Abstract
Purpose
We aimed to determine the effects and possible mechanisms of hyperthermic intraperitoneal chemotherapy (HIPEC) in targeting ovarian cancer stem-like cells (CSCs).
Methods
Murine ovarian cancer cell lines presenting CSC surface markers were grown intraperitoneally in both immunocompetent and immunodeficient mice, which were then treated by intraperitoneal hyperthermia with the chemotherapeutic agents: paclitaxel and cisplatin. Tumor growth was measured by non-invasive luminescent imaging. Intraperitoneal immune cells, such as CD4+, CD8+ T cells, macrophages, and dendritic cells, were evaluated through flow cytometry analysis.
Results
Combined hyperthermia and chemotherapy exhibited an efficient therapeutic effect in the immunocompetent mice. However, a similar effect was not observed in the immunodeficient mice. Intraperitoneal hyperthermia increased the number of Intraperitoneal macrophages and dendritic cells that were lost due to chemotherapy. Compared with ovarian cancer bulk cells, CSCs were more susceptible to phagocytosis by macrophages.
Conclusion
We demonstrated that the superior therapeutic efficacy and reduced proportion of CSCs associated with intraperitoneal hyperthermic chemotherapy were immune-related. Hyperthermia recruits the phagocytes that target surviving CSCs after chemotherapy. These results provide a novel mechanism for the efficacy of HIPEC in treating ovarian cancer.
Introduction
The standard primary treatment for ovarian cancer, maximal cytoreductive surgery followed by chemotherapy, is associated with a high frequency of recurrence, development of chemotherapeutic drug resistance, and poor outcomes [Citation1]. Given this malignancy primarily spreads inside the peritoneal cavity, several intraperitoneal treatments have been developed [Citation2,Citation3]. Intraperitoneal hyperthermia (IP hyperthermia), especially when combined with chemotherapy, has demonstrated improved therapeutic efficacy for optimally (or even suboptimally) debulked ovarian cancer [Citation4–6]. Hypotheses on its working mechanisms include direct thermal cytotoxicity and reduced blood perfusion in cancerous lesions that cause an acidic, hypoxic, and nutritionally deprived microenvironment [Citation7,Citation8]. Hyperthermia has also been thought to increase tissue perfusion and tumor permeability through cytotoxic agents that cause thermal chemosensitization [Citation9,Citation10]. However, all cells possess powerful hyperthermia-triggered resistance mechanisms mediated by the induction of heat shock proteins (HSPs). HSPs are chaperones that rapidly repair protein thermal damage and lead to thermal resistance, (commonly referred to as thermotolerance) which in turn maintains cell survival [Citation11,Citation12]. The mechanisms by which HIPEC benefits cancer treatment, therefore, warrant further exploration. The tumor-initiating activity and relative resistance to chemotherapeutic drugs of cancer stem-like cells (CSCs) are well-established [Citation13–17]. Tumor recurrence is thought to be linked to the dormant CSCs that escape the annihilation of chemotherapy. HIPEC has been reported to significantly improve survival in patients with recurrent ovarian cancer [Citation18,Citation19]. Thus, the potential of HIPEC for targeting CSCs requires further investigation.
It is believed that tumor cells can adapt to the surrounding microenvironment and escape immune surveillance during cancer development [Citation20,Citation21]. Through tumor-associated macrophages suppressing the inflammation reaction and myeloid-derived suppressor cells inhibiting lymphocyte infiltration, tumor lesions become immune-privileged sites not controlled by host immune systems [Citation22–24]. Conventional chemotherapy is generally conducted using a maximal dose of cytotoxic agents that affect both cancer cells and normal (including immunosuppressive) cells. Intraperitoneal chemotherapies (IP chemotherapies) might exert a local cytotoxic effect by directly exposing tumors to cytotoxic agents. However, the slow release of drugs into the blood reduces systemic cytotoxicity. Hyperthermia could alter the tumor microenvironments by activating innate and adaptive immune-related cells, including natural killer cells, granulocytes, monocytes, and T cells [Citation25]. Hyperthermia also alleviates chemotherapy-induced neutropenia by increasing serum levels of neutrophil-recruiting cytokines [Citation26]. One study found that HIPEC affects tumors in surrounding microenvironments and that both residual progenitor and differentiated tumor cells interact with the immune system [Citation26]. In the present study, we employed an immunocompetent mouse ovarian cancer model to assess the therapeutic efficacy and possible mechanisms of IP hyperthermic chemotherapy in vivo. Our prior study had identified a mouse ovarian cancer ID8 cell line expressing stem cell antigen 1 (SCA1) that could be enriched through continuous culture in non-attached sphere status and exhibited CSC characteristics [Citation27]. Prior studies using xenograft human CSCs in immunodeficient animals did not represent general host conditions [Citation28–31]. By employing an immunocompetent mouse ovarian cancer model, we were able to explore the mechanisms by which HIPEC might aid in targeting CSCs. Alterations in peritoneal immune cell populations after either IP hyperthermia or HIPEC were assessed and analyzed.
Materials and methods
Mice and cell line
C57BL/6 and NOD/SCID mice were purchased from BioLASCO (Taiwan) and maintained under specific pathogen-free conditions. The murine ovarian carcinoma cell line ID8-luc (C57BL/6 origin) was cultured in RPMI 1640 medium (Gibco) supplemented with 10% fetal bovine serum (FBS; Biological Industrial, Israel), 100 U/mL penicillin (Gibco), and 100 pg/mL streptomycin (Gibco) in a humidified atmosphere of 5% CO2/95% air at 37 °C. SCA1+ ID8 cells were derived from the ID8-luc cells by continuous culture in ultralow attachment multiwell plates (Corning) containing RPMI 1640 medium (Gibco) supplemented with 0.4% methylcellulose (Sigma), 10% FBS (Biological Industrial), 100 U/mL penicillin (Gibco), and 100 pg/mL streptomycin (Gibco) to form cell spheres and were disrupted by 0.05% trypsin (Gibco) when passaged.
Ethical statement
This study followed the guidelines on experimental animal welfare and was approved by the IACUC of Mackay Memorial Hospital (MMH-A-S-97030).
IP hyperthermia and chemotherapy in a mouse model
To develop mouse ovarian tumor models, 3 × 105 of ID8-luc cells were intraperitoneally injected into both mouse strains (C57BL/6 and NOD/SCID). Although there is a strain difference between C57BL/6 and NOD/SCID mice, NOD/SCID is defective in both innate and adaptive immune systems. After ensuring and confirming that the ID8-luc tumor could grow well in NOD/SCID mice, we divided them into four groups: control (no treatment), IP hyperthermia, IP chemotherapy only, and combination treatment. On exploration at the indicated time, the mouse abdomen was opened under anesthesia with Zoletil (VIRBAC, France) and Rompun (Bayer Vital GmbH, Leverkusen, Germany). For the IP hyperthermia, 1× phosphate-buffered saline (PBS) was warmed at 45 °C and was continuously infused into the mouse peritoneal cavity. The heated PBS was refilled to replace cooled PBS at a frequency of 20–30 s/cycle for a total of 20 min. To maintain the PBS at a sufficient temperature when added to the mouse abdominal cavity, we infused the PBS at a slightly higher temperature (45 °C). The temperature of PBS inside the peritoneal cavity has been measured to be ∼42 °C employing this technique. In our prior study, 20 min of hyperthermia for mice has shown a therapeutic effect [Citation26]. In the combination therapy group, 10 mg/kg of Taxol (Bristol-Myers Squibb, Italy) and 6 mg/kg of cisplatin (ABIC, Israel) were administrated intraperitoneally immediately after the completion of hyperthermia treatment. The cavity was sutured closed immediately after completion of chemotherapy. The mice were kept warm under a heating light until they recovered from the anesthesia.
Non-invasive growth measurement of intraperitoneal tumors
ID8-luc tumor-bearing mice were intraperitoneally injected with 200 μL of 3.9 mg/mL luciferin; image acquisition commenced 10 min later. The mice were then anesthetized with isoflurane (Abbott) before imaging. The chemiluminescence intensity of each mouse was detected using the IVIS system (Xenogen) with fixed exposure conditions per batch experiment.
Effects of combined heat and chemotherapy on tumor cells in vitro
Initially, 2 × 105 ID8-bulk and SCA1+ ID8 cells were seeded into 6-cm dishes and cultured overnight. For heat treatment, the cells were incubated at 42 °C for 20 and 40 min, respectively. Unheated cells were used as controls. Different dosages of Taxol were added to the culture medium to attain a final concentration between 1 and 1000 nM. Cells were then kept cultured at 37 °C for 48 h. Cell viability was analyzed with the 3-(4,5-dimethylthiazol-2-yl)-2,5-diphenyl tetrazolium bromide (MTT) assay.
Intraperitoneal cell isolation
Six days after surgery, the mice were euthanized with CO2 and then intraperitoneally injected with 8 mL lavage fluid (1× cold PBS with 3% FBS). After the abdominal massage, the fluid was evacuated using a syringe. The abdominal cavities were opened with scissors, and the residual lavage fluid was collected by gentle micro pipetting. The washout cells were pelleted down by centrifugation, and the erythrocytes were removed using an ammonium-chloride-potassium lysing buffer (Invitrogen). To isolate the intraperitoneal macrophages, the collected cells were cultured in plastic culture plates for 45 min, and the unattached cells were washed out thrice with the culture medium by vigorous pipetting. The macrophages were finally detached using 1× PBS with 5% FBS and 0.25 mM ethylenediaminetetraacetic acid. Cell purity was determined by staining cells with anti-mouse CD11b and F4/80 antibodies conjugated with fluorescein isothiocyanate and allophycocyanin, respectively (eBioscience) and analyzed by using the FACSCalibur flow cytometer (BD Biosciences, San Jose, CA, USA).
Flow analysis
Antibodies against CD3, CD4, CD8, CD11b, CD11c, F4/80, CD45.2, and SCA1 (eBioscience) were employed. All cells were resuspended in a staining buffer composed of 1× PBS and 1% bovine serum albumin. One hundred microliters of cells were stained with antibodies in darkness for 30 min at 4 °C, at the dilution indicated by the supplier. The cells were then washed with 2 mL of staining buffer and centrifuged at 1600 rpm for 3 min before flow analysis. Flow cytometry was performed on a FACSCalibur flow cytometer for cell surface marker analysis, followed by result evaluation on an FCS Express software.
In vitro phagocytosis
Both the bulk and SCA1+ ID8 cells were stained with 100 µM carboxyfluorescein succinimidyl ester (CFSE; Invitrogen) at 37 °C for 10 min and washed thrice with the culture medium. Next, 1 × 105 of both stained cells were mixed with 5 × 105 purified intraperitoneal macrophages separately in a flow tube with 1 mL culture medium and incubated at 37 °C for 5 h. The cell mixture was then stained with the anti-mouse F4/80 antibody. The phagocytosis patterns of F4/80-positive macrophages in both cell types were analyzed using flow cytometry.
In vivo phagocytosis
Bulk ID8 cells were stained with 100 μM CFSE (Invitrogen) at 37 °C for 10 min and washed thrice with the culture medium. The SCA1+ ID8 cells were stained with CellVue dyes (Sigma) according to the manufacturer’s instructions. The Cells were then mixed in a 1:1 ratio and suspended in a 1× Hanks’ balanced salt solution (Invitrogen) at a concentration of 2 × 107 cells/mL. The C57BL/6 mice were then intraperitoneally injected with 200 µL of the mixed cells. One day later, cells inside the peritoneal cavity were washed out by lavage and the macrophages were purified in plastic culture plates as described previously. The phagocytosis patterns of the intraperitoneal macrophages were analyzed using flow cytometry.
Statistical analysis
All data are presented as means ± standard errors from at least two independent experiments. The Student’s t-test was used to compare differences between the data points.
Results
Chemotherapy plus IP hyperthermia exhibited better antitumor effects in vivo
Comparison of chemiluminescence intensities revealed that the tumor growth was inhibited by IP chemotherapy alone and this antitumor effect was further enhanced by combination with hyperthermia (; p = 0.0294, HIPEC vs. chemotherapy alone). However, the tumor size did not differ significantly between the hyperthermia and control groups (p = 0.3103). These results indicated that IP hyperthermia alone cannot effectively inhibit tumor growth, but it can nevertheless enhance the antitumor effect of IP chemotherapy.
Figure 1. Hyperthermia treatment enhanced the antitumor effect of IP chemotherapy and reduced the proportion of CSCs enriched by chemotherapy in immune-competent mice. 3 × 105 ID8-luc cells were intraperitoneally injected into C57BL/6 for tumor development. Three days later, either IP hyperthermia or hyperthermic IP chemotherapy was administered. IP lavage was performed 6 days post-treatment. The GFP+ cancer cells were gated, and the proportion of CSCs was analyzed by flow cytometry with anti-SCA1 fluorescent antibody staining. (A) Tumor growth images of mice with different treatments taken by IVIS. (B) The proportion of CSCs to tumor cells in peritoneal of C57BL/6 mice with different treatments. Error bars denote the standard errors (#p < 0.05; ***p < 0.0001).
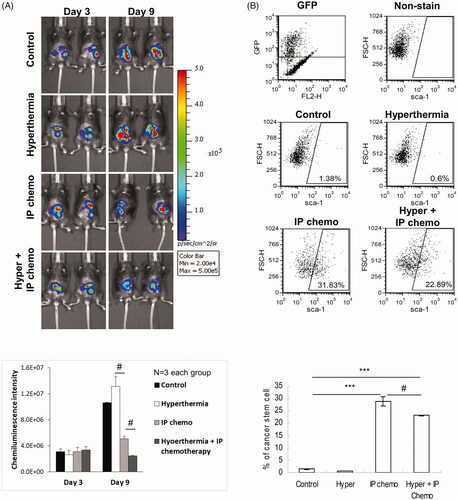
Hyperthermia reduced chemotherapy-induced increase in CSC proportion in vivo
CSCs have long been recognized as a drug-resistant cancer cell subpopulation. As previously mentioned, HIPEC led to better antitumor effects than chemotherapy alone. We determined whether the combination treatment works by targeting CSCs. The proportion of SCA1+ cells exhibiting CSC characteristics in the intraperitoneal cells was evaluated. presents the percentage variations in SCA1+ cells among green fluorescent protein–positive (GFP+) ID8 cells harvested through peritoneal lavage after each treatment. The proportion of SCA1+ CSCs was significantly higher in both the IP chemotherapy group and the HIPEC group than in the control group (p < 0.0001, control vs. IP chemotherapy; p < 0.0001, control vs. HIPEC). However, the SCA1+ proportion was significantly lower in the HIPEC group compared with the IP chemotherapy group (p = 0.0324). These results demonstrated that combining IP hyperthermia and chemotherapy facilitates CSC targeting.
Hyperthermia did not enhance the cytotoxic effects of chemotherapy on bulk or SCA1+ ID8 cells in vitro
To obtain CSCs, gradual enrichment of the SCA1+ ID8 cells was performed by repeated seeding of ID8 cells in ultralow attachment multiwell plates to allow cell sphere formation. Over 10 passages, almost all the ID8 cells became SCA1+ (). shows that different duration of heat treatment did not enhance the cytotoxicity of paclitaxel on either the bulk or SCA1+ ID8 cells.
Figure 2. Hyperthermia did not enhance the cytotoxic effect of chemotherapeutic drugs on bulk cells or CSCs in vitro. (A) SCA1+ ID8 cells were enriched through continuous sphere formation in RPMI 1640 medium supplemented with 0.4% methylcellulose. Proportions of SCA1+ cells at origin, passage 2 (p2), passage 4 (p4), and after passage 10 (>p10) were evaluated using flow cytometry analysis with fluorescent anti-mouse SCA1 antibody staining. (B) Bulk and SCA1+ ID8 cells incubated at 42 °C for 0, 20, and 40 min at different dosages of Taxol. After heat treatment, the cells were further incubated at 37 °C for 48 h. Cell viability was assessed using the MTT assay.
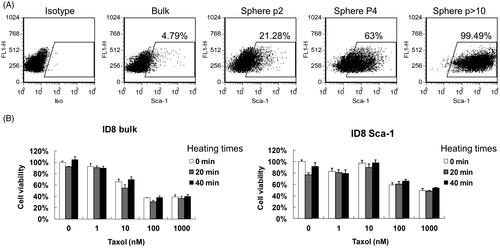
IP hyperthermia did not enhance the antitumor effects of chemotherapy in immunodeficient mice
ID8-luc cells were intraperitoneally injected into the NOD/SCID mice for tumor development. After 3 days, the abdominal cavities of the mice were opened, and either IP hyperthermia or HIPEC was administered. Seven days after treatment, no significant difference in antitumor effects was observed between the different treatments (). Flow cytometry analysis of the GFP+ cancer cells harvested from peritoneal lavage demonstrated that combination therapy did not reduce the proportion of SCA1+ CSCs enriched by chemotherapy in the immunodeficient mice (). The results suggest that the host immunity is likely involved with the enhanced antitumor effect and the reduction of the CSCs proportion is associated with the combination therapy.
Figure 3. HIPEC did not further inhibit tumor growth or reduce CSC proportions than IP chemotherapy alone in immunodeficient mice. 2 × 105 ID8-luc cells were first intraperitoneally injected into NOD/SCID mice. Three days later, either IP hyperthermia or hyperthermic IP chemotherapy was administered. IP lavage was performed 7 days post-treatment. The GFP+ cancer cells were gated, and the proportion of CSCs was analyzed using flow cytometry with anti-SCA1 fluorescent antibody staining. (A) Tumor growth images of mice with different treatments taken by IVIS. (B) The proportion of CSCs to tumor cells in the peritoneum of NOD/SCID mice with different treatments. Error bars denote the standard errors (#p < 0.05; ***p < 0.0001) n.s., not significant.
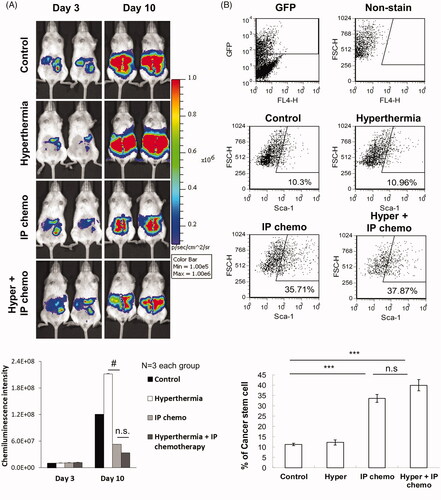
Innate immune cells in the peritoneal cavity were increased by IP hyperthermia
Analysis of the peritoneal lavaged cells 3 days after IP hyperthermia treatment revealed significant increases in dendritic cells and macrophages (p = 0.0047 IP hyperthermia vs. control for dendritic cells; p = 0.0098, IP hyperthermia vs. control for macrophages), but not in T cells (). Hyperthermia also restored the number of dendritic cells and macrophages in the abdominal cavity that were eliminated by intraperitoneal paclitaxel/cisplatin treatment (p = 0.0435 for combination treatment vs. IP chemotherapy for dendritic cells; p = 0.033 for combination treatment vs. IP chemotherapy for macrophages). These results have shown an association between enhanced antitumor effects and hyperthermia-induced increases of innate immune phagocytes.
Figure 4. Analysis of peritoneal exudate cell population change after IP hyperthermia or HIPEC. C57BL/6 mice underwent either IP hyperthermia or hyperthermic IP chemotherapy. Three days later, the peritoneal exudate cells were collected by IP lavage with 8 ml of lavage fluid. Flow cytometry was used to determine cell numbers and cell types. IP chemotherapy reduced numbers of all cell types, demonstrating inhibited host immunity. Hyperthermia increased numbers of macrophages and dendritic cells but not CD4+ and CD8+ T cells. This effect attenuated the cytotoxic effect of IP chemotherapy on these two cell types. N = 4 for each group. Error bars denote the standard errors. (#p < 0.05; *p < 0.01).
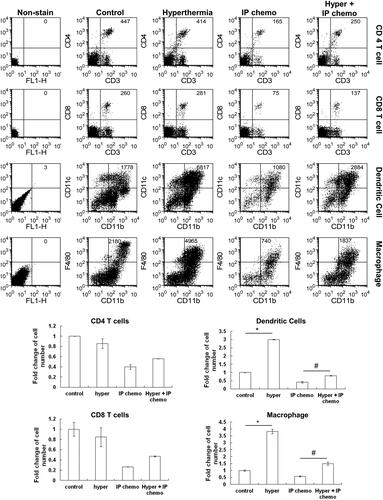
CSCs were targeted by macrophage phagocytosis both in vitro and in vivo
We further investigated whether the reduction in the number of CSCs in combination therapy and the increase in innate immune phagocytes in IP hyperthermia were correlated. Coculture of the lavaged peritoneal F4/80+ macrophages obtained from the C57BL/6 mice with CFSE-stained bulk or SCA1+ ID8 cells demonstrated that macrophages engulfed SCA1+ ID8 cells most in vitro (). To determine whether this result occurred in vivo, we stained bulk and SCA1+ ID8 cells with 2 dyes (CFSE and CellVue, respectively) for viable cell identification () and intraperitoneally injected them into the C57BL/6 mice. Flow cytometry analysis revealed that the isolated macrophages engulfed SCA1+ ID8 cells more than the bulk ID8 cells (). These results indicate that intraperitoneal macrophages induced by hyperthermia treatment specifically targeted CSCs and contributed to a better antitumor effect.
Figure 5. Macrophage phagocytosis of bulk cells and CSCs in vitro. Macrophages were isolated from the peritoneal cavity of C57BL/6 mice by IP lavage and then purified by plastic culture plate attachment. The F4/80-positive population was gated during flow cytometry analysis. For the phagocytosis assay, the bulk and SCA1+ ID8 cells were stained with CFSE and cocultured with the purified macrophages in a 1:5 ratio at 37 °C for 5 h. The efficiency of macrophage phagocytosis of each cell type was evaluated through flow cytometry. Error bars denote the standard errors. (#p < 0.05).
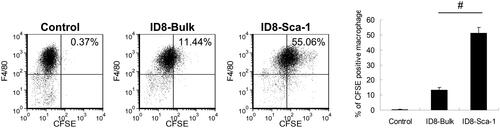
Figure 6. Macrophages preferentially phagocytose CSCs compared with bulk tumor cells in vivo. To distinguish which cells were phagocytosed in vivo, ID8-bulk cells were stained with CFSE and ID8 SCA1+ cells were stained with CellVue. After that, the same number of two types of cells were mixed and intraperitoneally injected into mice. (A) Flow cytometry analysis of fluorescence-labeled bulk and SCA1+ cells. (B) Purity of macrophages harvested from peritoneal lavage 1 day after intraperitoneal injection of fluorescence-labeled cancer cells and isolated by plastic culture dish attachment. (C) Flow cytometry analysis of the susceptibility of both cells to phagocytosis of macrophages in peritoneal exudates. The percentages of macrophages that were positive for different fluorescence represented the phagocytosed cell types. Error bars denote the standard errors.
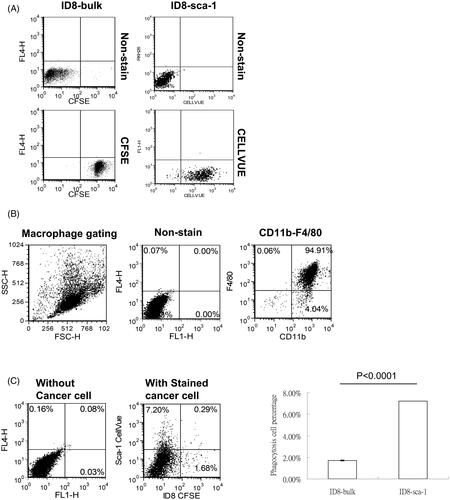
Discussion
According to the tumor immunosurveillance theory, differences in antigen expression between normal and tumor cells can aid host immune systems in recognizing and eliminating tumors [Citation24,Citation32]. The carcinogenesis process involves alterations in the tumor microenvironment or differentiation of immunogenic cells into non-immunogenic offspring. Developing modalities that use the protective effect of the tumor microenvironment could enhance the effects of cancer therapy. IP hyperthermia alone cannot effectively destroy cancer cells, although it enhanced antitumor effects and reduced the proportions of CSCs enriched by paclitaxel/cisplatin treatment in immunocompetent mice. However, a similar effect was neither observed in immunodeficient mice nor in vitro. The enhanced antitumor effect demonstrated by the combination treatment does not apply directly to the cancer cells themselves; rather, this phenomenon might be a result of interacting with the microenvironment. In the immunodeficient model, hyperthermia did not reduce the proportion of CSCs enriched by chemotherapy, implying that the enhanced therapeutic effects of the combination treatment might be associated with immune responses.
Chemotherapy kills not only cancer cells but also normal cells, including immune cells [Citation33]. Local chemotherapy, such as IP chemotherapy, can destroy intraperitoneal cancer cells, stromal cells, and immune cells. There is no severe hematological or non-hematological toxicity or neurotoxicity noted in this kind of treatment [Citation34]. The tumor microenvironment usually changes greatly under local chemotherapy; however, the CSCs might survive through developing drug resistance. The immune system sustains comparatively less damage from IP chemotherapy. Our prior results indicate that CSCs are susceptible to macrophage phagocytosis [Citation27]. In our model, IP hyperthermia restored many macrophages and dendritic cells to the peritoneal cavity that compensated for those eliminated by IP chemotherapy. Given hyperthermia has been recognized as a modality related to immune therapy, HSP induction could promote tumor antigen presentation and elicit tumor-specific immune responses [Citation35–37]. However, hyperthermia treatment alone did not exhibit therapeutic benefits in the present cancer model. We speculate that without eradication of cells around the tumor microenvironment, such as tumor-associated fibroblasts, myeloid-derived suppressor cells, and regulatory T cells, hyperthermia-enriched immune cells cannot infiltrate tumor lesions to attack cancer cells. In a study on breast cancer, the overexpression of HSPs, especially HSP90, was found in heat-treated CSCs and caused increasing thermal resistance; inhibition of HSP90 by the specific inhibitor could partially sensitize the CSCs to hyperthermia [Citation38]. This finding also explains why hyperthermia treatment alone is not therapeutically effective for cancer [Citation39].
The purpose of contemporary HIPEC is to facilitate tumor vasculature permeability and the penetration of chemotherapeutic agents to enhance cytotoxic effects against tumor cells. In this study, HIPEC was not feasible in real practice due to the lack of such a device for mice. Alternatively, we administered IP chemotherapy just after hyperthermia. According to a prior study, the tumor vasculature remains permeable at least for 8 h after hyperthermia [Citation40]. One other report had stated that thermosensitive liposomal drugs could be applied 20–30 min after the start of hyperthermia [Citation41]. We suspect our technique of performing hyperthermia followed by IP chemotherapy has a similar therapeutic effect with HIPEC. Why CSCs are more susceptible to macrophage phagocytosis than their differentiated counterparts is not entirely clear. It could be due to differences in the expression levels of cell surface proteins or cytokines. Some cell surface proteins, such as CD47 and calreticulin, have been reported to send ‘eat me’ and ‘don’t eat me’ signals to macrophages [Citation42,Citation43]. Our previous studies demonstrated that lower CD47 expression of ovarian CSCs might induce susceptibility to macrophage phagocytosis and they can be protected by surrounding bulk cells [Citation27]. Additional studies investigating the underlying mechanisms that balance the tumor bulk cells and CSCs are warranted.
In this study, we used a mouse ovarian cancer model to evaluate the effect of hyperthermic IP chemotherapy on tumor immune microenvironments in the peritoneal cavity. In hyperthermic IP chemotherapy, hyperthermia not only reduced CSCs that were enriched by chemotherapy but also recruited macrophages and dendritic cells to the tumor site and targeted the relatively drug-resistant CSCs that had survived the chemotherapy. The enhanced therapeutic efficacy of combination therapy was immune-dependent. Our study has disclosed a possible mechanism in hyperthermic IP chemotherapy: HIPEC might facilitate the development of an adaptive immune response that preferentially targets ovarian cancer stem cells. It is generally believed that recurrent ovarian cancer develops from dormant cancer stem cells that survived chemotherapy. HIPEC might play a role in minimal residual disease after adjuvant chemotherapy or in recurrent disease [Citation44]. In addition, the administration of immune checkpoints or other immune potentiating agents after HIPEC might be a reasonable approach to enhance the treatment efficacy for ovarian cancer [Citation45].
Disclosure statement
No potential conflict of interest was reported by the author(s).
Additional information
Funding
References
- Kim A, Ueda Y, Naka T, et al. Therapeutic strategies in epithelial ovarian cancer. J Exp Clin Cancer Res. 2012;31:14.
- Lu Z, Wang J, Wientjes MG, et al. Intraperitoneal therapy for peritoneal cancer. Future Oncol. 2010;6(10):1625–1641.
- Jaaback K, Johnson N, Lawrie TA. Intraperitoneal chemotherapy for the initial management of primary epithelial ovarian cancer. Cochrane Database Syst Rev. 2016;(1):CD005340.
- Helm CW. The role of hyperthermic intraperitoneal chemotherapy (HIPEC) in ovarian cancer. Oncologist. 2009;14(7):683–694.
- Ansaloni L, Agnoletti V, Amadori A, et al. Evaluation of extensive cytoreductive surgery and hyperthermic intraperitoneal chemotherapy (HIPEC) in patients with advanced epithelial ovarian cancer. Int J Gynecol Cancer. 2012;22(5):778–785.
- Van Driel WJ, Koole SN, Sikorska K, et al. Hyperthermic intraperitoneal chemotherapy in ovarian cancer. N Engl J Med. 2018;378(3):230–240.
- Song CW. Effect of local hyperthermia on blood flow and microenvironment: a review. Cancer Res. 1984;44(10 Suppl):4721s–4730s.
- Engin K. Biological rationale for hyperthermia in cancer treatment (II). Neoplasma. 1994;41(5):277–283.
- de Bree E, Tsiftsis DD. Principles of perioperative intraperitoneal chemotherapy for peritoneal carcinomatosis. Recent Results Cancer Res. 2007;169:39–51.
- Rettenmaier MA, Mendivil AA, Gray CM, et al. Intra-abdominal temperature distribution during consolidation hyperthermic intraperitoneal chemotherapy with carboplatin in the treatment of advanced stage ovarian carcinoma. Int J Hyperthermia. 2015;31(4):396–402.
- Li GC, Mivechi NF, Weitzel G. Heat shock proteins, thermotolerance, and their relevance to clinical hyperthermia. Int J Hyperthermia. 1995;11(4):459–488.
- Rylander MN, Feng Y, Bass J, et al. Thermally induced injury and heat-shock protein expression in cells and tissues. Ann N Y Acad Sci. 2005;1066:222–242.
- Dean M, Fojo T, Bates S. Tumour stem cells and drug resistance. Nat Rev Cancer. 2005;5(4):275–284.
- Steg AD, Bevis KS, Katre AA, et al. Stem cell pathways contribute to clinical chemoresistance in ovarian cancer. Clin Cancer Res. 2012;18(3):869–881.
- Ma S, Lee TK, Zheng BJ, et al. CD133+ HCC cancer stem cells confer chemoresistance by preferential expression of the Akt/PKB survival pathway. Oncogene. 2008;27(12):1749–1758.
- Ishikawa F, Yoshida S, Saito Y, et al. Chemotherapy-resistant human AML stem cells home to and engraft within the bone-marrow endosteal region. Nat Biotechnol. 2007;25(11):1315–1321.
- Hermann PC, Huber SL, Herrler T, et al. Distinct populations of cancer stem cells determine tumor growth and metastatic activity in human pancreatic cancer. Cell Stem Cell. 2007;1(3):313–323.
- Fagotti A, Costantini B, Vizzielli G, et al. HIPEC in recurrent ovarian cancer patients: morbidity-related treatment and long-term analysis of clinical outcome. Gynecol Oncol. 2011;122(2):221–225.
- Costales AB, Chambers L, Chichura A, et al. Effect of platinum sensitivity on the efficacy of hyperthermic intraperitoneal chemotherapy (HIPEC) in recurrent epithelial ovarian cancer. J Gynecol Obstet Hum Reprod. 2021;50(5):101844.
- Chew V, Toh HC, Abastado JP. Immune microenvironment in tumor progression: characteristics and challenges for therapy. J Oncol. 2012;2012:608406.
- Liu Y, Cao X. Immunosuppressive cells in tumor immune escape and metastasis. J Mol Med. 2016;94(5):509–522.
- Mantovani A, Sica A. Macrophages, innate immunity and cancer: balance, tolerance, and diversity. Curr Opin Immunol. 2010;22(2):231–237.
- Hart KM, Byrne KT, Molloy MJ, et al. IL-10 immunomodulation of myeloid cells regulates a murine model of ovarian cancer. Front Immunol. 2011;2:29.
- Veglia F, Perego M, Gabrilovich D. Myeloid-derived suppressor cells coming of age. Nat Immunol. 2018;19(2):108–119.
- Frey B, Weiss EM, Rubner Y, et al. Old and new facts about hyperthermia-induced modulations of the immune system. Int J Hyperthermia. 2012;28(6):528–542.
- Huang W-C, Wu C-C, Hsu Y-T, et al. Effect of hyperthermia on improving neutrophil restoration after intraperitoneal chemotherapy. Int J Hyperthermia. 2019;36(1):1254–1262.
- Chang C-L, Wu C-C, Hsu Y-T, et al. Immune vulnerability of ovarian cancer stem-like cells due to low CD47 expression is protected by surrounding bulk tumor cells. Oncoimmunology. 2020;9(1):1803530.
- Curley MD, Therrien VA, Cummings CL, Sergent PA, et al. CD133 expression defines a tumor initiating cell population in primary human ovarian cancer. Stem Cells. 2009;27(12):2875–2883.
- Chiba T, Kita K, Zheng YW, et al. Side population purified from hepatocellular carcinoma cells harbors cancer stem cell-like properties. Hepatology. 2006;44(1):240–251.
- Kumar D, Kumar S, Gorain M, et al. Notch1-MAPK signaling axis regulates CD133+ cancer stem cell-mediated melanoma growth and angiogenesis. J Invest Dermatol. 2016;136(12):2462–2474.
- Xiang D, Shigdar S, Bean AG, et al. Transforming doxorubicin into a cancer stem cell killer via EpCAM aptamer-mediated delivery. Theranostics. 2017;7(17):4071–4086.
- Marigo I, Dolcetti L, Serafini P, et al. Tumor-induced tolerance and immune suppression by myeloid derived suppressor cells. Immunol Rev. 2008;222:162–179.
- Yoshimatsu K, Kuhara K, Itagaki H, et al. Changes of immunological parameters reflect quality of life-related toxicity during chemotherapy in patients with advanced colorectal cancer. Anticancer Res. 2008;28(1B):373–378.
- Guardiola E, Delroeux D, Heyd B, et al. Pivot X: Intra-operative intra-peritoneal chemotherapy with cisplatin in patients with peritoneal carcinomatosis of ovarian cancer. World J Surg Oncol. 2009;7:14.
- Murshid A, Gong J, Calderwood SK. The role of heat shock proteins in antigen cross presentation. Front Immunol. 2012;3:63.
- Calderwood SK, Stevenson MA, Murshid A. Heat shock proteins, autoimmunity, and cancer treatment. Autoimmune Dis. 2012;2012:486069.
- Zhou YJ, Binder RJ. The heat shock protein-CD91 pathway mediates tumor immunosurveillance. Oncoimmunology. 2014;3(4):e28222.
- Burke AR, Singh RN, Carroll DL, et al. The resistance of breast cancer stem cells to conventional hyperthermia and their sensitivity to nanoparticle-mediated photothermal therapy. Biomaterials. 2012;33(10):2961–2970.
- Sticca RP, Dach BW. Rationale for hyperthermia with intraoperative intraperitoneal chemotherapy agents. Surg Oncol Clin N Am. 2003;12(3):689–701.
- Li L, ten Hagen TL, Bolkestein M, et al. Improved intratumoral nanoparticle extravasation and penetration by mild hyperthermia. J Control Release. 2013;167(2):130–137.
- van Rhoon GC, Franckena M, Ten Hagen TLM. A moderate thermal dose is sufficient for effective free and TSL based thermochemotherapy. Adv Drug Deliv Rev. 2020;163–164:145–156.
- Chao MP, Alizadeh AA, Tang C, et al. Anti-CD47 antibody synergizes with rituximab to promote phagocytosis and eradicate non-Hodgkin lymphoma. Cell. 2010;142(5):699–713.
- Chao MP, Jaiswal S, Weissman-Tsukamoto R, et al. Calreticulin is the dominant pro-phagocytic signal on multiple human cancers and is counterbalanced by CD47. Sci Transl Med. 2010;2(63):63ra94.
- Boussios S, Sadauskaite A, Kanellos FS, Tsiouris AK, et al. Neoadjuvant, HIPEC and maintenance treatment in ovarian and peritoneal serous cancer: current status. Gynecol Pelvic Med. 2020;3:19–19.
- Yagawa Y, Tanigawa K, Kobayashi Y, et al. Cancer immunity and therapy using hyperthermia with immunotherapy, radiotherapy, chemotherapy, and surgery. JCMT. 2017;3(10):218–230.