventions than previously studied [Citation28,Citation29]. As a lack of time is often cited as a barrier for exercise participation [Citation30], such a time-efficient strategy would have significant practical implications for the promotion of health in the general population.
Therefore, the aim of this study was to evaluate the acute BDNF response to endurance exercise combined with HOI in water set at 40 °C. It was hypothesized that 15 min of endurance exercise combined with HOI results in a larger BDNF response compared with both stressors individually (i.e., endurance exercise or HOI only).
Materials and methods
Participants
Ten young healthy males (age, 23.7 ± 0.8 years; height, 170.1 ± 7.0 cm; weight, 63.6 ± 7.2 kg; body mass index, 21.7 ± 1.7 kg/m2; VO2 max, 2.8 ± 0.3 l/min) participated in this study. None of the participants had performed regular exercise for at least 6 months. Exclusion criteria were smoking and the use of medication for medical or psychiatric conditions.
The study was approved by the Research Ethics Committee of Wakayama Medical University and was performed in accordance with the provisions of the Declaration of Helsinki. The purposes and risks of the study were explained to all participants, and they provided written informed consent.
Experimental protocol
At least 1 week prior to the experiment, VO2 max was determined using a stepwise incremental cycle ergometry test (828E; Monark, Varberg, Sweden). O2 uptake and ventilation were measured with a respiratory metabolic cart (MetaMax 3B, Cortex, Leipzig, Germany).
For the experimental trials, participants visited the laboratory three times. In a randomized order, they performed seated rest in water set at 40 °C (40 °C HOI) or cycling ergometer continuous exercise at 60% VO2 max in 40 °C (40 °C HOI-ex). In addition, the same participants were invited for a third control visit, namely endurance exercise in thermoneutral water (23 °C HOI-ex). The visits were separated by a minimum of 1 week. All participants were instructed to refrain from intense exercise, alcohol, and caffeine consumption on the day prior to the experiment and to consume only water after 10:00 p.m.
On the day of the experiment, participants arrived at the laboratory between 9:00 a.m. and 12:00 noon, with the starting time kept the same for each participant. Participants changed into swimming trunks and rested in a thermoneutral room (28 °C) for a minimum of 30 min. Heart rate (HR) was monitored throughout using an electrocardiogram (BSM-2401; Nihon Kohden, Tokyo, Japan). Systolic (SBP) and diastolic blood pressure (DBP) were measured at the brachial artery using an inflatable cuff. Measurements were made every 5 min during the experimental period, and the mean value was used for each period: at baseline, immediately after completion of the immersion protocol, 15 min after completion of the immersion protocol, and 30 min after completion of the immersion protocol. Tcore was measured throughout using a copper-constantan thermocouple probe, which was inserted through the nasal cavity, with the tip of the probe at the atrial level of the esophagus. The Tcore was continuously measured from the beginning to the end of the experiment.
Participants urinated prior to the start of the experiment and then sat down outside of the immersion tank. They were permitted to drink water ad-libitum throughout the experiment. Body mass was measured before and after the experimental period, and water consumption during the experiment was checked. After 30 min of seated rest, participants entered the immersion tank for 40 °C HOI or 40 °C HOI-ex or 23 °C HOI-ex. They were immersed up to the neck in the immersion tank shown in . Water temperature was continuously monitored using digital and mercury thermometers. The water temperature was manually adjusted to 40 °C ± 0.5 °C or 23 °C ± 0.5 °C and circulated using an in-built pump. For 40 °C HOI-ex or 23 °C HOI-ex, participants were instructed to maintain a pedaling speed of 50 to 60 rpm, with adjustments made when needed to maintain an intensity of 60% VO2 max. During HOI-ex, recumbent cycling was performed whilst the participants were immersed in water and secured to the seat using a waist belt (). Resistance at the ergometer can be adjusted in steps of eight, using a magnet (AF-6500; ALINCO Inc., Osaka, Japan). The magnetic resistance was adjusted during the trial to maintain an intensity of 60% VO2 max, using a respiratory metabolic cart. After completion of the session, participants rested while seated in the laboratory for 30 min.
Venous blood samples were obtained from an antecubital vein at four time points: after the 30-min rest period (before the session, pre), immediately after the completion of the 15-min session (post), after 15 (post 15) and 30 (post 30) min of the completion of the 15-min immersion session. The blood samples (8 ml each) were used for the measurement of serum BDNF (2 ml), P-selectin (2 ml), plasma lactate (1 ml), and plasma cortisol concentration (1 ml), and a blood cell count (2 ml).
Blood sample analyses
The total blood cell count was calculated with a cell counter (XN-10; Sysmex Corporation, Kobe, Japan). Hemoglobin (Hb) concentration was measured using the sodium lauryl sulfate Hb method. Hematocrit (Hct) was measured by centrifugation. Blood samples were obtained from the antecubital vein for the measurement of various parameters using heparinized tubes and serum venipuncture tubes and ethylenediaminetetraacetic acid (EDTA)–2K– and EDTA–2Na–containing tubes. The tubes were spun immediately at 3500 rpm for 15 min at 4 °C (Model 5500; KUBOTA Corporation, Tokyo, Japan), and the serum and plasma were separated and stored at −80 °C until analysis.
To determine the plasma lactate concentration, 1 ml of blood was mixed with an equal volume of 0.8 N perchloric acid and centrifuged at 3000 rpm for 10 min. The supernatant was then assayed for lactate using a commercially available kit (Dainabot Lab, Tokyo, Japan) with the lactate oxidase–pyruvate oxidase method [Citation31].
Serum concentrations of BDNF and P-selectin were analyzed using enzyme-linked immunosorbent assay (R&D Systems, Minneapolis, MN, USA). The minimum detectable BDNF was < 20 pg/mL, and the intra- and inter-assay coefficients of variability (average CVs of different concentrations) were 5.0% and 9.0%, respectively. The post-session BDNF concentrations were adjusted for changes in plasma volume according to the method postulated by Dill and Costill [Citation32]. The minimum detectable P-selectin was < 0.121 ng/mL, and the average intra- and inter-assay CVs were 7.8% and 9.1%, respectively. The plasma cortisol levels were assayed using electrochemiluminescence immunoassay (Roche Diagnostics K.K., Tokyo, Japan). BDNF, P-selectin, plasma cortisol, and plasma lactate tests were performed in duplicate by investigators blinded to the study design.
Statistical analysis
Data normality was examined using the Shapiro–Wilk test. Data are expressed as mean ± SD, except when noted otherwise. Differences between 40 °C HOI, 40 °C HOI-ex, and 23 °C HOI-ex were examined using a 2-way repeated measures analysis of variance. When significance was detected, Bonferroni-corrected pairwise comparisons were made at each time point as a post-hoc test. The significance level for all analyses was set at p < 0.05. Effect sizes (Cohen's d) and 95% confidence intervals were also calculated for the BDNF responses to assist the interpretation of the comparisons [Citation33,Citation34]. All statistical analyses were performed using SPSS version 25.0 (IBM Corp., Tokyo, Japan). Based on Kojima et al. [Citation29], a sample size calculation using G*Power (version 3.1.9.6; Heinrich Heine Universitaet Duesseldorf, Düsseldorf, Germany) [Citation35,Citation36], with a power of 0.8 and significance set at 0.05, deemed 10 participant to be sufficient to detect an acute BDNF response.
Results
Serum brain-derived neurotrophic factor and core body temperature
The serum BDNF concentration significantly increased immediately after 40 °C HOI-ex (26.7 ± 2.2 to 34.1 ± 3.2 ng/mL; p = 0.003; 95% confidence interval [CI], −11.994 to −2.756; effect size [ES], 0.87) and remained significantly increased 15 min after the session (30.8 ± 3.1 ng/mL; p = 0.003; 95% CI, −6.689 to −1.427; ES, 0.87). Serum BDNF concentration 30 min post (28.9 ± 3.2 ng/mL) was not statistically different from that before the task (). No changes in serum BDNF concentration were observed during 40 °C HOI and 23 °C HOI-ex. Immediately post-session BDNF concentration was significantly higher in 40 °C HOI-ex compared with 40 °C HOI (p = 0.004) and 23 °C HOI-ex (p = 0.006).
Figure 2. Changes in serum BDNF concentration in response to the three experimental conditions. Data are presented as mean ± SD. *p < 0.01, compared with before immersion (pre). †p < 0.01, compared with 40 °C HOI and 23 °C HOI-ex. BDNF, brain-derived neurotrophic factor; 40 °C HOI, head-out water immersion at 40 °C; 40 °C HOI-ex, cycling ergometer exercise in combination with head-out water immersion at 40 °C; 23 °C HOI-ex, cycling ergometer exercise in combination with head-out water immersion at 23 °C; pre, 30-min rest period prior to immersion; post, immediately after the completion of 15-min immersion protocol; post 15, 15 min after the immersion protocol; post 30, 30 min after the completion of immersion protocol.
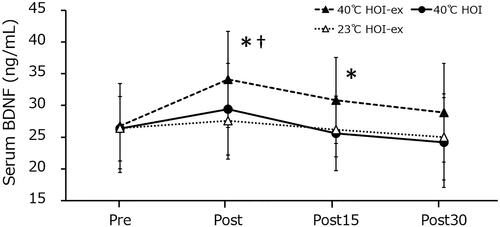
Tcore was significantly increased immediately after 40 °C HOI-ex (37.2 °C ± 0.1 °C to 40.2 °C ± 0.2 °C; p = 0.001) and remained elevated 15 and 30 min after the 40 °C HOI-ex task (38.9 °C ± 0.2 °C; p = 0.001; and 38.3 °C ± 0.1 °C; p = 0.002, respectively) (). Tcore was also significantly increased immediately after 40 °C HOI (37.1 °C ± 0.1 °C to 38.8 °C ± 0.1 °C; p = 0.001) and remained elevated 15 and 30 min after the session (37.9 °C ± 0.1 °C; p = 0.001; and 37.9 °C ± 0.1 °C; p = 0.002, respectively). No changes in Tcore concentration were observed during 23 °C HOI-ex. Tcore was significantly higher in 40 °C HOI-ex compared with 40 °C HOI and 23 °C HOI-ex immediately after and 15 min after the session (p = 0.001 and p = 0.001, respectively).
Figure 3. Changes in core temperature during the three experimental conditions. Data are presented as mean ± SD. *p < 0.01, compared with before immersion (pre). †p < 0.01, compared with 40 °C HOI and 23 °C HOI-ex. 40 °C HOI, head-out water immersion at 40 °C; 40 °C HOI-ex, cycling ergometer exercise in combination with head-out water immersion at 40 °C; 23 °C HOI-ex, cycling ergometer exercise in combination with head-out water immersion at 23 °C; pre, 30-min rest period prior to immersion; post, immediately after the completion of 15-min immersion protocol; post 15, 15 min after the immersion protocol; post 30, 30 min after the completion of immersion protocol.
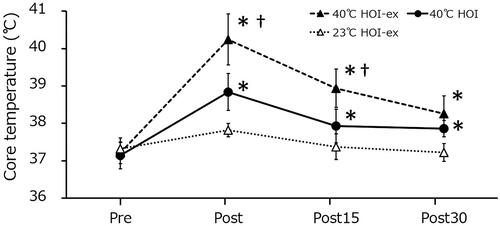
Heart rate, mean arterial pressure, hemoglobin, and hematocrit
HR was significantly increased immediately after 40 °C HOI-ex (p = 0.001) and remained elevated 15 and 30 min thereafter (p = 0.001 and p = 0.001, respectively) (). During 40 °C HOI, HR was significantly increased immediately after and remained elevated 15 and 30 min after the session (p = 0.001 and p = 0.046, respectively). HR was significantly increased immediately after 23 °C HOI-ex (p = 0.001) and remained elevated 15 and 30 min thereafter (p = 0.003 and p = 0.048, respectively). HR was significantly higher immediately after (p = 0.001), 15 min after (p = 0.001), and 30 min (p = 0.001) after 40 °C HOI-ex compared with 40 °C HOI and 23 °C HOI-ex (p = 0.001). SBP in 40 °C HOI, 40 °C HOI, and 23 °C HOI-ex remained unchanged throughout. DBP was significantly lowered immediately after (p = 0.001), 15 min after (p = 0.001), and 30 min after 40 °C HOI-ex (p = 0.001) compared with baseline. DBP in 40 °C HOI and 23 °C HOI-ex remained unchanged throughout. DBP was significantly lower in 40 °C HOI-ex compared with 40 °C HOI and 23 °C HOI-ex immediately after and 15 min after the session (p = 0.004 and p = 0.004, respectively). No changes in Hb or Hct were observed in 40 °C HOI, 40 °C HOI-ex, and 23 °C HOI-ex. There was no significant difference in body mass between before and after the intervention, nor in water consumed during the intervention, in either condition.
Table 1. The change in physiological parameters in response to the three experimental conditions.
Changes in platelet count and P-selectin level
The platelet counts in 40 °C HOI, 40 °C HOI-ex, and 23 °C HOI-ex remained unchanged throughout (). The P-selectin concentration was significantly increased immediately after 40 °C HOI-ex (p = 0.002) and remained elevated at 15 min post-session (p = 0.01) (). P-selectin concentration in 40 °C HOI and 23 °C HOI-ex remained unchanged throughout. The P-selectin concentration directly immediately after 40 °C HOI-ex was significantly higher than that in 40 °C HOI and 23 °C HOI-ex (p = 0.001).
Figure 4. Changes in platelet counts in response to the three experimental conditions. Data are presented as mean ± SD. 40 °C HOI, head-out water immersion at 40 °C; 40 °C HOI-ex, cycling ergometer exercise in combination with head-out water immersion at 40 °C; 23 °C HOI-ex, cycling ergometer exercise in combination with head-out water immersion at 23 °C; pre, 30-min rest period prior to immersion; post, immediately after the completion of 15-min immersion protocol; post 15, 15 min after the immersion protocol; post 30, 30 min after the completion of immersion protocol.
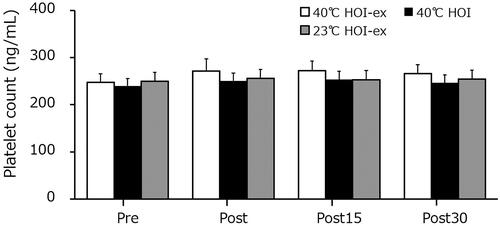
Figure 5. Changes in P-selectin concentration in response to the three experimental conditions. Data are presented as mean ± SD. *p < 0.01, compared with before immersion (pre). †p < 0.01, compared with 40 °C HOI and 23 °C HOI-ex. 40 °C HOI, head-out water immersion at 40 °C; 40 °C HOI-ex, cycling ergometer exercise in combination with head-out water immersion at 40 °C; 23 °C HOI-ex, cycling ergometer exercise in combination with head-out water immersion at 23 °C; pre, 30-min rest period prior to immersion; post, immediately after the completion of 15-min immersion protocol; post 15, 15 min after the immersion protocol; post 30, 30 min after the completion of immersion protocol.
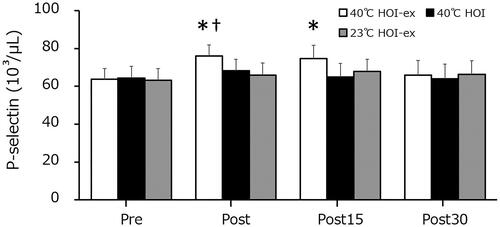
Changes in plasma cortisol and plasma lactate
Plasma cortisol concentration was significantly increased immediately after 40 °C HOI-ex and 40 °C HOI (p = 0.001 for both) and remained elevated 15 and 30 min post-session (p = 0.001 and p = 0.001, respectively) (). Plasma cortisol concentration was significantly increased immediately after 23 °C HOI-ex (p = 0.001) and remained elevated 15 min post-session (p = 0.001). Plasma cortisol concentration was higher in 40 °C HOI-ex compared with 40 °C HOI and 23 °C HOI-ex immediately after, 15 min after, and 30 min after the session (p = 0.001, p = 0.001, and p = 0.001, respectively). Plasma lactate concentration was significantly increased immediately after 40 °C HOI-ex (p = 0.001) and remained elevated 15 and 30 min thereafter (p = 0.001 and p = 0.001, respectively). The plasma lactate concentration significantly increased immediately after 23 °C HOI-ex and remained elevated 15 min after the session. No changes in plasma lactate concentration were observed during 40 °C HOI. Plasma lactate concentration was significantly higher in 40 °C HOI-ex compared with 40 °C HOI and 23 °C HOI-ex immediately after, 15 min after, and 30 min post-session (p = 0.001, p = 0.001, and p = 0.001, respectively).
Table 2. Change in plasma cortisol and plasma lactate concentration following the three experimental conditions.
Discussion
This study showed that 15 min of exercise in 40 °C water increases serum BDNF concentration, while the same duration 40 °C HOI alone or 23 °C HOI-ex do not elevate BDNF concentration. The BDNF response after 40 °C HOI-ex was accompanied by an increase in cortisol and lactate concentration.
In this study, both Tcore and serum BDNF concentration increased with 40 °C HOI-ex. Kojima et al. [Citation29] found that increasing Tcore to 39.5 °C ± 0.6 °C by a 20 min HOI in 42 °C water increases BDNF concentration, suggesting that heat stress can induce a BDNF response independently of exercise. However, while the passive 40 °C HOI condition increased the Tcore to 38.8 °C ± 0.1 °C, it did not increase serum BDNF concentration. Considering that a reduced heat exposure duration in the study of Kojima et al. [Citation29] could elicit an increased BDNF response, it may be that the time spent at an elevated Tcore was too short in our study to elevate BDNF concentration. In contrast, 40 °C HOI-ex increased Tcore to 40.2 °C ± 0.2 °C and did result in a significant BDNF response, suggesting that the exercise itself or the additional increase in Tcore may have contributed to the difference between the two conditions. In support for the use of combined exercise and heat stress, Van Cutsem et al. [Citation37] reported that Tcore increased to 38.7 °C and the serum BDNF concentration increased from 21.8 ± 1.3 to 26.5 ± 2.1 ng/mL after exercising on a cycling ergometer at 75% maximal power output for 30 min. Goekint et al. [Citation28] reported that after 60 min of cycling ergometer at 55% Wmax, the Tcore increased to 38.7 °C and the serum BDNF concentration also increased. Although BDNF concentration increased in these studies, the increase in Tcore was the same as that observed after 15-min HOI at 40 °C in our study (38.8 °C ± 0.1 °C). Possibly, BDNF concentration may have increased due to the additional effect of exercise even when the increase in Tcore was below the threshold required to increase serum BDNF concentration.
Despite the additive effects of exercise and heat stress, the increase in serum BDNF after 40 °C HOI-ex was smaller than in Kojima et al. [Citation29]. Specifically, in the study by Kojima et al., when HOI was performed at 42 °C for 20 min, serum BDNF increased ∼1.7 fold, whereas in the present study it increased ∼1.3 fold. As previously mentioned as a potential reason for the absent BDNF response after 40 °C HOI, session duration may have been a factor in this discrepancy. Indeed, the response in BDNF and other myokines have been reported to be largely dependent on intervention duration [Citation38–41]. In this study, after 15 min of 40 °C HOI-ex, Tcore was elevated to 40.2 °C ± 0.2 °C. In the experiment of Kojima et al., Tcore after 20 min of 40 °C HOI was 39.5 °C ± 0.6 °C. Peak Tcore is therefore unlikely to be the reason for the difference in BDNF response between both studies. On another note, exercise duration is also a likely explanation for the lack of a BDNF response following the 23 °C HOI-ex condition. Indeed, a minimum of 30 min at a moderate intensity has been suggested to elevate BDNF concentration [Citation42,Citation43].
The second reason is that the combined use of exercise and heat stress in this study resulted in a higher physiological stress compared with exercise at thermoneutral temperature. Cortisol is commonly known as a stress hormone [Citation44]. The increase in cortisol may have caused the downregulation of serum BDNF [Citation45,Citation46]. Exercise in this study was performed using 60% VO2 max, which is the suggested minimum exercise intensity required to increase serum BDNF concentration [Citation10,Citation25]. In addition, HR and lactate increased markedly during both 40 °C HOI-ex, suggesting that the physiological stress imposed by this condition was larger than land-based exercise at a similar intensity. The minimum HR that increases BDNF concentration after exercise may be around ≥70% of the maximal HR [Citation46]. The HR immediately after 40 °C HOI-ex was 90% of the maximal HR, which was sufficient to increase BDNF concentration. Furthermore, exercise-induced lactate and peripheral BDNF concentration have been reported to be correlated [Citation43,Citation47], which is supported by the results of this study. Previous studies have reported a negative correlation between cortisol and BDNF, with higher cortisol levels leading to lower BDNF concentrations [Citation44,Citation45]. In our study, high-intensity exercise was performed for 15 min continuously with 40 °C HOI-ex; thus, it was possible that cortisol increased, causing the serum BDNF levels to decrease.
With respect to the origin of BDNF, it has been reported that BDNF stored in platelets is released in response to exercise [Citation48–51]. In our study, there was no change in the platelet count immediately after and 15 min after the 40 °C HOI-ex. Nonetheless, the increased serum BDNF levels were likely to be derived from platelets, given that P-selectin, an indicator of platelet activity, was significantly increased.
Limitations and recommendations
Since this was the first study to investigate changes in BDNF during 40 °C HOI combined with endurance exercise, we decided to prescribe moderate-intensity exercise (i.e., 60% VO2 max). However, HR, lactate and cortisol concentration increased, resulting in an exercise session with a physiological stress akin to high-intensity exercise. Therefore, it is possible that the increase in serum BDNF concentration would have been lower with a lower-intensity exercise. On the other hand, Marquez et al. [Citation7] reported that BDNF concentration increases by ∼30% even when the total exercise time is only 20 min, using short high-intensity interval training (at 90% and 60% VO2 max, alternating every minute). In the present study, the increase in serum BDNF concentration after 15 min of exercise in hot water was similar to that reported by Marquez et al. [Citation7]. Thus, in keeping with high-intensity exercise, adding thermal stress to exercise may enhance the potency of exercise to induce an acute BDNF response. Considering the potential implications of this finding, we recommend future studies to further investigate the effects of session duration, temperature and intensity to optimize the new health interventions. In addition, we also recommend future studies with reduced exercise intensity and exploring additional exercise forms, such as high-intensity interval training. Moreover, it is necessary to adhere to the permitted exertion scores for the confirmation of the exercise intensity. It has also been reported that the serum BDNF response induced by exercise may vary depending on individual factors such as exercise habits [Citation46]. In addition, bathing habits may also affect perceived exercise intensity and physiological stress. Based on these points, a limitation of this study is that we did not collect participants’ bathing habits in the weeks prior to the study. Finally, given that the participants in this study were healthy young men, further studies are required in other populations, such as women, the elderly, and clinical populations.
Conclusion
The combination of 15-min HOI in 40 °C water with cycling ergometer exercise at 60% VO2 max acutely increases serum BDNF concentration, while 40 °C HOI and 23 °C HOI-ex do not. Therefore, the combination of exercise and heat stress may provide a time-efficient strategy to acutely elevate serum BDNF concentration. Further investigations, with multiple exercise protocols, temperatures, and time points; in addition to participants from a wide cross-section of the population in terms of health, age, and gender, are required to design widely-applicable novel interventions.
Acknowledgments
The authors thank the medical staff at Nachikatsuura Balneologic Town Hospital for their assistance, especially Dr. Izumi Yoshioka. We also thank Dr. Sven Hoekstra for the careful reading and editing of the manuscript.
Disclosure statement
No potential conflict of interest was reported by the author(s).
Additional information
Funding
References
- Pedersen BK. The anti-inflammatory effect of exercise: its role in diabetes and cardiovascular disease control. Essays Biochem. 2006; 42:105–117.
- Carroll S, Dudfield M. What is the relationship between exercise and metabolic abnormalities? A review of the metabolic syndrome. Sports Med. 2004; 34(6):371–418.
- Pedersen BK. The diseasome of physical inactivity-and the role of myokines in muscle-fat cross talk. J Physiol. 2009; 587(Pt 23):5559–5568.
- Tonoli C, Heyman E, Roelands B, et al. Effects of different types of acute and chronic (training) exercise on glycaemic control in type 1 diabetes mellitus: a meta-analysis. Sports Med. 2012; 42(12):1059–1080.
- Cotman CW, Berchtold NC. Exercise: a behavioral intervention to enhance brain health and plasticity. Trends Neurosci. 2002;25(6):295–301.
- Mattson MP. Energy intake and exercise as determinants of brain health and vulnerability to injury and disease. Cell Metab. 2012; 16(6):706–722.
- Marquez CMS, Vanaudenaerde B, Troosters T, et al. High-intensity interval training evokes larger serum BDNF levels compared with intense continuous exercise. J Appl Physiol (1985). 2015; 119(12):1363–1373.
- Buchman AS, Boyle PA, Yu L, et al. Total daily physical activity and the risk of AD and cognitive decline in older adults. Neurology. 2012; 78(17):1323–1329.
- Ahlskog JE. Does vigorous exercise have a neuroprotective effect in Parkinson disease? Neurology. 2011;77(3):288–294.
- Schulz K-H, Gold SM, Witte J, et al. Impact of aerobic training on immuno-endocrine parameters, neurotrophic factors, quality of life and coordinative function in multiple sclerosis. J Neurol Sci. 2004;225(1-2):11–18.
- Krabbe KS, Nielsen AR, Krogh-Madsen R, et al. Brain-derived neurotrophic factor (BDNF) and type 2 diabetes. Diabetologia. 2007;50(2):431–438.
- Oliff HS, Berchtold NC, Isackson P, et al. Exercise-induced regulation of brain-derived neurotrophic factor (BDNF) transcripts in the rat hippocampus. Brain Res Mol Brain Res. 1998;61(1-2):147–153.
- Wisse BE, Schwartz MW. The skinny on neurotrophins. Nat Neurosci. 2003;6(7):655–656.
- Pedersen BK, Pedersen M, Krabbe KS, et al. Role of exercise-induced brain-derived neurotrophic factor production in the regulation of energy homeostasis in mammals. Exp Physiol. 2009;94(12):1153–1160.
- Greenberg ME, Xu B, Lu B, et al. New insights in the biology of BDNF synthesis and release: implications in CNS function. J Neurosci. 2009;29(41):12764–12767.
- Park H, Poo MM. Neurotrophin regulation of neural circuit development and function. Nat Rev Neurosci. 2013; 14(1):7–23.
- Kim MW, Bang MS, Han TR, et al. Exercise Increased BDNF and trkB in the contralateral hemisphere of the ischemic rat brain. Brain Res. 2005;1052(1):16–21.
- Sasaki Y, Sasaki M, Kataoka-Sasaki Y, et al. Synergic effects of rehabilitation and intravenous infusion of mesenchymal stem cells after stroke in rats. Phys Ther. 2016;96(11):1791–1798.
- Neeper SA, Gómez-Pinilla F, Choi J, et al. Physical activity increases mRNA for brain-derived neurotrophic factor and nerve growth factor in rat brain. Brain Res. 1996;726(1-2):49–56.
- Levinger I, Goodman C, Matthews V, et al. BDNF, metabolic risk factors, and resistance training in middle-aged individuals. Med Sci Sports Exerc. 2008;40(3):535–541.
- Seifert T, Brassard P, Wissenberg M, et al. Endurance training enhances BDNF release from the human brain. Am J Physiol Regul Integr Comp Physiol. 2010;298(2):R372–7.
- Frazzitta G, Maestri R, Ghilardi MF, et al. Intensive rehabilitation increases BDNF serum levels in parkinsonian patients: a randomized study. Neurorehabil Neural Repair. 2014;28(2):163–168.
- Byun JE, Kang EB. The effects of senior brain health exercise program on basic physical fitness, cognitive function and BDNF of elderly women - a feasibility study. J Exerc Nutrition Biochem. 2016;20(2):8–18.
- Vedovelli K, Giacobbo BL, Corrêa MS, et al. Multimodal physical activity increases brain-derived neurotrophic factor levels and improves cognition in institutionalized older women. Geroscience. 2017;39(4):407–417.
- Castellano V, White LJ. Serum brain-derived neurotrophic factor response to aerobic exercise in multiple sclerosis. J Neurol Sci. 2008; 269(1-2):85–91.
- Hötting K, Schickert N, Kaiser J, et al. The effects of acute physical exercise on memory, peripheral BDNF, and cortisol in young adults. Neural Plast. 2016;2016:6860573.
- Santos CC, Diniz TA, Inoue DS, et al. Influence to high-intensity intermittent and moderate-intensity continuous exercise on indices of cardio-inflammatory health in men. J Exerc Rehabil. 2016;12(6):618–623.
- Goekint M, Roelands B, Heyman E, et al. Influence of citalopram and environmental temperature on exercise-induced changes in BDNF. Neurosci Lett. 2011;494(2):150–154.
- Kojima D, Nakamura T, Banno M, et al. Head-out immersion in hot water increases serum BDNF in healthy males. Int J Hyperthermia. 2018;34(6):834–839.
- Felipe FR, Aluísio JDB, Marlos RD, et al. The Role of Perceived Personal Barriers to Engagement in Leisure-Time Physical Activity. Am J Public Health. 2007;97(3):515–519.
- Asanuma K, Ariga T, Aida N, et al. A new method for simultaneous autoanalysis of plasma levels of lactic acids and pyruvic acids by means of the oxidase system. J Anal Biochem Sci. 1985; 8:16–24.
- Dill DB, Costill DL. Calculation of percentage changes in volumes of blood, plasma, and red cells in dehydration. J Appl Physiol. 1974;37(2):247–248..
- Grice JW, Barrett PT. A note on Cohen's overlapping proportions of normal distributions. Psychol Rep. 2014;115(3):741–747.
- Bosco FA, Aguinis H, Singh K, et al. Correlational effect size benchmarks. J Appl Psychol. 2015;100(2):431–449.
- Faul F, Erdfelder E, Lang A-G, et al. G*Power 3: a flexible statistical power analysis program for the social, behavioral, and biomedical sciences. Behav Res Methods. 2007;39(2):175–191.
- Faul F, Erdfelder E, Buchner A, et al. Statistical power analyses using G*Power 3.1: tests for correlation and regression analyses. Behav Res Methods. 2009;41(4):1149–1160.
- Van Cutsem J, Pattyn N, Vissenaeken D, et al. The influence of a mild thermal challenge and severe hypoxia on exercise performance and serum BDNF. Eur J Appl Physiol. 2015;115(10):2135–2148.
- Wahl P, Hein M, Achtzehn S, et al. Acute effects of superimposed electromyostimulation during cycling on myokines and markers of muscle damage. J Musculoskelet Neuronal Interact. 2015;15(1):53–59.
- Pedersen BK, Febbraio MA. Muscles, exercise and obesity: skeletal muscle as a secretory organ. Nat Rev Endocrinol. 2012;8(8):457–465.
- Walsh JJ, Tschakovsky ME. Exercise and circulating BDNF: Mechanisms of release and implications for the design of exercise interventions. Appl Physiol Nutr Metab. 2018;43(11):1095–1104.
- Gold SM, Schulz KH, Hartmann S, et al. Basal serum levels and reactivity of nerve growth factor and brain-derived neurotrophic factor to standardized acute exercise in multiple sclerosis and controls. J Neuroimmunol. 2003;138(1-2):99–105.
- Ferris LT, Williams JS, Shen CL. The effect of acute exercise on serum brain-derived neurotrophic factor levels and cognitive function. Med Sci Sports Exerc. 2007;39(4):728–734.
- Sapolsky RM, Romero LM, Munck AU. How do glucocorticoids influence stress responses? integrating permissive, suppressive, stimulatory, and preparative actions. Endocr Rev. 2000;21(1):55–89.
- Issa G, Wilson C, Terry AV, et al. An inverse relationship between cortisol and BDNF levels in schizophrenia: data from human postmortem and animal studies. Neurobiol Dis. 2010;39(3):327–333.
- Knaepen K, Goekint M, Heyman EM, et al. Neuroplasticity – exercise-induced response of peripheral brain derived neurotrophic factor: a systematic review of experimental studies in human subjects. Sports Med. 2010;40(9):765–801.
- Rothman SM, Mattson MP. Activity-dependent, stress-responsive BDNF signaling and the quest for optimal brain health and resilience throughout the lifespan. Neuroscience. 2013; 239:228–240.
- Sobral-Monteiro-Junior R, Maillot P, Gatica-Rojas V, et al. Is the “lactormone” a key-factor for exercise-related neuroplasticity? A hypothesis based on an alternative lactate neurobiological pathway. Med Hypotheses. 2019;123:63–66.
- Fujimura H, Altar CA, Chen R, et al. Brain-derived neurotrophic factor is stored in human platelets and released by agonist stimulation. Thromb Haemost. 2002;87(4):728–734.
- Tang SW, Chu E, Hui T, et al. Influence of exercise on serum brain-derived neurotrophic factor concentrations in healthy human subjects. Neurosci Lett. 2008;431(1):62–65.
- Matthews VB, Aström MB, Chan MH, et al. Brain-derived neurotrophic factor is produced by skeletal muscle cells in response to contraction and enhances fat oxidation via activation of AMP-activated protein kinase. Diabetologia. 2009;52(7):1409–1418.
- Heber S, Volf I. Effects of physical (in)activity on platelet function. Biomed Res Int. 2015;2015:1–11.