Abstract
Purpose
To retrospectively evaluate the efficacy and safety of computed tomography (CT)-guided microwave ablation (MWA) combined with percutaneous vertebroplasty (PVP) as a treatment for painful high thoracic vertebral metastases (T1–T4).
Materials and Methods
In this retrospective study, 23 adult patients (33 high thoracic vertebral metastases) with moderate to severe pain were treated with CT-guided MWA and PVP. The procedural effectiveness was evaluated using a Visual Analog Scale (VAS), daily morphine consumption, and the Oswestry Disability Index (ODI) before and immediately after the procedure and during follow-up.
Results
Technical success was achieved in all patients. The mean pre-procedure VAS score and morphine doses were 6.7 ± 1.7 (5–10) and 105.2 ± 32.7 (30–150) mg, respectively. The mean VAS scores and daily morphine doses at 24 h and 1, 4, 12, and 24 weeks post-operatively were 3.2 ± 1.4 and 41.3 ± 9.6 mg; 1.8 ± 1.0 and 31.5 ± 12.2 mg; 1.4 ± 1.3 and 19.6 ± 12.4 mg; 1.1 ± 0.8 and 14.5 ± 9.6 mg; and 1.0 ± 0.7 and 13.9 ± 9.3 mg, respectively (all p < 0.001). ODI scores significantly decreased (p < 0.05). Minor cement leakage occurred in 10 patients (30.30%) with no symptoms. Follow-up imaging showed no local tumor progression.
Conclusions
Preliminary results suggest MWA combined with PVP is an effective and safe treatment for painful high thoracic vertebral metastases (T1–T4) and can significantly relieve pain and improve the quality of life of patients. However, its efficacy should be confirmed by mid- and long-term studies.
Introduction
Spinal metastases are a significant source of morbidity in patients with systemic cancer. Cadaver studies have shown that spinal metastases occur in 30–90% of patients with terminal cancer [Citation1]. Spinal metastases cause progressive and persistent pain as well as other complications, including spinal fractures, nerve compression, and spinal cord compression. Tumor cells stimulate osteoblasts and osteolytic growth factors, resulting in bone destruction, local cancer cell proliferation, periosteal nerve ending stimulation, infiltration, and compression of nerves and surrounding tissues, pathological fractures, and persistent pain. Vertebral tumors cause spinal cord compression in >10% of patients [Citation2]. Therefore, the clinical goals of spinal metastasis treatment are to relieve pain and protect neurological function.
The traditional treatments for spinal metastases include surgery, bisphosphonate administration, and radiotherapy. Historically, radiotherapy has been the standard treatment for painful spinal metastases [Citation3–5]; however, ∼40% of treated patients do not experience adequate pain relief, and ∼50% have recurrent pain at a median of 16 weeks following treatment [Citation6,Citation7]. In the past two decades, image-guided interventional techniques have emerged, producing satisfactory outcomes for spinal neoplasm management. These techniques include cementoplasty [Citation2–4,Citation7], radiofrequency (RF) ablation [Citation8], combined RF ablation and cementoplasty [Citation5,Citation9], and cryotherapy ablation [Citation10]. More recently, percutaneous microwave ablation (MWA) has been used to treat various types of malignant tumors [Citation11].
Upper thoracic vertebroplasty may be technically different from that at the levels of lumbar and lower thoracic spines. The width of the thoracic pedicle isthmus is significantly narrower than that of the lumbar vertebrae. The average transverse diameter of the pedicle at the T1 vertebral body is 6.4 mm in women and 7.3 mm in men. At T3, however, the mean diameter is only 3.4 mm in women and 3.9 mm in men [Citation7]. The upper thoracic vertebrae have short and narrow pedicles shaped like a heart (back to front), and the thoracic vertebral arch encloses a small, round spinal canal. Thus, the percutaneous transpedicular approach to the upper thoracic vertebrae is technically challenging and even a small amount of cement leakage can cause serious neurological complications [Citation7]. However, there are few reports regarding treatment for high thoracic vertebral metastasis. Therefore, this study analyzed the efficacy and safety of computed tomography (CT)-guided MWA combined with percutaneous vertebroplasty (PVP) in treating painful high thoracic vertebral metastases (T1–T4).
Materials and methods
Case data
We retrospectively analyzed 23 patients with high thoracic vertebral metastases treated with CT-guided MWA combined with PVP in our hospital from January 2015 to June 2020. The retrospective research was approved by the Ethics Committee of Tengzhou Central People’s Hospital (approval no. 2020-Ethics Review-12). Before undergoing MWA combined with PVP surgery, all patients provided written informed consent. All patients underwent CT or magnetic resonance imaging (MRI) for the evaluation of the location, size, and nature of the lesions.
Patients
The inclusion criteria for patients were as follows: target lesion was a pathologically confirmed vertebral metastatic tumor; the tumor was localized at the high thoracic vertebrae (T1–T4); target lesion had corresponding focal and persistent pain; VAS score >4; the presence of persistent or recurrent pain after radiotherapy, or ineligibility for radiotherapy; and an estimated life expectancy of at least 3 months.
The exclusion criteria were as follows: uncorrectable coagulation dysfunction (platelet count: 50 × 109/L, international standardized ratio: 1.50); infection around the focus or uncontrolled systemic infection; tumors with retropulsion of the posterior cortices, epidural extension, or cord compression; patients with tumors whose margins approximated the nerve roots or the spinal canal; and Eastern Cooperative Oncology Group Performance Status score ≥3.
Instruments
The following instruments were used: ECO-2450B MWA system (ECO Microwave Electronic Institute, Nanjing, China. Registration standard: YZB/country 1475–2013. China: SFDA certified No.: [III] 20112251456); Vison-China Medical Devices Research & Development Center, Nanjing, China. Registration standard: YZB/country 4843-2012, No: SFDA (III) 20122250773; polymethylmethacrylate (Palacos®; Heraeus Medical GmbH, Hanau, Germany); and CT: SOMATOM Definition AS (Siemens, Munich, Germany).
Treatment
The operative characteristics are shown in . All operations were performed by the same chief physician with >10 years of experience in ablation treatment. An interdisciplinary team of radiologists, pain physicians, surgeons, and oncologists evaluated all patients before surgery.
Table 1. Baseline characteristics of study patients.
Preoperative preparation
MRI and contrast-enhanced CT of the target lesion were performed preoperatively to determine the location, size, and boundary of the lesion; to determine whether the posterior edge of the vertebral body was intact or not, and to formulate the ablation plan.
Operative procedure
The appropriate operative position was chosen based on the lesion (to maintain a fixed position) and the patient's comfort. The patients adopted a prone position and were fixed with a vacuum negative-pressure pad. The patients received a continuous intravenous infusion of fentanyl citrate (0.1 mg/2 ml diluted 1:10 with saline solution) and were maintained under conscious sedation.
The optimal needle puncture site and angle were selected according to the CT scan. The unilateral costal joint puncture was performed in 15 cases and the bilateral costal joint puncture in eight cases. Ropivacaine was used for local anesthesia. A 22-G × 15-cm bone puncture needle was used for layer-by-layer lidocaine injection. Scanning continued during the puncture with rapid three-dimensional reconstruction to adjust the direction and angle. A 0.32 × 13-cm bone puncture needle (Shandong Guanlong Medical Utensils Co., Ltd, Shandong Province, China) was placed at the anterior edge of the long axis of the lesion. If there was a compression fracture, the bone puncture needle was placed at the center of the compressed vertebral body. A 0.16 × 20-cm antenna was coaxially inserted into the tumor. The retreating puncture needle caused the front end of the microwave antenna to be exposed by ∼1.5 cm. During the operation, an ablation power of 20–30 W and ablation time of 3–5 min were selected according to the location, size, and adjacent tissue of the lesion. If the focus was close to the anterior edge of the vertebral body and the posterior edge of the vertebral body was intact, we used an ablation power of 30 W and time of 5 min; if the focus was close to the posterior edge of the vertebral body and the posterior edge of the vertebral body was incomplete, we used an ablation power of 20 W and time of 3 min. Short repeated microwave cycles (30–60 s) were performed. Lower-limb clinical examinations (motor and sensory) were conducted between MWA sessions, and the patients were instructed to alert the surgical team of the presence of lower-limb pain or paresthesia.
After ablation, the ablation antenna was withdrawn, and the bone puncture needle was pushed forward in the appropriate position. The puncture needle was accurately placed across the midline and in front of the vertebral body while the bone cement paste was prepared. We used Polymethylmethacrylate (PMMA) cement (Haraeus Medical GmbH, Wehrheim, Germany), which is highly viscous in nature. Due to viscosity, several 1-ml syringes were used to extract the bone cement, which was kept in ice-cold physiological saline to prolong the solidification time of the bone cement paste. Next, the PMMA cement was injected into the ablation focus slowly, intermittently, and in small quantities, with no more than 0.5 ml per injection. After each injection, CT scanning was performed to observe the degree of filling and the direction of flow of the bone cement. When the bone cement was nearing the posterior edge of the vertebral body, no more than 0.3 ml was used per injection. The amount of bone cement injected differed according to the size of the tumor and the injection site. If the bone cement was found leaking into the spinal canal or intervertebral foramen, the needle was immediately withdrawn. A final CT examination was performed to ensure that the lesion was sufficiently filled with cement and to determine the presence or absence of cement leakage (). Technical success was defined as the successful implantation of the microwave antenna into the center of the target bone, with the ability to completely ablate the target area, as well as the successful injection of the bone cement, filling 2/3 of the volume at the location of the lesion.
Figure 1. A 62-year-old man with painful osteolytic T3 metastases from lung adenocarcinoma was treated with microwave ablation combined with bone cementing. Preoperative sagittal MRI (A), axial MRI (B), and axial CT (C) revealed osteolytic T3 metastases with the complete destruction of the posterior vertebral wall, tumor compression of the dural sac, and severe compression fracture. Using the left costal joint approach, a microwave antenna (arrow) was guided to the anterior edge of the lesion (D) by a bone puncture needle (arrow). After microwave ablation, 3 ml of bone cement was injected slowly (E), and the sagittal CT showed that the bone cement was deposited in the lesion area without obvious leakage (F). CT: computed tomography; MRI: magnetic resonance imaging.
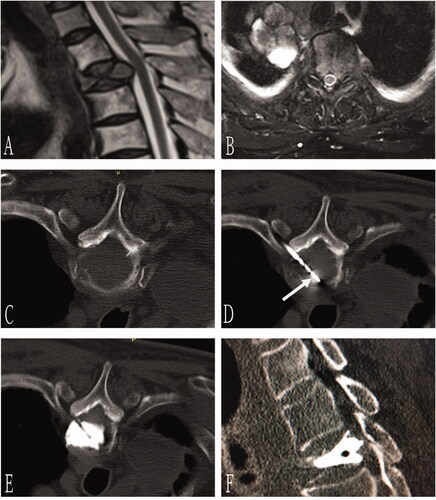
Figure 2. A 70-year-old man with T3 osteolytic metastases from lung adenocarcinoma was treated with microwave ablation combined with bone cement plasty. Preoperative axial CT showed T3 osteolytic destruction (A). The needle was inserted through bilateral costal vertebra joints, and the tip of the bone puncture needle was located in the middle 1/3 of the anterior vertebra (B). Next, the microwave antenna (arrow) was inserted through the right coaxial bone puncture needle (C), and 3 ml of bone cement was injected after microwave ablation (D). Sagittal (E) and coronal 3D CT (F) showed well-deposited bone cement in the vertebral body without extravasation.
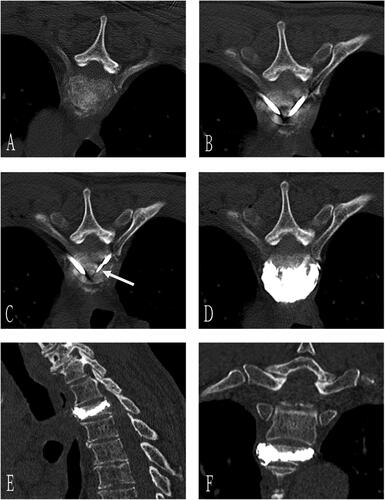
Follow-up
The primary goal of treatment was rapid pain relief due to the short life expectancy of the patients; therefore, the clinical follow-up period for this study was 6 months. Patients were asked to use VAS scores to measure their pain on a scale of 0–10 (where 10 represents the strongest pain ever experienced and 0 indicates no pain) before surgery and 24 h, 1 week, 4 weeks, 3 months, and 6 months after surgery [Citation12]. Daily opioid consumption was calculated according to the morphine sulfate equivalent used and we followed the National Comprehensive Cancer Network Clinical Practice Guidelines in Oncology for Adult Cancer Pain. The degree of disability was assessed using the Oswestry Disability Index (ODI) [Citation13], which includes categories assessing pain, self-care, lifting, walking, sitting, standing, sleep disturbance, sex life, and the intensity of travel. Contrast-enhanced CT and MRI scans were performed 1 month after treatment and then every 3 months and compared with the post-procedural CT scans to identify local tumor progression. Local tumor progression was defined as an osteolytic defect of the tumor or growth of soft tissue components.
Statistical methods
SPSS version 17.0 (IBM Corp., Armonk, NY, USA) was used for statistical analysis. Continuous variables are expressed as means ± standard deviations. VAS scores and opioid dosages before and after the operation were measured using a bilateral t-test, in which p < 0.05 was considered statistically significant.
Results
Twenty-three patients (33 high vertebral metastases) treated with CT-guided MWA with PVP were included in this study. Of the 23 patients, 11 were male and 12 were female, with an average age of 64.35 ± 8.28 (49–81) years. Eleven cases exhibited destruction of the posterior edge of the vertebral body and seven cases were complicated with a compression fracture. The general condition of the patients, vertebral body distribution, nature of bone destruction, and histology of the primary malignant tumors are shown in .
The technical success rate of all cases was 100%. Operative characteristics are shown in . The unilateral costal joint puncture was performed in 21 cases and the bilateral costal joint puncture in 12 cases. The average amount of bone cement injected into each lesion was 2.9 ± 1.1 ml (1.0–6.0 ml). In all cases, CT volumetric scanning was performed during the operation to determine complications, such as bleeding and bone cement leakage. Of the 33 lesions, bone cement leakage occurred in 10 lesions (30.30%), including intervertebral disk cement leakage in six and paravertebral cement leakage in four; none of which had clinical symptoms. Among the cases of bone cement leakage, the posterior edge was intact in six cases and incomplete in four cases.
Table 2. Operative characteristics.
Post-operatively, the VAS scores ranged from 5.0 to 10.0, with an average of 6.7 ± 1.7. The pain was relieved as early as 1 h after treatment, and the mean VAS score at 24 h after surgery was 3.2 ± 1.4 (1.0–6.0). The mean post-operative VAS scores decreased significantly after 1 day (3.2 ± 1.4, p < 0.05), 1 week (1.8 ± 1.0, p < 0.001), 4 weeks (1.4 ± 1.3, p < 0.001), 12 weeks (1.1 ± 0.8, p < 0.001), and 24 weeks (1.0 ± 0.7, p < 0.001) (). The daily use of morphine also decreased significantly, with a daily dose of 105.2 ± 32.7 mg (30–150 mg) pre-operatively and 41.3 ± 9.6 mg (30–60 mg) 24 h post-operatively. The mean daily post-operative morphine doses were as follows: 1 week, 31.5 ± 12.2 mg (p < 0.001); 4 weeks 19.6 ± 12.4 mg (p < 0.001); 12 weeks 14.5 ± 9.6 mg (p < 0.001); and 24 weeks 13.9 ± 9.3 mg (p < 0.001) (). Before the operation, ODI scores ranged from 20.0 to 56.0, with an average of 41.7 ± 6.7. The mean ODI score at 4 weeks post-operatively was 21.9 ± 5.5 (10.0–30.0). VAS scores and morphine dosages throughout the follow-up period are shown in . The ODI scores at 4, 12, and 24 weeks post-operatively were significantly lower than the preoperative ODI score (). One patient had local tumor progression during the follow-up period. None of the patients died during the 6-month follow-up.
Figure 3. VAS score before and after the procedure. VAS: Visual Analog Scale. The VAS scores showed statistical significance pre- and post-MWA combined with PVP (p < 0.05).
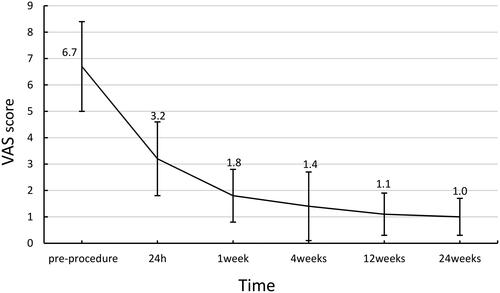
Figure 4. Daily morphine dose before and after the procedure. The daily morphine dose showed statistical significance pre- and post-MWA combined with PVP (p < 0.001).
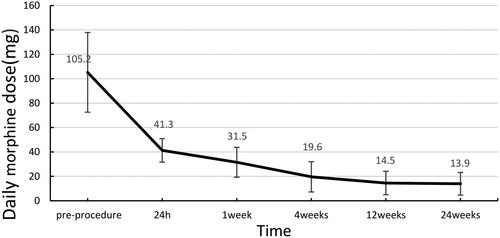
Figure 5. ODI score before and after the procedure. ODI: Oswestry Disability Index. The ODI scores showed statistical significance pre- and post-MWA combined with PVP (p < 0.05).
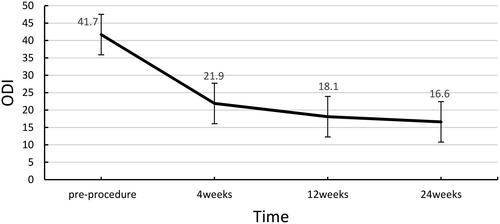
Complications
Complications during the operation were graded according to the Society of Interventional Radiology (SIR) guidelines [Citation14]. One patient (4.3%) developed a mild skin infection at the puncture site (SIR grade B). He was treated with oral antibiotics and was cured within 5 days. No cases of skin burn, nerve injury, bone cement embolism, or perioperative death were observed.
Discussion
This study describes our preliminary experience of combined MWA and PVP for treating malignant high thoracic vertebral metastases in patients who do not benefit from other non-invasive treatment methods. This combination therapy was successfully completed in all 23 patients.
MWA is advantageous in the treatment of bone metastases because it can achieve inactivation without removing the tumor and relieve the pain caused by bone tumors and metastases. Several possible mechanisms of pain relief have been proposed. One potential mechanism is that ablation directly destroys the adjacent sensory nerve fibers, inhibiting the transmission of pain. It has also been suggested that ablation functions by reducing the volume of tumors to relieve nerve fiber compression. The last mechanism proposes that ablation destroys tumor cells that produce cytokines involved in neurostimulation (tumor necrosis factor, interleukins, and so on), which reduces pain transmission and inhibits osteoclastic activity that causes pain [Citation14–16].
The study by Kastler et al. showed that, compared with RF ablation, MWA appears to be more effective in high impedance tissue, such as bone, particularly in osteosclerotic lesions. MWA also seems to be less affected by heating and desiccation of the surrounding tissue than RF ablation, resulting in deeper penetration and more effective heating [Citation6,Citation17]. MWA should particularly be used in lesions close to vital structures, such as spinal lesions, to avoid medullary lesions [Citation17]. However, MWA cannot increase the structural stability of the affected vertebral body. Galibert et al. first reported the application of PVP in 1987. After nearly 30 years of development, PVP is now widely used in the treatment of painful vertebral compression fractures caused by osteonecrosis, metastatic diseases, and even benign primary bone tumors [Citation18]. PVP not only relieves pain but also increases the structural support of the vertebral body. The main advantage of combined MWA and PVP is the optimal cement distribution, especially for infiltrative tumors that have extended to nearby tissues. Optimal cement distribution engulfs the extraosseous extension itself and enhances the efficacy of the ablative technique [Citation19]. The thermal effect of bone cement can also kill tumor cells.
Furthermore, the combination of MWA and PVP has a synergistic anti-tumor and analgesic effect [Citation19]. In this study, the average VAS score of the 23 patients significantly decreased from 6.7 pre-operatively to 1.0 post-operatively. However, it is important to note that cases with an incomplete posterior vertebral body edge are contraindicated for PVP. Hoffmann et al. reported that ablation before osteoplasty could destroy tumor tissue; therefore, ablation increases the safety of osteoplasty because it obliterates tumor vessels and changes the consistency of the tumor, allowing bone cement to be more easily distributed within the lesion [Citation20]. Hence, ablation combined with vertebroplasty can be used to treat vertebral metastases. This combination also converts the contraindications of PVP into indications, which greatly expands the scope of application. Schaefer et al. reported that coagulation necrosis can be produced by RF heat ablation, leading to homogenous distribution of the polymethylmethacrylate in the lesion [Citation21]. In this study, a total of 11 patients with the destruction of the posterior edge of the vertebral body were able to receive safe injections of bone cement for vertebral reconstruction, further demonstrating the advantage of combining MWA with PVP.
Bone cement leakage can occur in several ways. Bone cement can leak along the posterior venous plexus of the vertebral body to the vertebral basal vein at the posterior edge of the vertebral body. Leakage can also occur along with the interruption of the bone cortex of the vertebral body, with the interruption of the cortical continuity of the vertebral body being a risk factor for leakage. When the vertebral cortex is continuously interrupted, the bone cement may leak into the spinal canal and nerve root canal, resulting in nerve damage and its corresponding symptoms [Citation22]. When bone cement leaks in this way, we have found that first injecting 0.5 ml of viscous bone cement at the cortical fracture, pausing for 30 s, and then continuing to inject cement after the cortical fracture is blocked reduces the risk of leakage.
In this study, MWA combined with PVP was applied to the high thoracic vertebrae (T1–T4). Compared with the lumbar and lower thoracic vertebrae, the volume of the high thoracic vertebrae is relatively small and the average width of the pedicle is <4 mm [Citation23]. Kyphosis of the vertebral body has a large angle and is located adjacent to the lungs, large blood vessels, and other important organs [Citation5]. Hence, puncturing could cause pneumothorax and massive hemorrhage. Moreover, the upper thoracic vertebrae are covered by the overlapping bones of the shoulders, and it is often laborious to observe needle placement and bone cement deposition under digital subtraction angiography [Citation24]. Thus, conventional digital subtraction angiography-guided PVP is rarely used in the treatment of high thoracic vertebrae metastases. C-arm CT is not widely used because of the limitations of the equipment. Edwin et al. used CT-guided vertebroplasty during polymethylmethacrylate injection and found that this technique prevented substantial leaking through the posterior wall defect [Citation25]. The advantage of CT guidance is the tomographic display combined with three-dimensional reconstruction, which clearly shows the spatial structure. This allows the surgeon to see the direction of the puncture needle, position of the medial wall of the pedicle, spinal canal, and posterior wall of the vertebral body, encouraging the accurate placement of the puncture needle.
In our study, CT was only used to scan the target plane, reducing the scanning time while simultaneously enabling rapid sagittal reconstruction. The direction and position of the puncture needle can be adjusted in a step-by-step manner, and the puncture needle can be accurately placed parallel to the midline of the vertebral body to reach the anterior one-third of the vertebral body. This positioning prevents the puncture needle from entering the spinal canal and piercing the anterior side of the vertebral body. Moreover, the degree and extent of tumor ablation can be observed during MWA. The diffusion of bone cement can also be clearly observed during injection, and the direction and degree of bone cement leakage can be identified on time. We strictly controlled the speed and total amount of bone cement injected into the high thoracic vertebrae using slow and intermittent injections of a small amount of bone cement. The bone cement was placed in ice-cold saline to prolong the solidification time and CT was performed for each injection of 0.3–0.5 ml. If bone cement has leaked into the spinal canal or intervertebral foramen, the injection of bone cement should be stopped immediately to ensure the safety of the treatment.
The safe temperature range of the neural structure is between 15 and 42 °C [Citation26]. Spinal MWA may damage the spinal cord and nerves. To ensure the safety of treatment, the following methods should be performed to protect the spinal cord and nerves in the process of ablation. First, thermocouples should be used for real-time temperature detection, ensuring thermal monitoring at the interface between the expected ablation area and the at-risk structure [Citation1,Citation26]. Second, the gas should be injected into the spinal canal, causing physical displacement of the at-risk structure [Citation27]. Third, physiological saline should be injected into the spinal canal to reduce the temperature around the risk structure [Citation27]. Fourth, somatosensory/motor evoked potential monitoring should be performed to evaluate the nervous function through visualization of muscle contraction or evoked potential amplitude [Citation27].
Although the posterior edge of the vertebral body was incomplete in 11 cases in our study, patients with tumors whose margins approximated the nerve roots or the spinal canal were excluded. Therefore, there was no real-time temperature measurement or thermal protection in this study. A study by Kastler et al. showed that MWA can be safely performed using short, repeated cycles of ablation to control the diffusion of the heating zone without diminishing the effectiveness of MWA. This method should especially be used for lesions that are close to vital structures to avoid spinal nerve or medullary lesions [Citation1]. In our study, the operations were performed under local anesthesia, and patients were kept fully conscious. Low power (20–30 W) and short repeated MWA cycles (30–60 s) were used to prevent spinal cord and nerve injury, so there was no thermal protection.
There are some limitations to this study. The study was retrospectively performed using data from a single institution. Thus, a higher level of evidence could be achieved by performing a prospective, multicenter trial. Further, the number of cases was not sufficient. Lastly, the main limitation of MWA combined with PVP is the lack of a temperature control device to monitor the real-time temperature.
Conclusions
CT-guided MWA combined with PVP is an effective and safe clinical treatment in patients with high thoracic vertebral metastases. It can relieve pain quickly, control tumor progression, and restore spinal function.
Disclosure statement
No potential conflict of interest was reported by the author(s).
References
- Kastler A, Alnassan H, Aubry S, et al. Microwave thermal ablation of spinal metastatic bone tumors. J Vasc Interv Radiol. 2014;25(9):1470–1475.
- Madaelil TP, Wallace AN, Jennings JW. Radiofrequency ablation alone or in combination with cementoplasty for local control and pain palliation of sacral metastases: preliminary results in 11 patients. Skeletal Radiol. 2016;45(9):1213–1219.
- Chow E, Zeng L, Salvo N, et al. Update on the systematic review of palliative radiotherapy trials for bone metastases. Clin Oncol. 2012;24(2):112–124.
- Lee BH, Kim TH, Chong HS, et al. Prognostic factor analysis in patients with metastatic spine disease depending on surgery and conservative treatment: review of 577 cases. Ann Surg Oncol. 2013;20(1):40–400.
- Hurley MC, Kaakaji R, Dabus G, et al. Percutaneous vertebroplasty. Neurosurg Clin N Am. 2009;20(3):341–359.
- Khan MA, Deib G, Deldar B, et al. Efficacy and safety of percutaneous microwave ablation and cementoplasty in the treatment of painful spinal metastases and myeloma. Am J Neuroradiol. 2018;39(7):1376–1383.
- Seong JY, Kim JS, Jung B, et al. CT-guided percutaneous vertebroplasty in the treatment of an upper thoracic compression fracture. Korean J Radiol. 2009;10(2):185–189.
- Tomasian A, Jennings JW. Vertebral hemangioma: percutaneous minimally invasive image-guided radiofrequency ablation. J Vasc Interv Radiol. 2020;31(11):1949–1952.
- Pezeshki PS, Davidson S, Murphy K, et al. Comparison of the effect of two different bone-targeted radiofrequency ablation (RFA) systems alone and in combination with percutaneous vertebroplasty (PVP) on the biomechanical stability of the metastatic spine. Eur Spine J. 2016;25(12):3990–3996.
- Li L, Jiang XF, Sun LJ, et al. Computed tomography-guided argon-helium cryoablation for sacrum chordoma. Medicine. 2020;99(42):e22604.
- Cazzato RL, de Rubeis G, de Marini P, et al. Percutaneous microwave ablation of bone tumors: a systematic review. Eur Radiol. 2021;31(5):3530–3541.
- Masala S, Guglielmi G, Petrella MC, et al. Percutaneous ablative treatment of metastatic bone tumours: visual analogue scale scores in a short-term series. Singapore Med J. 2011;52:182–189.
- Jacobson RE, Granville M, Hatgis DJ. Targeted intraspinal radiofrequency ablation for lumbar spinal stenosis. Cureus. 2017;9(3):e1090.
- Toyota N, Naito A, Kakizawa H, et al. Radiofrequency ablation therapy combined with cementoplasty for painful bone metastases: initial experience. Cardiovasc Intervent Radiol. 2005;28(5):578–583.
- Nakamura N, Igaki H, Yamashita H, et al. A retrospective study of radiotherapy for spinal bone metastases from hepatocellular carcinoma (HCC). Jpn J Clin Oncol. 2007;37(1):38–3837.
- Kelekis A, Lovblad KO, Mehdizade A, et al. Pelvic osteoplasty in osteolytic metastases: technical approach under fluoroscopic guidance and early clinical results. J Vasc Interv Radiol. 2005;16(1):81–88.
- Kastler A, Alnassan H, Pereira PL, et al. Analgesic effects of microwave ablation of bone and soft tissue tumors under local anesthesia. Pain Med. 2013;14(12):1873–1881.
- Westbroek EM, Goodwin ML, Hui F, et al. Thermal injury to spinal cord, a rare complication of percutaneous microwave spine tumor ablation: case report. J Clin Neurosci. 2019;64:50–54.
- Woolf CJ, Allchorne A, Safieh-Garabedian B, et al. Cytokines, nerve growth factor and inflammatory hyperalgesia: the contribution of tumour necrosis factor alpha. Br J Pharmacol. 1997;121(3):417–424.
- Hoffmann Ralf T, Jakobs TF, Trumm C, et al. Radiofrequency ablation in combination with osteoplasty in the treatment of painful metastatic bone disease. J Vasc Interv Radiol. 2008;19(3):419–425.
- Schaefer O, Lohrmann C, Herling M, et al. Combined radiofrequency thermal ablation and percutaneous cementoplasty treatment of a pathologic fracture. J Vasc Interv Radiol. 2002;13(10):1047–1050.
- Nieuwenhuijse MJ, Van Erkel AR, Dijkstra PDS. Cement leakage in percutaneous vertebroplasty for osteoporotic vertebral compression fractures: identification of risk factors. Spine J. 2011;11(9):839–848.
- McLain RF, Ferrara L, Kabins M. Pedicle morphometry in the upper thoracic spine: limits to safe screw placement in older patients. Spine. 2002;27(22):2467–2471.
- Bayley E, Clamp J, Boszczyk BM. Boszczyk Bronek M, Percutaneous approach to the upper thoracic spine: optimal patient positioning. Eur Spine J. 2009;18(12):1986–1988.
- Kroft Lucia LE, Dijkstra JM, Sander PD. Treatment of vertebral tumor with posterior wall defect using image-guided radiofrequency ablation combined with vertebroplasty: preliminary results in 12 patients. J Vasc Interv Radiol. 2007;18(6):741–747.
- Kastler A, Krainik A, Sakhri L, et al. Feasibility of real-time intraprocedural temperature control during bone metastasis thermal microwave ablation: a bicentric retrospective study. J Vasc Interv Radiol. 2017;28(3):366–371.
- Cazzato RL, Auloge P, De Marini P, et al. Spinal tumor ablation: indications, techniques, and clinical management. Tech Vasc Interv Radiol. 2020;23(2):100677.