Abstract
Objective
To explore independent risk factors for incomplete radiofrequency ablation (iRFA) of colorectal cancer liver metastases (CRLM) and evaluate adverse outcomes following iRFA.
Materials and Methods
Magnetic resonance imaging data of CRLM patients who received percutaneous RFA were randomized into training (70%) and validation set 1 (30%) data sets. An independent validation set 2 was derived from computed tomography scans. Uni- and multivariate analyses identified independent risk factors for iRFA. Area under the curve (AUC) values were used to evaluate the predictive model performance. Risk points were assigned to independent predictors, and iRFA was predicted according to the total risk score. Kaplan–Meier curves were used to assess new intrahepatic metastases (NIHM), unablated tumor progression, and overall survival (OS).
Results
Multivariate regression determined as independent iRFA risk factors perivascular tumor location, subcapsular tumor location, tumor size ≥20 mm, and minimal ablative margin ≤5 mm. The AUC values of the model in the training set, validation set 1, and validation set 2 were 0.867, 0.772, and 0.820, respectively. The respective AUC values of the total risk score were 0.864, 0.768, and 0.817. During the 6-year follow-up, the cumulative OS was significantly shorter in the iRFA than in the complete RFA group, and NIHM (hazard ratio [HR] = 2.79; 95% confidence interval [CI]: 1.725, 4.513) and unablated tumor progression (HR = 3.473; 95% CI: 1.506, 8.007) were more severe.
Conclusions
Perivascular tumor location, subcapsular tumor location, tumor size ≥20 mm, and minimal ablative margin ≤5 mm were independent risk factors for iRFA. iRFA may be a potential predictor of NIHM, unablated tumor progression, and OS.
Introduction
Colorectal cancer is one of the most common malignant tumors in the world and the second leading cause of cancer-related death in the United States [Citation1]. The liver is the main organ affected by hematogenous metastasis of colorectal cancer, and over 25% of patients with colorectal cancer have hepatic metastases during their disease course [Citation2–4]. The preferred treatment for colorectal cancer liver metastases (CRLM) is surgical resection, but the resection rate of CRLM varies greatly among hospitals, and the overall resection rate is less than 30% [Citation5–7]. For CRLM not suitable for resection, radiofrequency ablation (RFA) plays a crucial role in eliminating tumor cells, reducing tumor burden, stimulating the host’s anti-tumor immune response [Citation8], controlling disease progression, and prolonging overall survival (OS). With the advantages of being minimally invasive and reproducible, RFA rarely leads to a sudden reduction in liver reserve function and can be performed in some patients with comorbidities, which expands the number of patients eligible for this treatment. The results of a phase-II randomized clinical trial proved that, compared with a systemic treatment alone, active implementation of local RFA combined with systemic treatment can effectively improve the local tumor progression-free survival and mid- to long-term OS rates of patients with unresectable CRLM [Citation9].
However, the temperature distribution range and treatment effect of RFA are affected by multiple factors such as the internal tissue composition, tumor size and location, peripheral blood vessels (portal vein, hepatic vein) and organs (gallbladder, small intestine), and guidance equipment [Citation10–12]. For the treatment of some refractory CRLM, sufficient energy output within the acceptable range may also not always achieve a satisfactory ablative margin and tumor eradication. With the occurrence of incomplete RFA (iRFA), micro-metastases in the hypoxic transition zone around the lesion have acquired the potential to develop into highly proliferating and aggressive cancer phenotypes, which to a certain extent can rapidly accelerate tumor metastasis and proliferation processes, leading to shorter local tumor progression-free survival [Citation13–17]. The oncological outcomes of RFA treatment in CRLM, including the evaluation and prediction of OS and local tumor progression-free survival, were based on dynamic contrast-enhanced (DCE) computed tomography (CT) findings in several studies. Shady et al. [Citation18] reported an improved (in comparison to surgical literature) clinical risk score for ablation, whereas Han et al. [Citation6] completed a 15-year long-term follow-up and proposed a prediction algorithm for local tumor progression-free survival based on a multivariate Cox regression model.
In the present study, based on DCE magnetic resonance imaging (MRI) and relevant periprocedural clinical data, we used univariate and multivariate analyses to identify the independent risk factors for iRFA. Based on the identified risk factors, we developed and verified an algorithm for predicting iRFA. Hence, for the first time, the progression during follow-up has been separately analyzed and evaluated for unablated CRLM due to limited access windows and new intrahepatic metastases (NIHM) [Citation19].
Materials and methods
Study population
The study was conducted in accordance with the World Medical Association’s Declaration of Helsinki guidelines and approved by the Ethics Committee of Sir Run Run Shaw Hospital affiliated to Zhejiang University School of Medicine. The requirement of written informed consent was waived owing to the retrospective nature of this study. The study population included patients with CRLM who received RFA treatment between January 2012 and January 2017. In this retrospective study, the exclusion criteria for RFA treatment were [Citation1] uncorrectable coagulopathy [Citation2]; Child–Pugh grade C liver function [Citation3]; disturbance of consciousness [Citation4]; uncorrectable massive ascites [Citation5]; metastases greater than 50 mm or the number of metastases greater than 5 [Citation6]; failure of vital organs such as the heart, kidney, and liver; and [Citation7] biliary tract infections or sepsis. During statistical data processing, patients were excluded because of [Citation1] incomplete clinical or imaging data, such as missing periprocedural MR images and surgical records [Citation2]; additional RFA retreatment of the tumor; and [Citation3] follow-up period <4 months. The inclusion criteria were as follows [Citation1]: the number of lesions ≤5 [Citation2], the maximum size of tumor <50 mm [Citation3], RFA treatment for CLMs for the first time [Citation4], complete clinical and imaging data [Citation5], the primary tumor has been treated surgically. For some patients with multiple metastatic tumors that were not suitable for one-time RFA removal and had received the planned first- and second-phase RFA treatment within 4 weeks, these two RFA procedures were considered as one treatment episode.
Preprocedural examination
The MR examinations (GE Signa HDx 3.0 T, Boston, MA, USA) of the liver or upper abdomen were performed within 1 week before RFA and included nonenhanced and DCE T1-weighted imaging, T2-weighted imaging, and diffusion-weighted imaging (DWI) to determine the number, size, and location of the tumor, as well as adjacent blood vessels, tissues, and organs. The MR scan parameters are shown in Supplementary Table S1. The contrast agent gadopentetic acid dimeglumine solution (20 ml: 9.38 g; Beilu Pharmaceutical Co., Ltd., Beijing, China) was used for DCE MR examination with an injection speed of 3 ml/s. Then, 20 ml of normal saline was used for flushing, and images of the arterial phase, portal phase, and equilibrium phase were obtained by scanning at intervals of 30, 60, and 90 s, respectively. The acquisition parameters of DWI were as follows: field of view, 400 × 400; matrix, 320 × 224; section thickness, 6; B value, 0/800. The collected DWI images were post-processed (b800-b0), and the apparent diffusion coefficient (ADC) map was calculated and generated using the Picture Archiving and Communication Systems, and then, the ADC value was obtained by delineating the area of interest of the lesion. Laboratory tests, such as coagulation function, liver function, heart function, blood routine, renal function, tumor markers (carbohydrate antigen [CA]-125, CA19-9, carcinoembryonic antigen [CEA]), and electrolyte levels, were performed to identify possible contraindications.
RFA procedure
The puncture level and needle entry point on the body surface were determined using CT (Siemens Definition AS 20, Erlangen, Germany), and the patient was required to adopt the appropriate position (supine or lateral position) according to tumor localization and needle insertion point and angle. RFA treatment was performed under CT guidance after local disinfection of the puncture area and administering percutaneous infiltration anesthesia of 5–10 ml 2% lidocaine (North China Pharmaceutical Co. Ltd., Hebei, China). Hydromorphone hydrochloride (Renfu Pharmaceutical Co. Ltd., Hebei, China) was used for sedation and as an analgesic. An RF-3000 radiofrequency generator (Boston Scientific Corp., Boston, USA) was used for thermal CRLM ablation following a percutaneous intrahepatic puncture. According to tumor size, a single needle or needle cluster and a radiofrequency electrode were appropriately selected, and multiple CT scans were obtained during the puncture process to ensure the accuracy of the needle direction and insertion depth. After the front end of the electrode reached the edge of the lesion, the sub-electrodes were deployed according to the size of the lesion, and CT was performed again to ensure that the sub-electrode covered the entire lesion. The intended ablative margin of the RFA procedure was 5–10 mm. To prevent the occurrence of bleeding or tumor spreading during the treatment course, cauterization of the electrode path was performed when the needle was withdrawn. After reaching the target endpoint, whole-liver DCE CT was immediately performed to assess the coverage rate and possible complications. A tumor that had been ablated according to the treatment plan and was completely covered by the ablation zone was defined as a technical success.
Follow-up
All patients received DCE MR examination consistent with the preoperative scan plan within 4–8 weeks after RFA as a baseline scan for future comparison. Based on DCE MRI, any changes in morphology, T2-weighted imaging, ADC, and perfusion parameters of the treated CRLM sites and their periphery were observed and recorded to determine tumor recurrence. Unablated tumors due to limited access windows were treated with other comprehensive methods to achieve tumor control, and the post-RFA progression was evaluated. The time of the last contact with the doctor or the last hospitalization review was considered as censored data independent of whether the patient was lost to follow-up, had died of reasons unrelated to CRLM, or was still alive at the end of the follow-up period. In this study, iRFA, local tumor progression-free survival (NIHM or recurrence), and OS (death from any cause) were set as the primary outcome measures, and complication was set as the secondary outcome measure. During follow-up, NIHM and incompletely treated CRLM were considered for additional RFA courses based on tumor size and location.
Definitions
Based on multiple previous studies [Citation6,Citation13,Citation18,Citation19] of MR examinations within 4 months post-RFA, we defined iRFA as [Citation1] the presence of active lesions in the original ablation zone [Citation2], the presence of new active lesions within 1 cm of the ablation zone, and [Citation3] expansion of the initial ablation zone. When these abnormal imaging changes were not found within 4 months post-RFA, the procedure was considered as complete RFA (cRFA). CRLM were defined as perivascular tumors when they had any contact with first- or second-order branches of the portal or hepatic vein with a diameter ≥5 mm. Based on MR images, crucial structures or organs, such as the diaphragm, gallbladder, stomach, intestine, and kidneys, that may affect the entire RFA procedure were recorded as potential influencing factors. CRLM with a distance of < 5 mm from the nearest hepatic capsule were defined as subcapsular tumors [Citation6]. The distance from the deepest CRLM point to the nearest hepatic capsule was defined as the tumor depth [Citation20].
According to previously reported methods to determine the minimal ablative margin [Citation6,Citation18], this study measured the distance from the tumor edge to selected surrounding anatomical landmarks (such as the hepatic capsule, second-degree branches of the portal or hepatic vein) using pre-RFA MR images, and the distance from the ablative margin to the same landmarks was measured using post-RFA MR images. The minimum of the distance differences between pre- and post-RFA images was defined as the minimal ablative margin ().
Figure 1. The axial (A,C) and coronal (B,D) images showing the measurement process of the minimal ablative margin. The distances (L1–L7) are measured from the edge of the tumor to the selected anatomical landmarks in the pre-RFA magnetic resonance (MR) images, and then the corresponding distances (L1′–L7′) are measured from the ablation edge to the same landmarks in the post-RFA MR images. The differences between the corresponding distances are determined, and the smallest value among the seven differences is selected as the minimal ablative margin. RFA: radiofrequency ablation.
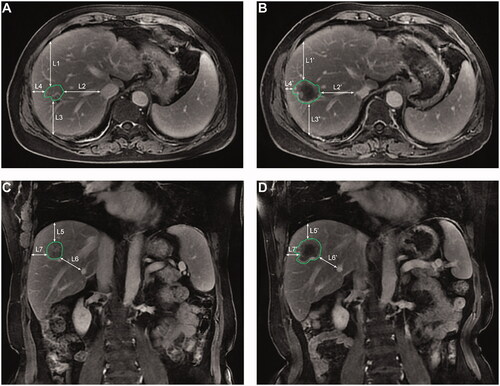
All the above-mentioned measurements of CRLM-related parameters were collected by two radiologists with experiences of more than 10 years of hepatobiliary DCE T1-weighted MRI with axial and coronal acquisitions. According to the definition of complications in the clinical practice guidelines of the Society of Interventional Radiology (SIR), events that caused severe morbidity or disability, increased the level of care, or substantially lengthened the hospital stay were defined as major complications [Citation19]. All other events were considered minor complications.
Predictive model
The process of developing and verifying a reliable iRFA prediction model included three steps [Citation1]. Appropriate continuous or categorical variables were selected as clinically possible predictors for iRFA to reduce possible deviations in regression coefficients by considering clinical application, measurement costs, literature reports, expert knowledge, and predictive model fit [Citation2,Citation6,Citation19,Citation21,Citation22]. The iRFA prediction model was developed and verified using independent datasets. (3) The risk points were assigned to predictor variables, and the total risk score of a tumor was calculated to predict iRFA.
The data were randomly divided into training and internal validation (validation set 1) datasets at a ratio of 7:3 based on the transparent reporting of multivariate predictive models [Citation22]. If independent variables that can only be measured on MR images (ADC, T2-weighted signal intensity, enhancement degree) were not included in the prediction model, the effective predictor variables of the model could also be accurately measured on the three-dimensional reconstruction image of the DCE CT portal phase using the same method as above. Therefore, an independent dataset (validation set 2) based on CT images was prepared as an alternative to assess the broadness of the model’s applicability.
Statistical analysis
SPSS 24.0 (IBM, Chicago, USA), R 4.0.2 (R Foundation for Statistical Computing, Vienna, Austria), and GraphPad Prism 7.0 (Graph Pad Software, San Diego, USA) were used for statistical analyses. Continuous measurement data that met the criteria of normal distribution and homogeneity of variance tests are presented as the mean ± standard deviation, whereas data with skewed distribution or uneven variance are presented as the median and quartiles. Categorical data are presented as n (%). The characteristics of patients in different groups of datasets were compared using Student’s t-test, one-way ANOVA, the post hoc least significant difference test, and the Student–Newman–Keuls test for continuous variables with normal distribution and the χ2 test for categorical variables. The rank-sum test was used for data with uneven variance or skewed distribution.
According to the data distribution characteristics in the training set, the above univariate analysis method was used to select variables. To prevent the loss of crucial predictors, variables with univariate analysis results of p < 0.15 were analyzed using the forward maximum likelihood method (forward: LR) for multivariate logistic regression (MLR). The area under the curve (AUC) of the receiver operating characteristics (ROC) analysis was used to verify the performance of the predictive model in the training set, validation set 1, and validation set 2.
The β regression coefficient of the predictor variable included in the MLR model was multiplied by 2, and the nearest integer was assigned as the weighted score to each tumor [Citation6,Citation23]. The total risk score of each tumor in the training and validation sets was calculated and used to assess the risk of iRFA occurrence.
The Kaplan–Meier method and log-rank test were used to evaluate NIHM, the progression of unablated tumors, and OS. The cumulative hazard function was used to estimate the relative risk of NIHM, unablated tumor progression, and patient death per unit of time during the follow-up period. A two-sided p-value <0.05 was considered statistically significant.
Results
Patient information
This study included 152 consecutive patients, aged 27–89 (mean, 60.22 ± 11.38) years, based on their MRI findings. Among 408 CRLM in these patients, 316 CRLM were successfully ablated, and they were randomly divided into a training set of 220 CRLM (103 patients) and validation set 1 of 96 CRLM (49 patients). Ninety-two tumors in 57 patients were not ablated, and 31 of these tumors in 14 patients were surgically removed during the follow-up period. Therefore, 61 CRLM in 43 patients were not cured owing to various reasons, such as limited location accessibility, large tumor size, and lack of patient willingness. These patients were treated with comprehensive post-RFA strategies such as stereotactic body radiotherapy, chemotherapy, targeted therapy, and transcatheter arterial chemoembolization to control tumor progression. The independent data validation set 2 was derived from DCE CT images of 117 CRLM in 57 patients. The differences among baseline data of the three datasets were not significant (; all p > 0.05).
Table 1. Baseline characteristics of the development and validation datasets.
Development and verification of the predictive model
Of the tumors used for model training, 165 CRLM (75%) achieved cRFA. The information on tumors of the training set is summarized in . Univariate analysis revealed differences between the iRFA and cRFA groups in the following parameters: perivascular location, liver segment, subcapsular location, size (≥20 mm), and minimal ablative margin (≤5 mm). In the multivariate analysis, only perivascular tumor location, tumor size (≥20 mm), subcapsular location, and minimal ablative margin ≤5 mm were identified as independent predictive variables for iRFA. The analysis of tolerance and variance inflation factors ruled out possible collinearity problems. Omnibus tests of model coefficients confirmed the statistical significance of the predictive model (χ2 = 85.174, p < 0.001); the Hosmer–Lemeshow test confirmed the goodness of fit of the logistic regression model (χ2 = 0.499, p = 0.974). The ROC curve of the training model is shown in , with an AUC of 0.867 and a Nagelkerke R2 of 0.454, indicating that the model had a good predictive performance for iRFA in the training set.
Figure 2. (A,C) Receiver operating characteristic (ROC) curves for the multivariate logistic regression model in the training set, validation set 1, and validation set 2. (B, D) ROC curves for the total risk score in the training set, validation set 1, and validation set 2. The area under the curve (AUC) shown in each figure summarizes the performance of the overall prediction model, with 0.5 indicating that the model has no predictive value and 1.0 indicating a perfect prediction performance. The data in parentheses represent 95% confidence intervals.
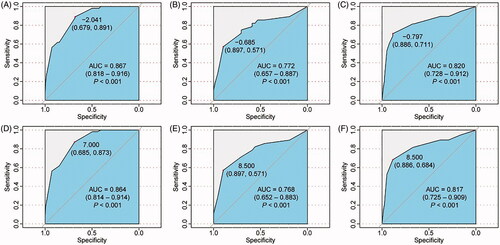
Table 2. Multivariate logistic regression analysis of predictive factors for iRFA in the training dataset.
During the development of the model, no significant differences in the comparison of the independent MRI-specific variables were found among the study groups, which means that the predictive variables included in the model are all derived from anatomical information, such as tumor size and minimal ablative margin, and can, therefore, be accurately obtained from CT images. Therefore, we verified the model using CT images of validation set 2. The performances of the prediction model in validation set 1 and 2 are shown in with AUC values of 0.772 and 0.820, respectively. Considering that the predictor variable minimal ablative margin <5 mm had the largest contribution to the model, another model was developed that used this parameter as its only predictor variable. Its AUC values in the training set, validation set 1, and validation set 2 were 0.733, 0.669, and 0.630, respectively (Supplementary Figure S1A–S1C).
Total risk score for iRFA
The predictor variable scores were assigned as follows: 3 points for perivascular tumors, 2 points for subcapsular tumors, 3 points for tumor size ≥20 mm, and 6 points for minimal ablative margin ≤5 mm. The occurrence of iRFA of the corresponding CRLM was evaluated based on the total risk score, which is shown in . When the total risk score was used to predict iRFA, the AUC values of the ROC curve for the training set, validation set 1, and validation set 2 were 0.864, 0.768, and 0.817, respectively, with a good predictive performance for iRFA ().
Major complications
According to the SIR Classification System for Complications, the incidence of treatment-related major complications was 7.9%, including bleeding or oozing (5 cases), pleural effusion (2 cases), pneumothorax (2 cases), skin burns (1 case), abdominal effusion (1 case), and abdominal wall edema (1 case). Pleural effusion was drained using a closed thoracic cavity, negative pressure drainage was used for abdominal effusion, and chest tube placement was adopted for pneumothorax. The other major complications improved after symptomatic treatment.
Follow-up findings
During the 6-year follow-up period, 42 patients (27.6%) died, and the cumulative OS rates at 1, 3, and 5 years were 95.0, 66.7, and 41.7%, respectively, with a median OS of 55 months (). Compared with those of the cRFA group, the cumulative OS rates of the iRFA group at 1, 3, and 5 years were significantly lower (χ2 = 21.27, p < 0.001; ).
Figure 4. Kaplan–Meier curves for overall survival (OS), new intrahepatic metastases, and unablated tumor progression in the entire magnetic resonance imaging data set (A,C,E). Kaplan–Meier curves and the log-rank (Mantel–Cox) test comparing OS, new intrahepatic metastases, and unablated tumor progression between complete radiofrequency ablation (cRFA) and incomplete radiofrequency ablation (iRFA) groups (B, D, F). p < 0.05 means that the difference is significant.
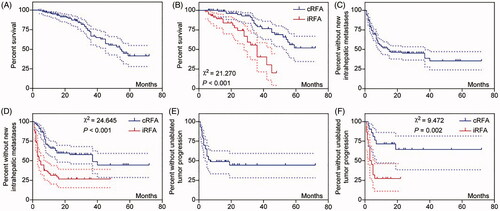
During the follow-up period, 80 patients (52.6%) developed NIHM, with a median NIHM-free time of 15 months, and the rates of NIHM-free patients at 1, 3, and 5 years were 52.027, 45.255, and 35.534%, respectively (). NIHM were more likely to occur in the iRFA group than in the cRFA group (43/62, 69.4% vs. 37/90, 41.1%; χ2 = 11.746, p = 0.001); further, the median NIHM-free time was also significantly shorter in the iRFA group than in the cRFA group (χ2 = 24.645, p < 0.001; ). Repeated RFA was performed in 62 NIHM patients, with a median time of 5 months. The remaining 18 patients did not undergo repeated RFA owing to excessive tumor size, excessive tumor number, poor accessibility, or rejection of RFA. These patients underwent hepatectomy (1 case), radioactive-seed implantation (2 cases), transcatheter arterial chemoembolization (2 cases), and other combined treatment strategies (such as stereotactic body radiotherapy, or chemotherapy) for tumor control.
Of the 43 patients with unablated tumors, 23 (53.5%) showed progression after combined therapy, and 21 of these progression cases (91.3%) occurred within 7 months after RFA (). Compared with the cRFA group (7/21, 33.3%), the iRFA group had more cases (16/22, 72.7%; χ2 = 6.702, p = 0.01) and more rapid progression of unablated CRLM progression (χ2 = 9.472, p = 0.002; ). The hazard ratios for iRFA to cRFA were 2.79 (95% confidence interval [CI]: 1.725, 4.513) for NIHM, 3.473 (95% CI: 1.506, 8.007) for unablated tumor progression, and 3.555 (95% CI: 1.712, 7.381) for death (Supplementary Figure S1D–S1F).
Discussion
To the best of our knowledge, only a few clinical studies reported on iRFA of liver metastases in colorectal cancer [Citation13], and no research on risk factors for iRFA of CRLM has been published yet. In the present study, the results of the univariate analysis suggested that iRFA might be associated with hepatic segments, but this parameter was not selected for the final predictive model. Based on the MLR analysis, independent predictors for iRFA were CRLM contacting first- or second-order branches of the portal or hepatic vein with sizes ≥5 mm, tumor size ≥20 mm, subcapsular location, and a minimal ablative margin ≤5 mm. With satisfactory goodness of fit and prediction performance, this predictive model can be used as an aid in clinical decision-making to select patients most likely to benefit from treatment. By assigning points to each predictive variable, the iRFA risk can be intuitively quantified, preliminarily estimated, and predicted based on periprocedural MRI features. The total risk score indicated good performance in the prediction of iRFA, with AUC values similar to those of the MLR model. The iRFA incidence was >50% for CRLM, with total risk scores indicating a high risk. During the follow-up period, we found for the first time that iRFA may lead more frequently to the rapid occurrence of new metastatic tumors in the liver, affecting the comprehensive treatment effect of local unablated tumors and thus adversely affecting the patient’s OS. Therefore, complete tumor ablation by primary RFA, essential for tumor control and survival of the affected patients, should be the treatment target in CRLM.
Sufficient ablative margins are the key determinants of RFA to achieve local tumor control [Citation24,Citation25]. Similar to the width of the tumor resection margin in hepatectomy being correlated with the prolonged OS, there is a correlation between the ablative margin in RFA and the viability of remaining tumor cells [Citation24–26]. However, it is already difficult to obtain accurate histopathological results following liver resection, let alone precise findings based on only MR or CT images, given tissue motion artifacts, partial volume effects, and insufficient resolution due to similar signal intensity/density. In this study, minimal ablative margins ≤ 5 mm were assigned the highest risk points as the most critical risk factor for iRFA. However, when used alone to predict iRFA, the performance of this parameter alone was not as good as in the MLR and risk score models, indicating the additional contribution of other variables to iRFA.
Tumor size is considered to be a risk factor for local tumor progression because a tumor with a larger diameter will exceed the ablation zone targeted by the RFA probe and lead to iRFA [Citation6,Citation18]. In view of the impact of tumor location and surrounding key anatomical structures on iRFA, it was finally found that subcapsular and perivascular tumor locations were independent risk factors. The treatment of subcapsular tumors may be technically difficult. Sufficient ablative margins cannot be obtained close to the liver capsule, and RFA may even cause heat damage to adjacent structures such as the diaphragm and gastrointestinal tract, bleeding, or extrahepatic tumor spreading [Citation11,Citation27,Citation28]. Therefore, a subcapsular tumor location may be another factor affecting the efficacy of tumor treatment [Citation6,Citation11,Citation27,Citation28]. However, the efficacy of RFA in the treatment of subcapsular tumors remains controversial. Some studies suggest that tumors in high-risk locations do not respond differently to therapeutic interventions when compared with tumors in other locations. By applying appropriate image-guided and assistive technologies, avoiding damage to the tumor capsule exposed on the peritoneal surface, and selecting the appropriate needle insertion site and patient position, cRFA can be achieved to a large extent to improve the patient’s prognosis and reduce complications, such as tumor seeding and bleeding caused by percutaneous RFA [Citation29–32]. In view of the small sample sizes of these studies, frequently from a single treatment center, the controversy about the impact of the tumor location on treatment effects still needs to be addressed by large, prospective, and long-term follow-up studies, especially for subcapsular tumor locations.
Unlike in hepatocellular carcinoma, dilatation of the intrahepatic bile duct was less common in CRLM patients of this study. Therefore, we did not include this parameter as an independent variable in the statistical analysis. The retrospective analysis by Kang et al. [Citation33] concluded that the long-term treatment results by RFA were similar for hepatocellular carcinoma near small blood vessels (i.e. with exposure to hepatic blood vessels with a diameter ≥3 mm) and for nonperivascular hepatocellular carcinoma. Similar results for RFA treatment of CLMs were also reported in the study of Jiang et al. [Citation34]. Since the heat sink effect of the perivascular tissue is generally present when the vein has a diameter >5 mm [Citation35] and to obtain more meaningful variables, we defined a perivascular CRLM as a tumor contacting first- or second-order branches of the portal or hepatic vein with a diameter ≥5 mm. For perivascular CRLM, the heat sink effect affects the temperature distribution during the treatment procedure, limiting the degree of tissue damage caused by RFA [Citation12,Citation19]. The protection of blood vessels to prevent massive bleeding during the treatment procedure is also one of the causes of tumor iRFA that cannot be ignored. Sublethal high temperatures will affect the microenvironment of residual tumor cells, potentially leading to high invasiveness and rapid local progression [Citation17].
Tissue-specific characteristics such as tissue elasticity, fibrosis, ratio of cystic and solid lesions, tissue water content, and blood supply status may also affect the realization of cRFA [Citation12,Citation19]. Therefore, our study also analyzed T2-weighted signals, ADC values, and enhancement on DCE MR images of the tumors. However, no significant differences were found in this process. Given that the anatomical features of a tumor can also be accurately obtained from CT images, we opted for the subsequent verification of the predictive model data obtained using this imaging modality and achieved satisfactory prediction performances. This suggests that it is not important whether the model parameters are determined using CT or MR images; CT- and MRI-derived variables are similar non-negligible risk factors for iRFA, which is of clinical value for the assessment of the perioperative tumor treatment.
During the follow-up process, RFA retreatment of new metastases or progressive tumors in the liver is considered effective and has been clinically recommended [Citation18]. The patient’s long-term OS should not only be attributed to RFA; it is also affected by many other factors, including lymph node status, TNM staging of the primary tumor, CEA level, the number of tumors, extrahepatic metastasis, and physical fitness [Citation18,Citation36]. However, these variables do not seem to have a significant impact on iRFA in this study, which may be owing to the short 4-month time frame used in the definition of iRFA. The presence of residual or recurrent tumors and the patient’s OS cannot be completely reflected by iRFA. In this study, patients in the iRFA group seemed to be more likely to develop NIHM and unablated tumor progression, which means that the presence of residual tumors adversely affects the comprehensive treatment of intrahepatic tumors. This adverse effect may even involve the expression of genes involved in local inflammation, immunosuppression, autophagy, metastasis, and proliferation of tumor cells [Citation13,Citation16,Citation37,Citation38].
This study has several limitations. First, this study was a retrospective analysis with the possibility of selection bias. In addition, follow-up results were limited by subject compliance, disease severity, and medical treatment conditions, and the existence of patients who were lost to follow-up may have affected the statistical results, especially the observation of NIHM and unablated tumor progression. Second, owing to the small sample size and lack of strong external validation, it is uncertain whether the developed model is applicable to patients in other regions. Third, this study did not include some important factors that may have an impact on tumor recurrence and patient survival, such as neutrophil-to-lymphocyte ratio, general anesthesia, mutant KRAS, and RAS [Citation39–42]. Finally, changes in treatment strategies may be an important confounding variable that affects the study results, and it is uncertain whether this confounding variable is evenly distributed in the cRFA and iRFA groups. These limitations should also be considered in future studies.
In summary, iRFA of CRLM may aggravate NIHM and local unablated tumor progression. Perivascular and subcapsular tumor locations, a minimal ablative margin ≤5 mm, and a tumor size ≥20 mm are independent risk factors for iRFA. The MLR and total risk score models based on these variables can predict the likelihood of iRFA and assist in clinical decision-making processes.
Supplemental Material
Download PDF (325.4 KB)Disclosure statement
No potential conflict of interest was reported by the author(s).
Additional information
Funding
References
- Siegel RL, Miller KD, Goding Sauer A, et al. Colorectal cancer statistics, 2020. CA A Cancer J Clin. 2020;70(3):145–164.
- Manfredi S, Lepage C, Hatem C, et al. Epidemiology and management of liver metastases from colorectal cancer. Ann Surg. 2006;244(2):254–259.
- Ruers T, Bleichrodt RP. Treatment of liver metastases, an update on the possibilities and results. Eur J Cancer. 2002;38(7):1023–1033.
- Engstrand J, Nilsson H, Stromberg C, et al. Colorectal cancer liver metastases - a population-based study on incidence, management and survival. BMC Cancer. 2018;18(1):78.
- Hackl C, Neumann P, Gerken M, et al. Treatment of colorectal liver metastases in Germany: a ten-year population-based analysis of 5772 cases of primary colorectal adenocarcinoma. BMC Cancer. 2014;14:810.
- Han K, Kim JH, Yang SG, et al. A single-center retrospective analysis of periprocedural variables affecting local tumor progression after radiofrequency ablation of colorectal cancer liver metastases. Radiology. 2021;298(1):212–218.
- Fenton HM, Taylor JC, Lodge JPA, et al. Variation in the use of resection for colorectal cancer liver metastases. Ann Surg. 2019;270(5):892–898.
- Mizukoshi E, Yamashita T, Arai K, et al. Enhancement of tumor-associated antigen-specific T cell responses by radiofrequency ablation of hepatocellular carcinoma. Hepatology. 2013;57(4):1448–1457.
- Ruers T, Van Coevorden F, Punt CJ, et al. Local treatment of unresectable colorectal liver metastases: results of a randomized phase II trial. J Natl Cancer Inst. 2017;109(9):djx015.
- Ruzzenente A, Manzoni GD, Molfetta M, et al. Rapid progression of hepatocellular carcinoma after radiofrequency ablation. World J Gastroenterol. 2004;10(8):1137–1140.
- Kim JS, Ko Y, Kwon H, et al. Impact of energy and access methods on extrahepatic tumor spreading and the ablation zone: an ex vivo experiment using a subcapsular tumor model. Korean J Radiol. 2019;20(4):580–588.
- Brace C. Radiofrequency and microwave ablation of the liver, lung, kidney, and bone: what are the differences? Curr Probl Diagn Radiol. 2009;38(3):135–143.
- Shi L, Wang J, Ding N, et al. Inflammation induced by incomplete radiofrequency ablation accelerates tumor progression and hinders PD-1 immunotherapy. Nat Commun. 2019;10(1):5421.
- Nijkamp MW, Hoogwater FJ, Steller EJ, et al. CD95 is a key mediator of invasion and accelerated outgrowth of mouse colorectal liver metastases following radiofrequency ablation. J Hepatol. 2010;53(6):1069–1077.
- Zhang Z, Zhang Y, Zhang L, et al. Incomplete radiofrequency ablation provokes colorectal cancer liver metastases through heat shock response by PKCα/Fra-1 pathway. Cancer Biol Med. 2019;16(3):542–555.
- Su T, Liao J, Dai Z, et al. Stress-induced phosphoprotein 1 mediates hepatocellular carcinoma metastasis after insufficient radiofrequency ablation. Oncogene. 2018;37(26):3514–3527.
- Yoshida S, Kornek M, Ikenaga N, et al. Sublethal heat treatment promotes epithelial-mesenchymal transition and enhances the malignant potential of hepatocellular carcinoma. Hepatology. 2013;58(5):1667–1680.
- Shady W, Petre EN, Gonen M, et al. Percutaneous radiofrequency ablation of colorectal cancer liver metastases: factors affecting outcomes–a 10-year experience at a single center. Radiology. 2016;278(2):601–611.
- Ahmed M, Solbiati L, Brace CL, Standard of Practice Committee of the Cardiovascular and Interventional Radiological Society of Europe, et al. Image-guided tumor ablation: standardization of terminology and reporting criteria-a 10-year update. Radiology. 2014;273(1):241–260.
- Vietti Violi N, Duran R, Demartines N, et al. Local recurrence rate in patients with colorectal cancer liver metastasis after wedge resection or percutaneous radiofrequency ablation. Int J Hyperthermia. 2018;34(7):1020–1028.
- Han K, Song K, Choi BW. How to develop, validate, and compare clinical prediction models involving radiological parameters: study design and statistical methods. Korean J Radiol. 2016;17(3):339–350.
- Collins GS, Reitsma JB, Altman DG, et al. Transparent reporting of a multivariable prediction model for individual prognosis or diagnosis (TRIPOD): the TRIPOD statement. BMJ. 2015;350:g7594.
- Hatta W, Tsuji Y, Yoshio T, et al. Prediction model of bleeding after endoscopic submucosal dissection for early gastric cancer: BEST-J score. Gut. 2021;70(3):476–484.
- Sotirchos VS, Petrovic LM, Gönen M, et al. Colorectal cancer liver metastases: biopsy of the ablation zone and margins can be used to predict oncologic outcome. Radiology. 2016;280(3):949–959.
- Wang X, Sofocleous CT, Erinjeri JP, et al. Margin size is an independent predictor of local tumor progression after ablation of colon cancer liver metastases. Cardiovasc Intervent Radiol. 2013;36(1):166–175.
- Sadot E, Groot Koerkamp B, Leal JN, et al. Resection margin and survival in 2368 patients undergoing hepatic resection for metastatic colorectal cancer: surgical technique or biologic surrogate? Ann Surg. 2015;262(3):476–485.
- Shirai K, Tamai H, Shingaki N, et al. Clinical features and risk factors of extrahepatic seeding after percutaneous radiofrequency ablation for hepatocellular carcinoma. Hepatol Res. 2011;41(8):738–745.
- Jaskolka J, Asch M, Kachura J, et al. Needle tract seeding after radiofrequency ablation of hepatic tumors. J Vasc Interv Radiol. 2005;16(4):485–491.
- Kang T, Lim H, Lee M, et al. Long-term therapeutic outcomes of radiofrequency ablation for subcapsular versus nonsubcapsular hepatocellular carcinoma: a propensity score matched study. Radiology. 2016;280(1):300–312.
- Teratani T, Yoshida H, Shiina S, et al. Radiofrequency ablation for hepatocellular carcinoma in so-called high-risk locations. Hepatology. 2006;43(5):1101–1108.
- Sartori S, Tombesi P, Macario F, et al. Subcapsular liver tumors treated with percutaneous radiofrequency ablation: a prospective comparison with nonsubcapsular liver tumors for safety and effectiveness. Radiology. 2008;248(2):670–679.
- Worakitsitisatorn A, Lu DS, Lee MW, et al. Percutaneous thermal ablation of subcapsular hepatocellular carcinomas: influence of tumor-surface contact and protrusion on therapeutic efficacy and safety. Eur Radiol. 2020;30(3):1813–1821.
- Kang TW, Lim HK, Lee MW, et al. Perivascular versus nonperivascular small HCC treated with percutaneous RF ablation: retrospective comparison of long-term therapeutic outcomes. Radiology. 2014;270(3):888–899.
- Jiang B, Luo H, Yan K, et al. Ten-year outcomes of percutaneous radiofrequency ablation for colorectal cancer liver metastases in perivascular vs. non-perivascular locations: a propensity-score matched study. Front Oncol. 2020;10:553556.
- Steinke K, Haghighi KS, Wulf S, et al. Effect of vessel size on creation of hepatic radiofrequency lesions in pigs assessment of the heat sink effect. AJR Am J Roentgenol. 2002;178(1):47–51.
- Sofocleous CT, Petre EN, Gonen M, et al. CT-guided radiofrequency ablation as a salvage treatment of colorectal cancer hepatic metastases developing after hepatectomy. J Vasc Interv Radiol. 2011;22(6):755–761.
- Zhao Z, Wu J, Liu X, et al. Insufficient radiofrequency ablation promotes proliferation of residual hepatocellular carcinoma via autophagy. Cancer Lett. 2018;421:73–81.
- Wu H, Li SS, Zhou M, et al. Palliative radiofrequency ablation accelerates the residual tumor progression through increasing tumor-infiltrating MDSCs and reducing T-cell-mediated anti-tumor immune responses in animal model. Front Oncol. 2020;10:1308.
- Zhang Y, Peng Z, Chen M, et al. Elevated neutrophil to lymphocyte ratio might predict poor prognosis for colorectal liver metastasis after percutaneous radiofrequency ablation. Int J Hyperthermia. 2012;28(2):132–140.
- Gillams A, Goldberg N, Ahmed M, et al. Thermal ablation of colorectal liver metastases: a position paper by an international panel of ablation experts, The Interventional Oncology Sans Frontières meeting 2013. Eur Radiol. 2015;25(12):3438–3454.
- Jiang BB, Yan K, Zhang ZY, et al. The value of KRAS gene status in predicting local tumor progression of colorectal liver metastases following radiofrequency ablation. Int J Hyperthermia. 2019;36(1):211–219.
- Donadon M, Lleo A, Di Tommaso L, et al. The shifting paradigm of prognostic factors of colorectal liver metastases: from tumor-centered to host immune-centered factors. Front Oncol. 2018;8:181.