Abstract
Purpose
This study aimed to investigate the technical efficiency and therapeutic response of fusion imaging (considered as virtual navigation) between contrast-enhanced ultrasound (CEUS) and contrast-enhanced computed tomography/magnetic resonance imaging (CECT/CEMRI) for the guidance of radiofrequency ablation (RFA) in patients with residual hepatocellular carcinoma (HCC) after transcatheter arterial chemoembolization (TACE).
Methods
For this prospective study, 98 patients with residual HCC lesions after TACE treatment were enrolled between June 2017 and December 2020. All the lesions were invisible on conventional ultrasound scans. Percutaneous RFA was performed using either CEUS (CEUS group, 52 lesions) or virtual navigation (VN group, 46 lesions) guidance. The lesion display rate, disease-free survival rate, local recurrence rate, overall survival rate and complication incidence were calculated and compared.
Results
Fusion imaging had a significant impact on the RFA outcomes (hazard ratio, 2.629; 95% confidence interval, 1.256–5.505; p = .01). The median disease-free survival time of the VN group was significantly higher than that of the CEUS group (10.9 vs. 8.8 months; p = .007). The local recurrence rates after 3, 6 and 12 months in the VN group were significantly lower than those in the CEUS group (p = .014, .002 and .011). The minor complication rate was not significantly different between the two groups.
Conclusions
CEUS–CECT/CEMRI fusion imaging for guiding RFA enables an efficient and useful therapy of inconspicuous HCC lesions after TACE. The novel solution prolongs the disease-free survival time and reduces the long-term local recurrence of residual lesions treated when using virtual-navigation (VN)-guided RFA.
Introduction
The latest Global Cancer Statistics indicate that liver cancer is the sixth most common and invasive cancer worldwide [Citation1]. Moreover, the 5-year survival rate of liver cancer barely reaches 18%, making it one of the most deadly tumor types, only surpassed by pancreatic carcinomas [Citation2]. Hepatocellular carcinomas (HCC) account for most primary liver cancers (75–85%) [Citation1,Citation3]. Although surgery remains an effective primary treatment for primary liver cancer, advanced stage patients cannot be subjected to surgery due to their limited reserve of liver function, vascular involvement and other compromising factors [Citation4]. Transcatheter arterial chemoembolization (TACE) is the preferred conservative treatment for patients who cannot undergo surgery [Citation5]. In fact, Barcelona Clinic Liver Cancer considers TACE as the standard treatment for stage B HCC [Citation6]. Nevertheless, a single TACE intervention often fails to completely inactivate all tumor cells, and postoperative pathological results have shown that less than 69.4% of tumor tissues are completely necrotic after TACE, indicating a higher recurrence and metastasis rate after a single intervention [Citation7]. Therefore, strengthening the treatment of liver cancer recurrence after TACE is of great clinical significance.
Radiofrequency ablation (RFA) has been widely used as an alternative treatment for small or multiple HCCs, showing more promising results than surgery [Citation8,Citation9]. Furthermore, RFA can effectively supplement TACE [Citation10,Citation11]. From the various guiding modalities for percutaneous RFA, ultrasound provides numerous advantages [Citation12], such as simple operation, real-time boot, no radiation damage and relatively low cost. However, not all residual lesions are suitable for percutaneous-ultrasound-guided RFA because it is difficult to accurately determine the active region of recurrent or residual lesions due to the effect of lipiodol. Additionally, the performance of two-dimensional ultrasound is limited by soft tissue resolution, scanning section and physical acoustics. Fortunately, various techniques can be adopted to overcome these problems, such as contrast-enhanced computed tomography (CECT), contrast-enhanced magnetic resonance imaging (CEMRI) and contrast-enhanced ultrasound (CEUS).
Fusion imaging can provide real-time information from both ultrasound and computed tomography or magnetic resonance imaging (MRI) scans on a single screen by overlaying on an ultrasound image. Thus, fusion imaging is being increasingly used in clinical practice, contributing with the development of interventions for liver lesions, RFA, and evaluation methods for the efficacy, regeneration or recurrence of HCC after intervention [Citation13]. Furthermore, invisible lesions on ultrasound can be visualized through CEUS–CECT/CEMRI fusion imaging [Citation14,Citation15].
To the best of our knowledge, this is the first study to evaluate the value of virtual-navigation (VN)-guided RFA in the treatment of HCC residual after TACE and to compare this technique with percutaneous CEUS-guided RFA. We aimed to introduce an imaging monitoring method for HCC patients whose lesions are not clearly visible by conventional ultrasound after interventional surgery.
Materials and methods
Patients
For this study, patients with HCC who underwent TACE and planned to undergo RFA were recruited between June 2017 and December 2020. This study was approved by the hospital institutional review board, and informed written consent was obtained from all the participants. Patients with residual or recurrent HCC underwent CEUS- or VN-guided RFA according to their preference, obtaining a random distribution of the procedures.
The inclusion criteria were as follows: 1) patients were confirmed to have HCC by pathological examination before TACE; 2) imaging evidence (CECT or CEMRI) indicated that the patient had residual HCC; 3) the lesion was single and invisible on conventional ultrasound; 4) patients were unsuitable or refused hepatectomy; and 5) CECT/CEMRI scans were available in the DICOM format.
The exclusion criteria were as follows: 1) patients with Child–Pugh class C cirrhosis; 2) maximum diameter of the residual viable tumor above 5 cm; 3) patients with coagulation dysfunction; and 4) follow-up for patients below 1 year.
CEUS examination
The CEUS examination proceeded as follows. 1) Using the Esaote MyLab 90 diagnostic ultrasound system, an abdominal probe CA541 (1–8 MHz) was selected and adjusted to CEUS mode. 2) A solution of 2.4 ml SonoVue contrast agent (Bracco, Milan, Italy) was injected into the vein as a bolus, followed by a flush with saline solution (5 ml). 3) Simultaneously, the image storage function was started, and recording was conducted for 3 min.
Ultrasound with fusion imaging before RFA
VN fusion imaging system included an ultrasonic diagnostic instrument with navigation software, magnetic field generator, and two-position sensors. First, the VN fusion imaging system was connected to the ultrasound scanner while the patient lay in the operating bed. Second, the operator began to scan the patient’s abdomen and located the target lesion. Third, CECT/CEMRI data (DICOM format) were imported to the VN fusion imaging system. Image registration between B-mode ultrasound and CECT/CEMRI scans was applied as in [Citation16]. Fourth, CEUS imaging was applied routinely to confirm the size and location of the lesions. Finally, the proper type of electrode needle and the needle route was selected, and VN-guided RFA was performed.
Percutaneous RFA
Following different guidance methods of percutaneous liver cancer ablation, the patients were divided into two groups: 1) CEUS-guided RFA group (CEUS group) and 2) VN + CEUS-guided RFA group (VN group). RFA was performed on patients under local anesthesia combined with an intravenous injection of sober sedatives and analgesics. Then, RFA was applied using the same system under the guidance of VN or CEUS. The Cool-tip RFA system (Covidien, Mansfield, MA) was used. The system consisted of a radiofrequency generator with a maximum power of 200 W and a 17 g internal cool straight electrode. The index of RFA termination was the echo-bubble covering the target lesion as observed on the fusion CEUS scan with a sufficiently safe boundary of at least 5 mm. After RFA, the electrodes were removed. Moreover, to prevent iatrogenic tumor cell spreading and implantation and reduce the risk of bleeding, the puncture path was cauterized while the electrode was removed.
The RFA therapeutic parameters, duration of surgery, and complications were recorded. A routine CEUS examination was applied to assess whether the target lesion was ablated completely and to determine the occurrence of any RFA-associated complications. Ultrasound–CECT/CEMRI fusion imaging was used to check whether the non-perfusion area on the CEUS image covered the target. If not, RFA under VN guidance was immediately repeated to achieve complete coverage.
Assessment of ablation areas and follow-up
Immediately after RFA, the ablation area of the VN group was evaluated by VN and CEUS. The intervention should cover the tumor region and reach the safe ablation boundary of 5 mm. In the CEUS group, CEUS was used to evaluate the ablation area. Whether a second RFA was required after 10 min depended on the ablation area.
CEMRI or CECT scans and the alpha-fetoprotein were reexamined 1 month after the first RFA intervention to evaluate the technical efficiency. Follow-up was performed every 3 months with ultrasound and CEUS and every 6 months with CECT/CEMRI. The local recurrence rate, disease-free survival rate, overall survival rate and complications after RFA were evaluated.
Statistical analysis
The statistical analysis was conducted using SPSS version 23.0 (IBM, Armonk, NY). Continuous and categorical variables were expressed as medians with range and numbers and percentages, respectively. The continuous variables were compared using the t-test, and the categorical ones were compared using the chi-squared test. The Kaplan–Meier estimator was used for survival analysis, and the difference between the two groups was compared using the log-rank test. The significant factors for survival were determined using univariate and multivariate Cox regression analyses. Statistical significance was set to p < .05.
Results
Population characteristics and tumor profiles
A total of 98 patients who met the inclusion criteria were enrolled in the study. The clinical characteristics of the patients in the two groups are summarized in . In the VN group, 35 cases fused CEUS with CEMRI, while 11 cases fused CEUS with CECT. The results of CEUS-guided RFA showed that four residual viable HCC lesions were unclear. The residual HCC area display rate was 100% (46/46) in the VN group and 92.3% (48/52) in the CEUS group. and show the CEUS- and VN-guided RFA, respectively.
Figure 1. Contrast-enhanced ultrasound-guided radiofrequency ablation. (a) Contrast-enhanced ultrasound showed some residual viable hepatocellular carcinomas in the arterial phase with high enhancement after transcatheter arterial chemoembolization. (b) The blue arrow represents the radiofrequency ablation needle.
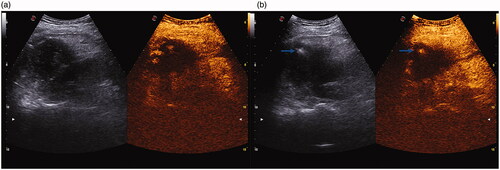
Figure 2. Virtual navigation-guided radiofrequency ablation. The green boundary area represents the entire post-TACE area it is difficult to identify the residual viable HCC area using CEUS alone. (a) The fusion image of CEUS and CEMRI showed that the residual viable HCC of both were well matched; (b) The cross marker is an active part of HCC in MRI image, indicating the incomplete deposition of lipiodol.
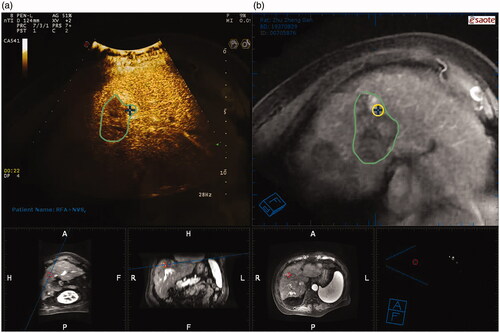
Table 1. Patient characteristic.
Therapeutic RFA efficiency and complications
The median follow-up period was 8.5 months (range, 1–12 months), and a patient died and thus lost the follow-up. The estimated 1-, 3-, 6- and 12-month disease-free survival rates were 97.8% (45/46), 95.6% (44/46), 87.0% (40/46) and 78.3% (36/46) in the VN group and 94.2% (49/52), 78.8% (41/52), 59.6% (31/52) and 53.8% (28/52) in the CEUS group, respectively. The 12-month overall survival rate was 89.1% in the VN group and 78.8% in the CEUS group. Furthermore, the recurrence rates at 3, 6 and 12 months in the VN group were significantly lower than those in the CEUS group. There were no significant differences in the recurrence rates at 1 month between the two groups ().
Table 2. The clinical outcomes and therapeutic response.
No intervention-related deaths occurred during the follow-up period. Minor complications occurred in 13 cases (28.3%) in the VN group, including fever (7 cases), biloma formation (1 case), and abdominal pain (5 cases). Mild complications occurred in 18 of the 52 patients (34.6%) in the CEUS group, including fever (10 cases), abdominal pain (6 cases) and biloma formation (2 cases). No major complications occurred in either group.
Correlation between clinical factors and ablation outcome
Factors associated with the prognosis of RFA were studied using univariate Cox regression analysis. The results showed that sex, age, and the maximum diameter of the lesion had no significant influence on the local recurrence rate after RFA, while the application of VN had a significant impact on the RFA outcome (hazard ratio, 2.629; 95% confidence interval, 1.256–5.505; p = .01) ().
Table 3. Cox regression analysis of clinical factors associated with ablation outcome.
Effect of VN on survival after RFA
According to the Kaplan–Meier curve, the ablation prognosis showed that the median disease-free survival time of the two groups was 9.8 months (). In addition, the log-rank test showed that the median disease-free survival time of the VN group (10.9 months) was significantly higher than that of the CEUS group (8.8 months) (χ2 = 7.17; p = .007) ().
Discussion
We prospectively investigated the feasibility and therapeutic effect of CEUS–CECT/CEMRI fusion imaging for guiding RFA to treat residual viable HCC lesions after TACE.
Treatment with TACE for HCC is likely to leave remnants and lead to recurrence for the following reasons: 1) TACE causes hypoxic ischemia of tumor cells, thus promoting the upregulation of vascular endothelial growth factor expression [Citation17]; 2) portal vein is the main blood supply in some tumors [Citation18]; 3) partial recanalization can occur in tumor tissue embolization vessels [Citation19], and the deposited embolization agent is continuously washed away by the blood flow, hindering the complete truncation of tumor blood vessels; and 4) repeated TACE treatment can further aggravate preexisting liver function impairments.
RFA is an effective treatment for residual or recurrent lesions after TACE, but it requires the target lesion to be clearly visible on ultrasound. In routine ultrasound examinations, however, residual HCC lesions after TACE are often uncertain and insidious given the incomplete deposition of lipiodol. In addition, tumors at specific sites (e.g., diaphragm top, great vessels and hepatic portal vein), residual tumors with irregular shapes, and changes in tumor sonogram echo hamper the accurate targeting of lesions by conventional ultrasound-guided RFA. Such residual tumors or recurrent tissues can be detected on CECT or CEMRI scans. However, these imaging techniques are limited by static image, radiation and the operator experience. Consequently, they are not widely used to guide RFA. Furthermore, the enhancement evaluation on CECT/CEMRI scans for tumors with oil deposition is challenging owing to artifacts produced by the high attenuation of iodized oil [Citation20,Citation21]. We hypothesized that CEUS–CECT/CEMRI fusion imaging can provide the advantages of these techniques while mitigating their drawbacks, as illustrated in .
Figure 4. A representative case for VN-guided RFA. (a) CEUS demonstrates residual viable HCC with red border. The green boundary area represents the entire post-TACE area; (b) The cross marks the active area after TACE on CEMRI image; (c) The superposition of CEUS, CEMRI and MRI image; (d) The cross marker is an active part of HCC in MRI image, indicating the incomplete deposition of lipiodol. VN: virtual navigation; RFA: radiofrequency ablation; CEUS: contrast-enhanced ultrasound; HCC: hepatocellular carcinoma; TACE: transcatheter arterial chemoembolization; CEMRI: contrast-enhanced magnetic resonance imaging; MRI: magnetic resonance imaging.
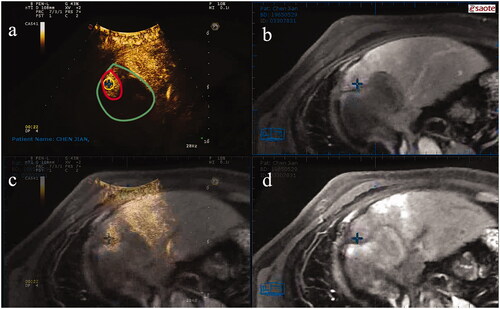
First, we found that CEUS–CECT/CEMRI fusion imaging showed a higher lesion display rate (46/46, 100%) than CEUS alone (48/52, 92.3%). Therefore, fusion imaging outperforms CEUS for monitoring unobvious nidus. Previous studies have indicated that CEUS–CEMRI fusion imaging can detect smaller and fuzzier HCCs than conventional ultrasound or CEUS alone [Citation14,Citation22]. For inconspicuous lesions after TACE observed on conventional ultrasound, CEUS, and CECT/CEMRI scans, fusion imaging may be the best choice. CEUS allows to dynamically observe blood perfusion and microvascular network distribution of tumors, and combined with the high spatial resolution of CECT/CEMRI, it can substantially enhance RFA guidance.
In previous study [Citation14,Citation23,Citation24], fusion imaging was combined with CEUS to guide percutaneous RFA of liver cancer. However, most of these studies were retrospective, likely leading to selection bias and complicating the assessment of long-term treatment outcomes. In addition, no study has reported the value of VN-guided RFA to treat residual viable HCC after TACE.
Regarding the factors associated with the disease-free survival rate after RFA, we found that sex, age, and maximum lesion diameter did not cause significant differences between the two groups, but VN was a significant factor for the RFA outcome. We also found that the local recurrence rate in the CEUS group was significantly higher at 3, 6 and 12 months after RFA than that in the VN group. Moreover, no significant difference was observed in the local recurrence rate between the two groups 1 month after RFA. Similarly, other studies have shown that the difference in recurrence is small during early follow-up, but it tends to be significant in late follow-ups [Citation25].
CEUS–CECT/CEMRI fusion imaging can increase the disease-free survival time because it enables more accurate RFA of lesions under guidance of VN compared with the use of CEUS alone. In fact, under VN guidance, the operator has more time to place the needle, and it is easier to determine the shape or size of the lesion and the three-dimensional spatial relation between the lesion and surrounding structures. In this study, we observed no significant differences regarding postoperative complications and no major complications or operation-related deaths between the two groups. These findings suggest that CEUS–CECT/CEMRI fusion imaging significantly improves ultrasound for guiding RFA. This conclusion is consistent with other reports [Citation22,Citation26].
We used SonoVue as the ultrasound contrast agent, which does not provide a post-vascular phase, resulting in a short display time for the detection of viable HCC areas during the arterial phase. In contrast, other studies have demonstrated that Sonazoid provides a post-vascular phase known as the Kupffer phase (10 min after injection and lasting 1 h) [Citation27,Citation28]. Thus, Sonazoid-enhanced CEUS may show both the necrotic area and the viable HCC area as a defective area during the post-vascular phase. An additional Sonazoid injection can allow to classify the necrotic area and viable HCC area because the latter shows hypervascularity. Thus, Sonazoid-enhanced CEUS-guided RFA seems promising for residue therapy after TACE. In future studies, Sonazoid-enhanced CEUS, SonoVue-enhanced CEUS and VN-guided RFA should be compared.
Various limitations of this study should be considered. First, although this was a prospective study, it included patients from a single center and a small sample size with short follow-up time. Hence, the long-term efficacy and survival rate should be further investigated. Second, all residual cancer lesions after TACE were invisible by conventional ultrasound, and the treatment was percutaneous-ultrasound-guided RFA, excluding computed tomography guidance. Further studies should be conducted on patients from multiple centers and considering a larger sample size, long-term follow-ups, percutaneous ultrasound guidance, percutaneous computed tomography guidance and other methods that allow to confirm our findings.
In conclusion, we confirmed that VN-guided RFA is an efficient and useful therapeutic option for residual viable HCC after TACE when conventional ultrasound detection fails. In addition, the long-term recurrence rate of residual HCC lesions treated with VN-guided RFA was significantly lower than that of CEUS-guided RFA.
Disclosure statement
No potential conflict of interest was reported by the author(s).
Additional information
Funding
References
- Bray F, Ferlay J, Soerjomataram I, et al. Global cancer statistics 2018: GLOBOCAN estimates of incidence and mortality worldwide for 36 cancers in 185 countries. CA Cancer J Clin. 2018;68(6):394–424.
- Jemal A, Ward EM, Johnson CJ, et al. Annual report to the nation on the status of cancer, 1975–2014, featuring survival. J Natl Cancer Inst. 2017;109(9):djx030.
- Villanueva A. Hepatocellular carcinoma. N Engl J Med. 2019;380(15):1450–1462.
- Dhir M, Melin AA, Douaiher J, et al. A review and update of treatment options and controversies in the management of hepatocellular carcinoma. Ann Surg. 2016;263(6):1112–1125.
- Boulin M, Delhom E, Pierredon-Foulongne MA, et al. Transarterial chemoembolization for hepatocellular carcinoma: an old method, now flavor of the day. Diagn Interv Imaging. 2015;96(6):607–615.
- Hirooka M, Hiraoka A, Ochi H, et al. Transcatheter arterial chemoembolization with or without radiofrequency ablation: outcomes in patients with Barcelona clinic liver cancer stage B hepatocellular carcinoma. AJR Am J Roentgenol. 2018;210(4):891–898.
- Arata S, Tanaka K, Okazaki H, et al. Risk factors for recurrence of large HCC in patients treated by combined TAE and PEI. Hepatogastroenterology. 2001;48(38):480–485.
- EASL clinical practice guidelines: management of hepatocellular carcinoma. J Hepatol. 2018;69(1):182–236.
- Heimbach JK, Kulik LM, Finn RS, et al. AASLD guidelines for the treatment of hepatocellular carcinoma. Hepatology. 2018;67(1):358–380.
- Gillams A, Goldberg N, Ahmed M, et al. Thermal ablation of colorectal liver metastases: a position paper by an international panel of ablation experts, The Interventional Oncology Sans Frontières meeting 2013. Eur Radiol. 2015;25(12):3438–3454.
- Keane FK, Hong TS, Zhu AX. Evolving systemic therapy in hepatocellular carcinoma: current management and opportunities for integration with radiotherapy. Semin Radiat Oncol. 2018;28(4):332–341.
- Wu J, Chen P, Xie YG, et al. Comparison of the effectiveness and safety of ultrasound- and CT-guided percutaneous radiofrequency ablation of non-operation hepatocellular carcinoma. Pathol Oncol Res. 2015;21(3):637–642.
- Calandri M, Mauri G, Yevich S, et al. Fusion imaging and virtual navigation to guide percutaneous thermal ablation of hepatocellular carcinoma: a review of the literature. Cardiovasc Intervent Radiol. 2019;42(5):639–647.
- Bo XW, Xu HX, Wang D, et al. Fusion imaging of contrast-enhanced ultrasound and contrast-enhanced CT or MRI before radiofrequency ablation for liver cancers. BJR. 2016;89(1067):20160379.
- Lee MW, Lim HK, Rhim H, et al. Percutaneous radiofrequency ablation of small (1-2 cm) hepatocellular carcinomas inconspicuous on B-mode ultrasonographic imaging: usefulness of combined fusion imaging with MRI and contrast-enhanced ultrasonography. Can J Gastroenterol Hepatol. 2018; 2018(2018):7926923.
- Zhao QY, Xie LT, Chen SC, et al. Virtual navigation-guided radiofrequency ablation for recurrent hepatocellular carcinoma invisible on ultrasound after hepatic resection. Hepatobiliary Pancreat Dis Int. 2020;19(6):532–540.
- Ichihara T, Sakamoto K, Mori K, et al. Transcatheter arterial chemoembolization therapy for hepatocellular carcinoma using polylactic acid microspheres containing aclarubicin hydrochloride. Cancer Res. 1989;49(15):4357–4362.
- Pesapane F, Nezami N, Patella F, et al. New concepts in embolotherapy of HCC. Med Oncol. 2017;34(4):58
- Nakai M, Sato M, Kawai N, et al. Hepatocellular carcinoma: involvement of the internal mammary artery. Radiology. 2001;219(1):147–152.
- Ito K, Honjo K, Fujita T, et al. Therapeutic efficacy of transcatheter arterial chemoembolization for hepatocellular carcinoma: MRI and pathology. J Comput Assist Tomogr. 1995;19(2):198–203.
- Najmi Varzaneh F, Pandey A, Aliyari Ghasabeh M, et al. Prediction of post-TACE necrosis of hepatocellular carcinoma usingvolumetric enhancement on MRI and volumetric oil deposition on CT, with pathological correlation. Eur Radiol. 2018;28(7):3032–3040.
- Ahn SJ, Lee JM, Lee DH, et al. Real-time US-CT/MR fusion imaging for percutaneous radiofrequency ablation of hepatocellular carcinoma. J Hepatol. 2017;66(2):347–354.
- Lee MW. Fusion imaging of real-time ultrasonography with CT or MRI for hepatic intervention. Ultrasonography. 2014;33(4):227–239.
- Min JH, Lim HK, Lim S, et al. Radiofrequency ablation of very-early-stage hepatocellular carcinoma inconspicuous on fusion imaging with B-mode US: value of fusion imaging with contrast-enhanced US. Clin Mol Hepatol. 2014;20(1):61–70.
- Schullian P, Johnston E, Laimer G, et al. Thermal ablation of CT ‘invisible’ liver tumors using MRI fusion: a case control study. Int J Hyperthermia. 2020;37(1):564–572.
- Kim JW, Shin SS, Heo SH, et al. Ultrasound-guided percutaneous radiofrequency ablation of liver tumors: how we do it safely and completely. Korean J Radiol. 2015;16(6):1226–1239.
- Yanagisawa K, Moriyasu F, Miyahara T, et al. Phagocytosis of ultrasound contrast agent microbubbles by Kupffer cells. Ultrasound Med Biol. 2007;33(2):318–325.
- Watanabe R, Matsumura M, Munemasa T, et al. Mechanism of hepatic parenchyma-specific contrast of microbubble-based contrast agent for ultrasonography: microscopic studies in rat liver. Invest Radiol. 2007;42(9):643–651.