Abstract
Aim
Swarming motility is a virulence factor for Proteus mirabilis and is a coordinated multicellular movement of bacteria. In this study, we investigated the inhibitory effect of hyperthermia on bacterial swarming motility and antimicrobial resistance.
Methods
Thirty-one P. mirabilis isolates were included in the study. Seven inoculated agar plates were incubated inside incubators with increasing temperature levels: at 36 °C (control) and 40–45 °C. On the next day, inhibition of swarming was evaluated and minimum paralyzing temperature (MPT) values were determined. An antimicrobial susceptibility test (antibiogram) is performed by exposing bacteria to increasing concentrations of antibiotics, in vitro. Thus, we used the Kirby-Bauer disk diffusion test as a screening method to analyze the antibiogram profiles of the isolates at 36 °C and 42 °C. Finally, a time-kill assay was performed to analyze the killing effect of hyperthermia (42 °C) on planktonic bacteria, in combination with the antibiotic meropenem at the first and third hours. A Wilcoxon signed-rank test was used to compare the killing effects of meropenem, hyperthermia and their combinations.
Results
The median MPT value was determined as 44 °C. In the disk diffusion assay, susceptibility development was observed in 94% of isolates for at least one antibiotic. In the time-kill assay, we observed a significant killing effect of hyperthermia in combination with meropenem. Under the microscope, we observed the formation of spherical cells by the effect of heat.
Conclusion
We conclude that these findings might be useful when employing the hyperthermia method to treat infectious diseases caused by P. mirabilis in the future.
Graphical Abstract
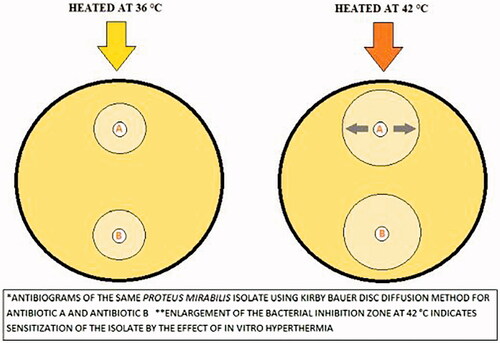
Introduction
Mild hyperthermia is a therapeutic technique in which the target tissue is heated above body temperature to induce a physiological or biological effect, but it is often not intended to directly produce substantial cell death. In this method, the aim is to obtain temperatures of 40–45 °C for time periods up to 120 min [Citation1]. Some microorganisms are known to be sensitive to temperatures at the level of hyperthermia [Citation2,Citation3]. So, this sensitivity may help us to eliminate thermo-susceptible microbial pathogens by using local heat therapy [Citation2–5].
The swarming phenomenon is a rapid and coordinated translocation of a bacterial population across solid or semi-solid surfaces and is an example of bacterial multi-cellularity [Citation6]. Swarming motility has been shown in different Gram-negative bacterial pathogens including the genera Salmonella, Yersinia, Pseudomonas and Proteus [Citation7–10]. It has also been reported that some swarming bacterial species such as Salmonella typhimurium show elevated resistance to certain antibiotics compared to undifferentiated and non-swarming cells [Citation11].
Proteus mirabilis is a Gram-negative rod-shaped bacterium from the order of Enterobacterales. It is well-known for its urease production and distinctive ability to differentiate into elongated swarming cells, and its characteristic bull’s-eye/circular pattern of swarming motility on agar plates [Citation12,Citation13]. Swarming cells of P. mirabilis migrate rapidly and in a coordinated manner away from the colony as multicellular rafts until they pause in the consolidation stage and undergo some de-differentiation [Citation14,Citation15]. Regular cycles of migration and consolidation generate a colony on the agar surface with a characteristic circular swarming pattern [Citation14,Citation15]. P. mirabilis swarming involves the coordinate differentiation of short, motile, vegetative cells with a few peri-trichous flagella into multi-nucleate, hyper-flagellated swarm cells of up to 40 times the vegetative cell length and with much more surface density of flagella. The ability of P. mirabilis to express virulence factors, including urease and hemolysin, and to invade human urothelial cells, is reported to be coordinately regulated with swarming differentiation [Citation15–17].
P. mirabilis is the most commonly isolated species from clinical samples, with 90% being from urinary infections, but also from other infections including those of the respiratory system, eye, ear, nose, and skin, and burn and wound infections [Citation18]. Resistance genes to antibiotic families including beta-lactams, aminoglycosides, quinolones and carbapenems, are increasingly identified in P. mirabilis. These genes may be carried on genetic elements such as plasmids, transposons and on integrons that can be mobilized [Citation12]. Previously, the emergence of multidrug-resistant P. mirabilis-producing extended spectrum beta lactamase, AmpC, and carbapenemases has been reported [Citation19,Citation20]. Since there is a trend of developing resistance to various antimicrobial drugs, it is important to investigate new drugs, combination therapies or methods to overcome antimicrobial resistance problems caused by P. mirabilis bacteria.
In the field of clinical microbiology, an antimicrobial susceptibility test (antibiogram) is performed by exposing pathogen bacteria to increasing concentrations of an antibiotic, in vitro. Clinical microbiologists try to isolate and identify bacterial and fungal agents from patient samples using culturing methods. Afterwards, they perform antibiogram tests to determine the minimum inhibitory concentration (MIC) of an antimicrobial drug. If the MIC value is lower than a determined threshold, the isolate is accepted as susceptible/sensitive to that antimicrobial [Citation2,Citation21]. These threshold values (critical breakpoints) are determined by scientific committees for each bacterial/fungal species and antimicrobial drug according to the drug’s achievable concentrations. Nowadays, the Kirby-Bauer disk diffusion method is widely used in clinical microbiology laboratories since it has a strong correlation with reference antibiogram methods and is easy to perform [Citation21]. Similarly, the thermobiogram method can be used to determine the minimum inhibitory temperature (MIT) of an isolate by incubating bacteria in various incubators with increasing temperature levels [Citation2]. After one night of incubation, the lowest temperature value inhibiting the microbial growth can be determined as the MIT. The thermobiogram method can be modified and used to analyze bacterial swarming or synergistic effects of antibiotics at different temperatures.
Since swarming is an organized movement and accepted as a virulence factor for P. mirabilis, we hypothesized that hyperthermia may be employed to overcome bacterial infections by inhibiting the swarming motility of this pathogen. In this study, we aimed to investigate the inhibitory effect of in vitro hyperthermia on the swarming motility of P. mirabilis clinical isolates. Secondly, we compared the antimicrobial susceptibility profiles and bacterial killing of P. mirabilis isolates at body temperature and the temperature level of hyperthermia in order to determine whether hyperthermia will cause susceptibility to any antimicrobial drug.
Methods
Bacterial isolates
Thirty-one Proteus mirabilis clinical isolates recovered from patients in a university hospital (Gaziantep, Turkey) between January 2019 and December 2020 were included in the study. Ethical approval and transfer documents have been obtained from the Gaziantep University Clinical Researches Ethical Committee with date and number: 2021/05. The isolates were obtained from cultures of urine (n = 13), wound (n = 7), tracheal aspirate (n = 6), blood (n = 2), cerebrospinal fluid (n = 1), catheter (n = 1) and sputum (n = 1) samples during routine microbiological examinations in the clinical microbiology laboratory of Sanko University Hospital. The study isolates were selected among imipenem non-susceptible strains in order to see the sensitization effect of hyperthermia on the antimicrobial resistance profiles of multi-drug resistant bacteria. Only one isolate per patient was included to avoid repeat samples. All bacterial isolates were stored at −80 °C until further testing. Deep frozen isolates were thawed and sub-cultured twice prior to testing. The identification and routine susceptibility testing of the isolates was performed using the Vitek2 automated bacterial identification and antibiogram system (bioMérieux, France) before the study [Citation22].
Swarming inhibition test
A modified thermobiogram method was used to investigate the minimum temperature level inhibiting the bacterial swarming motility [Citation2]. Bacterial colony suspensions were prepared from overnight P. mirabilis cultures. Bacterial suspensions with an optical density equal to 0.5 McFarland were diluted by 100 times. 10 μl of a bacterial suspension was inoculated onto 5% sheep blood agar (SBA) (BD, USA). Drops from the bacterial suspension were inoculated onto the center of the agar plate in order to better observe the swarming motility. For each isolate, seven inoculated SBA plates were incubated inside seven different incubators with increasing temperature levels: at 36 °C (control), 40 °C, 41 °C, 42 °C, 43 °C, 44 °C and 45 °C for 24 h. On the next day, inhibition of swarming motility was evaluated by the naked eye and if inhibition was noted, the SBA plate was again incubated at 36 °C for 24 h to see whether the swarming inhibition was irreversible. The minimum temperature value inhibiting the swarming motility was determined for each isolate and called the minimum paralyzing temperature (MPT). For supplementary analysis, a urease test was performed to see the effect of heat on bacterial urease enzyme. Seven urea agar (BD, USA) slants were inoculated with a loopful of bacterial colonies from each isolate and incubated at seven different temperatures (36 °C, 40–45 °C) for 24 h. An hourly check for the yellow to pink color change indicating urease positivity was made and results noted [Citation23].
Kirby-Bauer disk diffusion method
Antimicrobial susceptibility testing at two different temperatures was carried out using the Kirby-Bauer disk diffusion method according to the recommendations of the European Committee on Antimicrobial Susceptibility Testing (EUCAST) [Citation24], and the results were interpreted according to EUCAST guidelines [Citation21]. Overnight bacterial cultures were adjusted to a turbidity equivalent to that of a 0.5 McFarland standard and spread over the entire surface including the rim of a Mueller Hinton agar (MHA; BD, USA) medium using a sterile swab. A panel of 10 antibiotics (Group A, B and C) which were recommended for Enterobacteriaceae in the EUCAST guidelines [Citation21] were tested in the study. Antibiotic disks of imipenem (10 µg), meropenem (10 µg), ampicillin (10 µg), amoxicillin-clavulanate (20–10) µg, ciprofloxacin (5 µg), gentamicin (10 µg), cefazolin (30 µg), cefotaxime (5 µg), trimethoprim-sulfamethoxazole (1.25–23.75 µg), and piperacillin-tazobactam (30–6 µg) were placed on the inoculated MHA plates. The disks were purchased from a commercial medical company (Bioanalyse, Turkey). The inoculated antibiogram plates were incubated at two different temperatures for 20 h: at 36 °C for the control and at 42 °C to see the antimicrobial sensitization effect of in vitro hyperthermia. The diameter of the bacterial inhibition zones was measured with a transparent ruler. Isolates were classified as susceptible (S), susceptible at increased exposure (I), areas of technical uncertainty (ATU), or resistant (R) according to the breakpoints defined by EUCAST 2021 [Citation21]. The terms ‘ATU’ and ‘I’ refer to “intermediate resistance” which is between R and S. Thereafter, antimicrobial susceptibility test results for two different temperatures (36 °C and 42 °C) were compared.
Modified time-kill assay
In this step, we planned to investigate the synergistic effect of hyperthermia in a limited time period (one and three hours) which hyperthermia treatment could be employed clinically. Additionally, using this method, we tried to exclude the agar effect since the diffusion rates of the antibiotics may change with temperature inside the agar plates. A time-kill assay was performed to analyze the killing effect of hyperthermia (at 42 °C) on planktonic bacteria in combination with the antibiotic meropenem. Firstly, overnight bacterial cultures were adjusted to a turbidity equivalent to 0.5 McFarland standard (5 × 108 CFU/ml). Two Mueller Hinton broth (MHB) tubes were inoculated with a standard amount of bacteria to reach 106 CFU/ml final bacterial population and the meropenem concentration inside the tubes was adjusted to 4 µg/ml. The MHB tubes were incubated at 37 °C (body temperature) and 42 °C (hyperthermia) to see the effect of meropenem in combination with hyperthermia. In the same time, MHB tubes (containing 106 CFU/ml bacteria) without meropenem were incubated at 42 °C and 37 °C to see the effect of hyperthermia alone and for control. At the first and third hours, 10 µl amounts from all tubes were inoculated on MHA plates and the colonies growing were counted manually to determine the killing effect of meropenem alone, hyperthermia alone and the meropenem + hyperthermia combination. In the same time, 1:10, 1:100 and 1:1000 dilutions were prepared and 10 µl amounts from these tubes were inoculated on agar plates for counting the bacteria growing at high numbers. A Wilcoxon signed-rank test was used to analyze the killing effects and compare the survival rates of bacteria which were exposed to meropenem alone, hyperthermia alone and the meropenem + hyperthermia combination for one and three hours. Statistical analysis was performed by SPSS for Windows, version 22.0 (IBM Corp. Released 2013). A P value <0.05 was considered as statistically significant.
Transmission electron microscopy
The bacterial isolates incubated at 37 °C and 42 °C for 24 h were imaged under a transmission electron microscope (TEM) to observe the structural alterations caused by the effect of hyperthermia. Firstly, Proteus colonies (a loopful) growing on an SBA plate were transferred into a tube containing 2 ml of MHB. Then, a 1 ml amount was taken and resuspended inside 5 ml of 2.5% glutaraldehyde solution, kept at room temperature for 10 min and centrifuged at 3,000 g for five minutes. The supernatant was discarded and 2.5% glutaraldehyde solution was added to the pellet. The pellet was placed into the well of a u-bottom microplate filled with 1 ml fresh MHA. After two hours at room temperature, the solidified agar piece was scraped from the wall of the well and divided into 1 mm3 pieces using a scalpel tip. The pieces were stored in tubes containing 15 ml of phosphate buffer at 4 °C and conveyed to TEM laboratory. The post-fixation was applied to samples with 1% osmium tetraoxide solution for one hour at 4 °C. Later, dehydration of the samples was achieved by using graded series of alcohol, and a transparency process was applied with propylene oxide. Subsequently, the samples were embedded in epoxy resin in embedding capsules and polymerized in a 60 °C incubator for 24 h. From the blocks obtained, sections of 70 nm thickness were placed on copper grids with an ultra-microtome and the sections were contrasted with uranyl acetate and lead citrate. The contrasted sections were examined and photographed using a TEM (Jeol® JEM1011, Japan).
Results
Swarming inhibition test
P. mirabilis clinical isolates were obtained from urine (45%), wounds (16%), tracheal aspirate (16%), blood (6%), cerebrospinal fluid (3%), and other specimens (12%). The antimicrobial susceptibility profiles of the isolates were as follows: 61% of the isolates were susceptible to piperacillin-tazobactam, 38% of the isolates were susceptible and 22% of the isolates were susceptible at increased exposure (I) to meropenem, 48% of the isolates were susceptible at increased exposure (I) to imipenem, 22% of the isolates were susceptible to amoxicillin-clavulanate, 13% of the isolates were susceptible to gentamicin, 9% of the isolates were susceptible and 3% of the isolates were in the range of ATU to ciprofloxacin, 3% of the isolates were susceptible and 3% of the isolates were susceptible at increased exposure (I) to cefotaxime, 3% of the isolates were susceptible to trimethoprim-sulfamethoxazole and none of the isolates were susceptible to ampicillin and cefazolin. When P. mirabilis isolates were revived on SBA before the study (at 36 °C), all isolates showed typical swarming characteristics as expected and covered all the surface of the SBA plates in 24 h (). In the thermobiogram step (swarm inhibition test), the swarming motilities of all isolates were inhibited at 45 °C (). However; lower temperature levels also had inhibitory effects on swarming motility. For one isolate, MPT was determined as 41 °C; for three isolates, MPT was 42 °C; for two isolates, MPT was 43 °C; for 11 isolates, MPT was 44 °C; and for 14 isolates, MPT was 45 °C (). The median MPT value was determined as 44 °C for all isolates. The isolates that were incubated at 40 °C and 36 °C (control temperature) were not inhibited and showed typical swarming motility by covering all the surface of the SBA plate (). In the last step, SBA plates containing those motility inhibited (paralyzed) clones were again placed inside an incubator at 36 °C for another 24 h, and this time the swarming motility was observed in all isolates on the next day (). Regarding the urease test, all isolates gave positive urease reactions at all temperatures tested (36 °C, 41–45 °C), indicating that hyperthermia has no effect on bacterial urease enzyme ().
Table 1. Results of the swarming inhibition test after 24 h of incubation at increasing temperatures.
Kirby-Bauer disk diffusion method
When we analyze the antibiogram test results at 42 °C, we observed the development of susceptibility for at least one antibiotic in 29 (94%) isolates. A significant increase of the bacterial inhibition zone diameter indicated sensitization of the isolate for the tested antibiotic [Citation21] (). Susceptibility development (at 42 °C) was observed in 64% of the isolates for imipenem, 48% of the isolates for meropenem, 32% of the isolates for piperacillin-tazobactam, 29% of the isolates for cefazolin, 26% of the isolates for ciprofloxacin, 26% of the isolates for cefotaxime, 22% of the isolates for ampicillin, 22% of the isolates for amoxicillin-clavulanate, 13% of the isolates for gentamicin and 3% of the isolates for trimethoprim-sulfamethoxazole antibiotic. The antibiogram test results are summarized in .
Figure 3. Kirby-Bauer disk diffusion test patterns of an isolate at 36 °C and 42 °C. IPM: imipenem; MEM: meropenem; CN: gentamicin; CZ: cefazolin. The plate on the left was incubated at 36 °C while the plate on the right was incubated at 42 °C for the same isolate. The diameter of the inhibitions zone increased at 42 °C for all antibiotics.
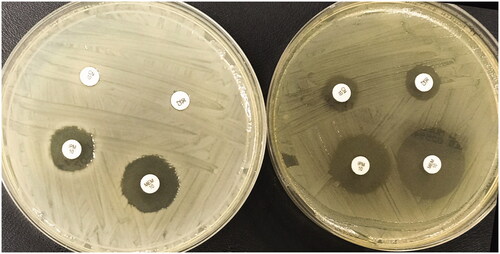
Table 2. Antibiogram results of Proteus mirabilis isolates using the Kirby-Bauer disk diffusion method at 36 °C and 42 °C.
Modified time-kill assay
When we analyzed the results of the time-kill assay using the Wilcoxon signed-rank test, we observed that antibiotic alone or hyperthermia alone did not affect the bacterial survival rate in the first and third hours (compared to control; p > 0.05). However, hyperthermia + meropenem significantly decreased the bacterial population both in the first (p = 0.000006) and third hours (p = 0.000002). Interestingly, there was no significant difference between the survival rates of isolates exposed to hyperthermia for one and three hours in the presence of 4 µg/ml meropenem (p = 0.9508). So, in the presence of 4 µg/ml meropenem, heating for one hour at 42 °C was as effective as heating for three hours at 42 °C. The results are summarized in .
Table 3. Colony counts of the planktonic P. mirabilis isolates in the modified time-kill assay.
Transmission electron microscopy results
We compared the images of bacteria incubated at 37 °C and 42 °C and observed some structural alterations caused by the effect of heat. The most important finding was the formation of spherical cells by the effect of heat. When the bacterial cell morphology was examined under a TEM, a bacillus shape was observed at 37 °C (), while the bacterial morphology changed to a coccus or coccobacillus form at 42 °C (). As another finding, we observed increased vacuolization in the bacterial cells that were exposed to hyperthermia at 42 °C ().
Figure 4. Transmission electron microscopy images of the isolate (No.: 16) incubated at 37 °C and 42 °C. (A) Bacillus forms of Proteus mirabilis are observed after incubation at 37 °C. (B) The elongated bacillus is a typical swarmer cell. (C) Unusual coccus/coccobacillus forms of Proteus mirabilis are observed after incubation at 42 °C. (D) The arrows indicate formations of large vacuoles inside the spherical bacterial cells (cocci).
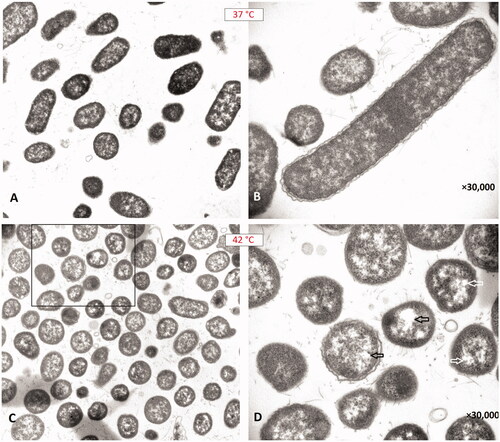
Discussion
Some virulence factors were reported to be responsible for the pathogenicity of P. mirabilis. Among these, flagellum, the motility organelle, was reported to be involved in establishing infection [Citation25]. Previously, it was reported that the expression of the virulence factors was regulated by RsbA coordinately with swarming differentiation in P. mirabilis [Citation17]. In 2006, Wang et al. [Citation26] investigated the inhibitory effects of resveratrol on virulence factors (hemolysin and urease) and swarming motility of P. mirabilis. They concluded that swarming colonies had much higher levels of hemolysin and urease virulence factors compared to non-swarming mutants. In the clinical settings, swarming motility may present an issue for patients with indwelling urinary catheters. P. mirabilis can swarm across the surface of both latex and silicone urinary catheters [Citation27,Citation28]. In addition, P. mirabilis may cause other types of infection including eye, ear, nose, skin, throat, burn, and wound infections. Moreover, it has been reported as a pathogen in neonatal meningoencephalitis, empyema, and osteomyelitis [Citation29,Citation30].
In our study, exposure to a temperature at the level of hyperthermia inhibited the swarming motility of all strains. The swarming motility characteristics of all isolates were inhibited at 45 °C. However; inhibition of swarming motility started at different temperature levels for different P. mirabilis isolates. In our study, we managed to determine an MPT value for each isolate by using the modified thermobiogram method. In our study, MPT was determined as 41 °C only for one isolate, while MPT was determined as 45 °C for the majority of isolates (n = 14). These findings emphasized the importance of performing thermobiogram tests to find the optimum temperature level for hyperthermia treatment. According to our findings, the minimum effective temperature level can be used with minimum thermal damage during a thermal therapy in order to inhibit the swarming of the pathogen bacteria in the future. Secondly we showed that even if hyperthermia does not inhibit the growth of pathogen bacteria, it can be effective by inhibiting the swarming phenomenon which is accepted as an important virulence factor for bacterial species such as P. mirabilis and P. aeruginosa. When we evaluate our thermobiogram study, we think that swarming bacterial strains such as P. mirabilis and P. aeruginosa might become good research candidates for investigating the antimicrobial effects of hyperthermia treatment. Since the hyperthermia treatment method could be applied to human patients at heating levels up to 45 °C, infected patients could benefit from such new thermal treatment methods in the future. Using antimicrobial thermal therapies on superficial body infections such as skin, subcutaneous tissue or wound infections could also be possible. Since swarming is a multicellular and organized movement of these bacteria and is accepted as a virulence factor, hyperthermia can be employed to overcome bacterial infections caused by other swarming bacterial pathogens. In vivo heating of the prosthesis for patients with Pseudomonas or Proteus prosthetic joint infections could help patients get well without the need to remove the infected device. Also, heating the catheters (or inventing heatable catheters) may be alternative methods to reduce the pathogenicity of the swarming bacteria causing catheter infections. There are also some limitations to be mentioned. Although MPT (the minimum paralyzing temperatures) can be applied as described, prolonged heating may not be safe for the patient. So, in the light of our findings, further in vivo and in vitro studies are necessary to better analyze the effect of hyperthermia on swarming motility in shorter heat application periods of about 30–120 min.
In the thermobiogram step, we also performed urease tests for P. mirabilis isolates at all temperature levels. We wanted to investigate whether there was a relation between swarming and urease production; however; we could not find a relation since the isolates whose swarming phenomena were inhibited at 45 °C (or lower temperatures) gave a positive urease reaction in six hours. So, our results contradict those of Wang et al. [Citation26] who reported that swarming colonies had higher levels of urease virulence factors compared to non-swarming mutants. An additional finding was that when we placed the SBA plates containing swarming-inhibited (by hyperthermia) bacterial isolates back in the incubator at 36 °C, we observed that P. mirabilis colonies again started to move and covered the surface of the plate by swarming motility. So, this inhibitory (or paralyzing) effect is reversible and limited to the period of hyperthermia application.
In the next step, we tested the hypothesis that hyperthermia would reduce the antimicrobial resistance levels of P. mirabilis isolates. The Kirby-Bauer disk diffusion test was used for screening the isolates. We tested the temperature level of 42 °C in combination with a standard antimicrobial susceptibility test (antibiogram). When we performed the antibiogram at 42 °C instead of 36 °C, we observed the development of susceptibility for at least one antibiotic in the majority (97%) of the isolates. Regarding these isolates, larger inhibition zones were observed around antibiotic disks at 42 °C, and isolates which are fully resistant (R) to an antibiotic became low resistant (I or ATU) or became susceptible/sensitive (S) by the effect of hyperthermia. The sensitization (susceptibility development) was observed from R to I, R to S or I to S for most of the antimicrobial agents. However, the sensitization was from R to ATU, R to S or ATU to S for ciprofloxacin and piperacillin-tazobactam because of the specific criteria (the ATU category is defined instead of the I category) of these antibiotics in the EUCAST guidelines [Citation21]. As we expected, none of the isolates became resistant to the antimicrobial drugs by the effect of hyperthermia. When we analyze the results, carbapenems were observed to be the most promising antibiotics since susceptibility development (at 42 °C) was observed in 64% of the isolates for imipenem and 48% of the isolates for meropenem. Trimethoprim-sulfamethoxazole and gentamicin were the least effective antibiotics since susceptibility development was observed in only one and four isolates by the effect of hyperthermia, respectively. In light of these findings, antimicrobial chemotherapy in combination with hyperthermia may be a promising tool to treat infections caused by multi-drug or pan-resistant bacterial pathogens because of the sensitization effect of hyperthermia. Since swarming bacteria were reported to be more resistant to the antibiotics, this method could be especially useful for multi-drug resistant bacterial infections [Citation11]. It was reported that dramatic morphological changes associated with swarm-cell differentiation may be indicative of an altered cell wall structure with increased resistance to disruption, consequently decreasing the effectiveness of the cell wall-targeting antibiotics [Citation11]. Accordingly, we observed increased sensitivity to cell wall-targeting antibiotics, transformation to spherical cells and inhibition of swarming motility by the effect of heat. So, both inhibition of swarming motility and increased sensitivity to beta-lactam drugs may be due to the alteration of the cell wall structure. In the future, the inhibitory effects of cyclic heat applications on swarming motility could be investigated to facilitate the adaptation and optimization of these techniques in the clinic.
In a study in 2017, Ricker and Nuxoll [Citation31] investigated the synergistic effects of heat and antibiotics using ciprofloxacin, tobramycin and erythromycin on P. aeruginosa strains and they reported that combined treatments had synergistic effects for heat shock conditions of 60 °C for 5 min to 70 °C for 1 min. Recently, Pijls et al. [Citation32] investigated the synergistic effects of non-contact induction heating and antibiotic cocktails and reported that a combination of heat plus antibiotics had synergistic effects on Staphylococcus aureus biofilms at 60 °C. Both studies achieved promising results; however; they performed their research at high temperatures, which may cause thermal damage to human tissue. Wu et al. [Citation33] designed a magnetic nanoparticle platform to investigate the synergistic effects of nanoparticle heating and the antimicrobial drug amoxicillin on H. pylori and reported that this combination increased the susceptibility of H. pylori to amoxicillin due to the destruction of the cell membrane and microbial biofilm at 41 °C. In contrast to our study, they used amoxicillin-loaded nanoparticles in their research. In 2020, Alumutairi et al. [Citation34] reported that magnetic nanoparticle hyperthermia enhanced the susceptibility of S. aureus biofilm to ciprofloxacin and vancomycin antibiotics. Wardlow et al. [Citation35] developed low temperature-sensitive liposomes containing ciprofloxacin antibiotic for induced release at mild hyperthermia and they reported a triggered release at 42 °C which may cause greater killing and membrane deformation of S. aureus strains. In our study, we demonstrated for the first time that antibiotic-resistant (R) bacterial isolates became susceptible (S) or susceptible at increased exposure (I) to some antibiotics by the direct effect of hyperthermia (at 42 °C) without the need to use nanoparticles, liposomes or any other tools.
In the modified time-kill study, we selected the drug meropenem because it is one of last resort antibiotics to be used in P. mirabilis infection. If a Proteus species is resistant to other drugs such as ampicillin, the patient can be treated with a carbapenem drug (meropenem), which is a last resort antibiotic. However, the problem starts if the pathogen is also resistant to carbapenems [Citation21]. In addition, carbapenems were detected as the most effective combination-antibiotics during our agar screening test. Since imipenem (another carbapenem) is always used in combination with cilastatin compound during antimicrobial chemotherapy, we excluded this carbapenem in the final assay. In the time-kill study, while meropenem alone (at body temperature, 37 °C) or hyperthermia alone had no killing effect on bacterial isolates, a hyperthermia + meropenem combination showed a synergistic effect and significantly decreased the bacterial population even in the first hour. Since hyperthermia therapy can be applied for time periods up to 120 min, we think that this combination therapy could be used to treat some drug resistant Proteus infections in the future. As another finding, we observed bacterial re-growth in seven isolates (No: 1, 5, 8, 18, 19, 20 and 28) in the third hour. The meropenem + hyperthermia combination exhibited a biphasic killing pattern (bacterial killing followed by bacterial re-growth). Thus, three hours of combination therapy may not be appropriate for these isolates.
In our study, hyperthermia not only inhibited the swarming but also caused formation of coccus/coccobacillus appearances in the bacterial populations of P. mirabilis isolates (). Since P. mirabilis is known to have a bacillus shape, this spherical form was unusual for these bacteria. We know that the highly cross-linked peptidoglycan meshwork of the cell wall (produced by penicillin-binding proteins) and the bacterial cytoskeleton are the major determinants of cell shape [Citation36]. Carbapenems are known to bind with high affinity to penicillin-binding protein-2 (PBP2) and then to PBPs 1a and 1 b of Gram-negative bacteria, causing spherical cell formation and lysis [Citation37]. Horii et al. [Citation38] reported that inhibition of penicillin-binding protein-2 (PBP-2) produces spherical non-growing bacterial cells (cocci formation). In addition, they reported that the sub-inhibitory exposure to a carbapenem drug which kills or inhibits P. mirabilis by blocking penicillin-binding protein also caused formation of coccus forms of P. mirabilis, in vitro [Citation38]. Thus, we hypothesize that hyperthermia may act like a carbapenem antibiotic and inhibit the PBP-2 enzyme. This mechanism may cause the formation of non-growing spherical P. mirabilis cells and inhibition of swarming motility at the same time. Accordingly, in the agar screening step, the least effective drugs were trimethoprim-sulfamethoxazole and gentamicin, which are not beta-lactam drugs and do not act on PBPs. We think that these two drugs did not show a synergistic effect with heat since they act on different structures or enzymes than cell wall or PBPs [Citation37]. Another interesting finding of TEM was the increased vacuolization in the bacterial cells exposed to hyperthermia at 42 °C (). Vacuolization is an indicator of physical stress and a sign of autolysis and programmed cell death for some bacterial species [Citation39]. Thus, some apoptotic mechanisms might be activated by the effect of heat, which could facilitate bacterial killing (in combination with meropenem) in the presence of hyperthermia in our study. We think that further studies are needed to better understand the effects of heat/hyperthermia on bacterial PBP enzymes or bacterial apoptosis.
As a limitation of our study, we used Clinical and Laboratory Standards Institute (CLSI) criteria [Citation40] instead of EUCAST criteria for interpretation of the antibiogram profile of one drug (cefazolin) since there are no criteria for cefazolin in the EUCAST guidelines. Since we did not find a synergy between hyperthermia and some antibiotics tested, a better method would be to perform a synergy test on each cultivated bacterial isolate in order to select the strain that would benefit from this application. Secondly, testing the swarming behavior of bacteria on agar plates by heating for longer periods (20–24 h) may not reflect the clinical response to hyperthermia treatment which is applied for time periods up to 120 min. As another limitation, in vitro susceptibility tests may not always reflect the real effects of antibiotics, so it is necessary to plan novel in vivo studies to see the real effects of antibiotics at the temperature levels of hyperthermia.
Conclusions
The swarming phenomenon may be seen in different bacterial genera including Proteus, Pseudomonas and Salmonella and is accepted as an important virulence factor. It may be possible to treat such bacterial infections caused by swarming bacteria using thermal therapy devices and hyperthermia methods in the future. As a second conclusion, a temperature of 45 °C can be used to inhibit the swarming of the Proteus species to the whole surface of SBA plates during routine microbiological examination of samples in clinical microbiology laboratories. As we demonstrated in our study, antimicrobial drugs and hyperthermia could be used in combination to obtain synergistic effect on P. mirabilis bacteria.
Ethical approval
Ethical approval has been obtained from the Gaziantep University Clinical Researches Ethical Committee with date and number: 2021/05.
Consent for publication
All the authors have read the manuscript and have approved this submission.
Proofreading/editing
Proofreading was performed by Anchor English.
Acknowledgments
The authors thank Prof. Dr. Ebru Ballı and Prof. Dr. Banu Coşkun Yılmaz for technical assistance during TEM imaging.
Disclosure statement
No potential conflict of interest was reported by the author(s).
Additional information
Funding
References
- Yarmolenko PS, Moon EJ, Landon C, et al. Thresholds for thermal damage to normal tissues: an update. Int J Hyperthermia. 2011;27(4):320–343.
- Gazel D, Yılmaz M. Are infectious diseases and microbiology new fields for thermal therapy research? Int J Hyperthermia. 2018;34(7):918–924.
- Hajdu S, Holinka J, Reichmann S, et al. Increased temperature enhances the antimicrobial effects of daptomycin, vancomycin, tigecycline, fosfomycin, and cefamandole on staphylococcal biofilms. Antimicrob Agents Chemother. 2010;54(10):4078–4084.
- Ibelli T, Templeton S, Levi-Polyachenko N. Progress on utilizing hyperthermia for mitigating bacterial infections. Int J Hyperthermia. 2018;34(2):144–156.
- Borel N, Sauer-Durand AM, Hartel M, et al. wIRA: hyperthermia as a treatment option for intracellular bacteria, with special focus on Chlamydiae and Mycobacteria. Int J Hyperthermia. 2020;37(1):373–383.
- Harshey RM. Bacterial motility on a surface: many ways to a common goal. Annu Rev Microbiol. 2003;57:249–273.
- Harshey RM. Bees aren't the only ones: swarming in gram-negative bacteria. Mol Microbiol. 1994;13(3):389–394.
- Young GM, Smith MJ, Minnich SA, et al. The Yersinia enterocolitica motility master regulatory operon, flhDC, is required for flagellin production, swimming motility, and swarming motility. J Bacteriol. 1999;181(9):2823–2833.
- Déziel E, Lépine F, Milot S, et al. rhlA is required for the production of a novel biosurfactant promoting swarming motility in Pseudomonas aeruginosa: 3-(3-hydroxyalkanoyloxy)alkanoic acids (HAAs), the precursors of rhamnolipids. Microbiology (Reading)). 2003;149(Pt 8):2005–2013.
- Rather PN. Swarmer cell differentiation in Proteus mirabilis. Environ Microbiol. 2005;7(8):1065–1073.
- Kim W, Killam T, Sood V, et al. Swarm-cell differentiation in Salmonella enterica serovar typhimurium results in elevated resistance to multiple antibiotics. J Bacteriol. 2003;185(10):3111–3117.
- Girlich D, Bonnin RA, Dortet L, et al. Genetics of acquired antibiotic resistance genes in proteus spp. Front Microbiol. 2020;11:256
- Adeolu M, Alnajar S, Naushad S, et al. Genome-based phylogeny and taxonomy of the 'Enterobacteriales': proposal for Enterobacterales ord. nov. divided into the families Enterobacteriaceae, Erwiniaceae fam. nov., Pectobacteriaceae fam. nov., Yersiniaceae fam. nov., Hafniaceae fam. nov., Morganellaceae fam. nov., and Budviciaceae fam. nov. Int J Syst Evol Microbiol. 2016;66(12):5575–5599.
- Rauprich O, Matsushita M, Weijer CJ, et al. Periodic phenomena in Proteus mirabilis swarm colony development. J Bacteriol. 1996;178(22):6525–6538.
- Allison C, Hughes C. Closely linked genetic loci required for swarm cell differentiation and multicellular migration by Proteus mirabilis. Mol Microbiol. 1991;5(8):1975–1982.
- Allison C, Lai HC, Hughes C. Co-ordinate expression of virulence genes during swarm-cell differentiation and population migration of Proteus mirabilis. Mol Microbiol. 1992;6(12):1583–1591.
- Liaw SJ, Lai HC, Wang WB. Modulation of swarming and virulence by fatty acids through the RsbA protein in Proteus mirabilis [published correction appears in. Infect Immun. 2004;72(12):6836–6845.
- Schaffer JN, Pearson MM. Proteus mirabilis and urinary tract infections. Microbiol Spectr. 2015;3(5):10–1128.
- Bontron S, Poirel L, Kieffer N, et al. Increased resistance to carbapenems in proteus mirabilis mediated by amplification of the blaVIM-1-Carrying and IS26-Associated Class 1 Integron. Microb Drug Resist. 2019;25(5):663–667.
- Pagani L, Migliavacca R, Pallecchi L, et al. Emerging extended-spectrum beta-lactamases in Proteus mirabilis. J Clin Microbiol. 2002;40(4):1549–1552.
- The European Committee on Antimicrobial Susceptibility Testing. Breakpoint tables for interpretation of MICs and zone diameters. Version 11.0, 2021. http://www.eucast.org.
- Ling TK, Tam PC, Liu ZK, et al. Evaluation of VITEK 2 rapid identification and susceptibility testing system against gram-negative clinical isolates. J Clin Microbiol. 2001;39(8):2964–2966.
- Brink B. Urease test protocol. ASM MicrobeLibrary, USA (2010). https://www.asmscience.org/content/education/protocol/protocol.3223.
- Matuschek E, Brown DF, Kahlmeter G. Development of the EUCAST disk diffusion antimicrobial susceptibility testing method and its implementation in routine microbiology laboratories. Clin Microbiol Infect. 2014;20(4):O255–O266.
- Harmon RC, Rutherford RL, Wu HM, et al. Monoclonal antibody-mediated protection and neutralization of motility in experimental Proteus mirabilis infection. Infect Immun. 1989;57(7):1936–1941.
- Wang WB, Lai HC, Hsueh PR, et al. Inhibition of swarming and virulence factor expression in Proteus mirabilis by resveratrol. J Med Microbiol. 2006;55(Pt 10):1313–1321.
- Jones BV, Young R, Mahenthiralingam E, et al. Ultrastructure of Proteus mirabilis swarmer cell rafts and role of swarming in catheter-associated urinary tract infection. Infect Immun. 2004;72(7):3941–3950.
- Schild S, Tamayo R, Nelson EJ, et al. Genes induced late in infection increase fitness of Vibrio cholerae after release into the environment. Cell Host Microbe. 2007;2(4):264–277.
- O'Hara CM, Brenner FW, Miller JM. Classification, identification, and clinical significance of Proteus, Providencia, and Morganella. Clin Microbiol Rev. 2000;13(4):534–546.
- Jacobsen SM, Stickler DJ, Mobley HL, et al. Complicated catheter-associated urinary tract infections due to Escherichia coli and Proteus mirabilis. Clin Microbiol Rev. 2008;21(1):26–59.
- Ricker EB, Nuxoll E. Synergistic effects of heat and antibiotics on Pseudomonas aeruginosa biofilms. Biofouling. 2017;33(10):855–866.
- Pijls BG, Sanders IMJG, Kuijper EJ, et al. Synergy between induction heating, antibiotics, and N-acetylcysteine eradicates Staphylococcus aureus from biofilm. Int J Hyperthermia. 2020;37(1):130–136.
- Wu T, Wang L, Gong M, et al. Synergistic effects of nanoparticle heating and amoxicillin on H. pylori inhibition. J Magn Magn Mater. 2019;485:95–104. Vol
- Alumutairi L, Yu B, Filka M, et al. Mild magnetic nanoparticle hyperthermia enhances the susceptibility of Staphylococcus aureus biofilm to antibiotics. Int J Hyperthermia. 2020;37(1):66–75.
- Wardlow R, Bing C, VanOsdol J, et al. Targeted antibiotic delivery using low temperature-sensitive liposomes and magnetic resonance-guided high-intensity focused ultrasound hyperthermia. Int J Hyperthermia. 2016;32(3):254–264.
- Pérez-Núñez D, Briandet R, David B, et al. A new morphogenesis pathway in bacteria: unbalanced activity of cell wall synthesis machineries leads to coccus-to-rod transition and filamentation in ovococci. Mol Microbiol. 2011;79(3):759–771.
- Lewis JF, Bush K. 2015. Antibacterial Agents. In: Jorgensen JH, Pfaller MA, Carroll KC, Funke G, Landry ML, Richter SS, Warnock DW, editors. Manual of clinical microbiology. 11st ed. Washington: ASM Press, 1168–1211.
- Horii T, Kobayashi M, Sato K, et al. An in-vitro study of carbapenem-induced morphological changes and endotoxin release in clinical isolates of gram-negative bacilli. J Antimicrob Chemother. 1998;41(4):435–442.
- Rice KC, Bayles KW. Death's toolbox: examining the molecular components of bacterial programmed cell death. Mol Microbiol. 2003;50(3):729–738.
- Clinical and Laboratory Standards Institute Performance Standards for Antimicrobial Susceptibility Testing. M100 (30th edition), CLSI, Wayne, PA, USA 2020.