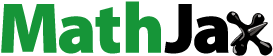
Abstract
Background
The therapeutic capacity of multiple cylindrical interstitial laser ablations (CILAs) of pancreatic tissue was evaluated with 1064 nm laser light in ex vivo and in vivo porcine pancreatic models.
Methods
A diffusing applicator was sequentially employed to deliver 1064 nm laser light in a cylindrical distribution to ablate a large volume of pancreatic tissue. Ex vivo tissue was tested at various power levels (5, 7, and 10 W) under US imaging. An in vivo porcine model was used to evaluate the clinical feasibility of multiple CILAs on pancreatic tissue at 5 W via laparotomy (N = 3).
Results
Multiple CILAs symmetrically ablated a range of ex vivo tissue volumes (2.4–6.0 cm3) at various power levels. Multiple CILAs warranted a therapeutic capacity of symmetrically ablating in vivo pancreatic tissue. Both ex vivo and in vivo pancreatic tissues after multiple CILAs at 5 W confirmed the absence of or minimal thermal injury to the peripheral tissue and carbonization.
Conclusions
The current findings suggest that the collective thermal effects from multiple CILAs can help widely ablate pancreatic tissue with minimal thermal injury. Further in vivo studies will investigate the safety of the proposed CILA treatment as well as acute/chronic responses of pancreatic tissue for clinical translations.
1. Introduction
Pancreatic cancer (PC) is the third most common cause of cancer mortality in the United States, with the lowest survival rate among major cancers [Citation1,Citation2]. Five-year survival rates of PC in patients still remain low in spite of a slight increase (from 0.9 to 4.2%) between 1975 and 2011 [Citation1]. The efficacy of PC treatments is dependent on the patient status (age, gender, grade, tumor size, and TNM stage) and treatment methods (resection and nonresection) [Citation1]. For unresectable PC patients, several locally advanced PC treatments have been developed, such as radiofrequency ablation (RFA), microwave ablation (MWA), irreversible electroporation (IRE), and interstitial laser ablation (ILA) via laparotomy or endoscopic approach [Citation3–8]. As a thermal ablation method, RFA using tissue impedance involves placement of single or multiple electrodes inside the tumor. However, the direct contact of the electrodes with the tissue often causes a high risk of postprocedural complications, including mechanical trauma and bleeding [Citation4,Citation9,Citation10]. Conversely, IRE was developed as a nonthermal ablation technique for treating local solid tumors through direct contact by creating nano-sized pores in the cellular membranes and by inducing the irreversible homeostatic disruption in the tumor cells [Citation11,Citation12]. IRE pulses must be synchronized with an electrocardiogram (ECG) according to the refractory period of the cardiac rhythm under general anesthesia to minimize the risk of arrhythmia and muscle tetany. However, due to weak and unstable insertions of IRE electrodes, positioning the electrodes properly in the tumor is quite challenging, which requires a high level of technical skill during the procedure [Citation11,Citation13]. Among numerous treatment methods, endoscopic ultrasound (EUS)-guided Nd: YAG ILA using an end-firing flat fiber can be an alternative option for treating unresectable PC owing to minimal invasiveness, high precision, and low trauma [Citation7,Citation8,Citation14]. Di Matteo et al. performed the first clinical study on nine patients without the use of complex techniques and adverse events [Citation7]. Although the ablation lesion exhibited an evident cytoreductive effect 30 days after the laser treatment, the study showed the incomplete ablation of the entire tumor (single ablation) and only 7.4 months of mean survival (29–662 days) [Citation7]. Thus, further improvements for the endoscopic laser treatment of unresectable PC are still required to ensure the complete tumor ablation as well as treatment safety.
The eventual goal of PC treatment is to achieve complete damage to tumor cells as well as a sufficient safety margin for the surrounding healthy tissue and crucial organs (blood vessels and biliary duct). Even at the late stage of cancer, most PC remains either clinically silent or occasionally diagnosed when a tumor grows to a large size (i.e. 2 ∼ 5 cm3) [Citation1]. In the clinical environment, treating a large tumor requires three or more ablations to cover the entire tumor region. Furthermore, IRE is used only for laparotomy procedures, while RFA and ILA are applicable for both laparotomy and endoscopic approaches. As a single RFA electrode generates a small ablation zone (i.e. 0.13 ∼ 0.34 cm3), multiple insertions of the electrodes are often necessary to encompass a large tumor. However, the multiple procedures prolong operation time and carry undesired risk, thus resulting in discomfort and complications for patients [Citation15]. In contrast, EUS-guided ILA with a deep optical penetration (i.e. ≥4 mm) can be a feasible and well-tolerated method to create larger ablation volumes (up to 2.3 cm3 with single insertion) and to ablate locally advanced PC both in vivo and human trials [Citation16]. Thus, ILA with deep and wide light distribution may lead to the minimal insertions of an optical fiber to ablate large-sized tumors, in contrast to RFA.
In spite of clinical feasibility, ILA uses an end-firing flat fiber with forward emission (top; ) that generates a high irradiance (7 kW/cm2 at 5 W) at the fiber tip, leading to nonuniform small-sized ablation, excessive thermal injury, and/or significant carbonization in the treated and peripheral tissues [Citation8,Citation16,Citation17]. On the other hand, the current study applies a diffusing applicator with circumferential light emissions for laser ablation of pancreas in order to overcome the limitations of the end-firing flat fiber and to treat the tissue uniformly via cylindrical interstitial laser ablation (CILA; ; bottom). Unlike the end-firing flat fiber (forward emission), the diffusing applicator can provide a lower power density (38 W/cm2 at 5 W) because of a wide light coverage, which is capable of ablating a large volume of the targeted tissue with no excessive heating and no or minimal tissue carbonization [Citation18–20]. Hence, the aim of the current study is to demonstrate a therapeutic capability of multiple CILAs to ablate a wide range of pancreatic tissue volumes at various power levels in both ex vivo and in vivo porcine models and to develop an EUS-guided multiple CILAs to treat the large-sized PC (target volume = 2 ∼ 5 cm3) with enhanced safety. We hypothesized that the combined photothermal effects from individual cylindrical light emissions could generate a uniformly large ablation volume in a predictable manner along with the minimum number of applicator insertions and no or minimal thermal injury/carbonization. Numerical simulations, ex vivo testing, and in vivo validations were performed to quantitatively evaluate the proposed multiple CILAs method in terms of spatio-temporal response of temperature, the extent of thermal necrosis, and histological responses of the pancreatic tissue to CILA. compares various features among RFA, ILA, and CILA for EUS-guided ablation of PC.
Figure 1. Cylindrical interstitial laser ablation (CILA) of pancreatic tissue: (a) comparison light emission between interstitial laser ablation (ILA: forward emission) and CILA (diffuse emission), and (b) image of diffusing applicator (DA) with uniform He-Ne light distribution through 19-G biopsy needle (GC: glass cap, P and D = proximal and distal ends of DA).
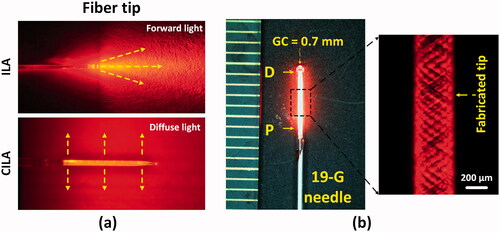
Table 1. Qualitative comparisons among RFA, ILA, and CILA.
2. Materials and methods
2.1. Numerical simulation
Numerical simulations were implemented to predict the thermal effect of multiple CILAs on porcine pancreatic tissue in terms of spatio-temporal developments of the temperature field and the extent of thermal damage. To emulate multiple CILAs in clinical scenarios, a diffusing applicator was sequentially inserted into porcine pancreatic tissue four times. Heat transfer in pancreatic tissue was analyzed by using the Pennes bioheat transfer equation integrated with the Beer’s law; the equation was solved by using COMSOL Multiphysics software (v5.3, COMSOL Inc., Burlington, Massachusetts) [Citation21,Citation22]. The Arrhenius equation defined the extent of thermal damage in tissue as a function of temperature and time. The final ablation volume was calculated by using the ‘Volume integration’ function in the COMSOL software. Numerical results were compared to experimental data to validate the thermal and histological responses of the treated pancreatic tissue to multiple CILAs. In order to evaluate the accuracy of the simulation models, deviation was calculated by dividing the difference between modeling and experimental results by the experimental one. The detailed descriptions of the numerical simulations are included in Appendix 1 (Supplementary material).
2.2. Ex vivo multiple CILAs tests
Multiple CILAs were employed on pancreatic tissue to evaluate a clinical capacity to treat large tumors of ≥2 cm3. Porcine pancreatic tissue was procured from a local slaughterhouse. A total of 15 pancreas specimens were cut into 30 × 60 × 30 mm3 sections and stored at 4 °C to minimize any structural deformation. Before ablation tests, each prepared specimen was placed on a 3 D-printed holder, and the initial tissue temperature was maintained at 20 °C. A 0.9% saline solution was used to minimize tissue dehydration during the experiments as the solution was considered to be isotonic to blood and other bodily fluids [Citation23]. A diffusing applicator (active length = 6 mm, core fiber diameter = 400 μm, glass cap diameter = 0.7 mm; Tecure, Inc., Busan, South Korea) was used to emit laser light in a circumferential direction (), which is compatible with a commercial endoscopic needle (standard 19-G). The micro-machined surface of the applicator allowed for a partial internal reflection inside the fiber for radial light diffusion. A He-Ne image visually confirmed the uniform light emissions along the diffusing applicator (). The detailed fabrication process is given in our previous study [Citation18]. Based on the mean pancreatic tumor size from clinical diagnosis [Citation1,Citation24,Citation25], the current study assumed a spherical tumor (2 cm diameter) to be located at the center of the pancreatic tissue. Then, four sequential insertions of the diffusing applicator through a 19-G needle (JK medical ltd., South Korea) were placed into the pancreatic tissue to surround the simulated tumor at the angles of 0°, 90°, 180°, and 270° (5 mm away from tissue center). Both distance and layout of the applicator insertions were determined by using a small ruler before each CILA. As each insertion created a circumferential ablation lesion, the four insertions resulted in a collective ablation lesion that could encompass the entire target tumor (). Owing to a deep optical penetration, a 1064 nm Nd: YAG laser system (FC-W-1065, CNI, Changchun, China) was selected for the current ablation testing. An US system (UGEO HM70A, Samsung Medison Co., Ltd, Seoul, South Korea) with a 4–7 MHz probe (L4-7) confirmed the precise position of the single diffusing applicator within the tissue. A thermocouple probe (HYP2-21, Omega Inc., Norwalk, USA) was located at the center of the simulated tumor (5 mm away from diffusing applicator) to monitor the interstitial temperature elevations in real-time during irradiation (). The thermocouple probe also monitored a temperature decrease after each CILA to achieve the same initial temperature (i.e., cooling time = 5 ∼ 10 min). The transverse US image (x-y plane) in displays the positions of the diffusing applicator and the thermocouple inside the pancreatic tissue. Based on the previous studies [Citation16,Citation26], the applied power levels were 5, 7, and 10 W, and a total energy of 4000 J (energy for each treatment = 1000 J) was delivered to assess the collective thermal effects from multiple CILAs on the pancreatic tissue. Ablation area (Ai) created by an individual applicator was estimated by assuming a circular shape of each ablation [Citation26] and by using the formula Ai= π·Ri2, where Ri denotes the radial ablation thickness estimated from the treated tissue at the insertion position of the diffusing applicator (i = 1, 2, 3, and 4). The corresponding irradiation times were 200, 143, and 100 s at 5, 7, and 10 W for individual CILA, respectively. Each condition was repeated five times (N = 5).
Figure 2. Experimental set-up for multiple circumferential interstitial laser ablations (CILAs) of porcine pancreatic tissue: (a) illustration of CILA in ex vivo pancreatic tissue with four sequential insertions of diffusing applicator under ultrasound (US) guidance and (b) three CILAs in in vivo pancreatic tissue after laparotomy (TC: thermocouple, D1∼D4: inserting positions of diffusing applicator).
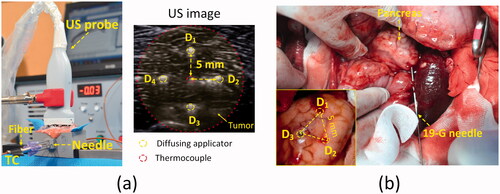
2.3. In vivo multiple CILAs tests
Three mini-pigs (30 ∼ 35 kg) were used for in vivo multiple CILAs tests. All animal experiments were conducted according to the Korean National Institutes of Health guidelines. The protocol was approved by the Institutional Animal Care and Use Committee at KNOTUS, South Korea (Permit Number: 20-KE-493). Before the tests, all the animals were starved overnight, and general anesthesia was induced with intramuscular injection (0.04 mg/kg atropine sulfate, 2 mg/kg xylazine, and 5 mg/kg tiletamine-zolazepam). Following intubation, the general anesthesia was maintained throughout the experiments with a ventilator providing isoflurane (0.5–2%) and oxygen (2 L/min). Laparotomy was performed by making an upper midline incision (15–20 cm) and subsequently placing a Balfour retractor. Due to a smaller size of in vivo pancreatic tissue than that of ex vivo tissue, single and three sequential CILAs were instead employed at 5 W (each treatment energy = 1000 J) using a 1064 nm laser system to evaluate the accumulative thermal effects. A long 19-G needle (89 mm length; Hakko spinal needle, Seohyun Medical Ltl., South Korea) was initially punctured into the pancreatic tissue and then withdrawn by 10 mm for light delivery. Then, a diffusing applicator was inserted into the 19-G needle to deliver laser energy for ablation after reaching the tissue (). Individual CILA was conducted at three points of an equilateral triangle (5 mm side) in the pancreatic tissue, as shown in . Both distance and layout of the three insertions were carefully determined by using a small ruler and a US imaging system to minimize any experimental errors. Each mini-pig was used for one single and one multiple CILAs experiments to compare the therapeutic capability of ablation in the pancreatic tissue (N = 3 per condition). After the ablation tests, all the animals were euthanized for post-experimental analysis by intravenous injection (100 mg/kg pentobarbital).
2.4. Quantification
After ex vivo ablation experiments, all treated samples were frozen at −80 °C for five hours. Subsequently, the frozen samples were transversely cut into six slices of 1 mm thickness along the diffusing applicator axis. A digital camera (D5100, Nikon Corp., Tokyo, Japan) was used to photograph the cross-sectional specimens. Image J (National Institute of the Health, Bethesda, MD) was used to detect the boundary of the treated tissue (discolored regions) and to measure the extent of radial thickness and the cross-sectional area of each ablated tissue. The entire ablation volume was calculated by integrating a series of the measured ablation areas.
(1)
(1)
where A(x) and dx are the measured ablation area and the thickness of each specimen, respectively.
For pathologic analysis, in vivo tissue samples were fixed in 10% neutral buffered formalin for a week, prepared for histology slides, and then sectioned into 6 μm thick slices. All the prepared slides were stained with hematoxylin and eosin (HE) and imaged at 40 and 400
using a light microscope (ICC50 HD, Leica Camera AG, Wetzlar, Germany) to evaluate photothermal effects of CILA on the pancreatic tissue.
2.5. Statistical analysis
SPSS software (Version 24, SPSS Inc., Chicago, USA) was used for statistical analysis. All data are presented as mean ± standard deviation. For nonparametric statistical analysis, the Mann–Whitney U-test (MU) was used to compare two groups, and p < 0.05 was considered statistically significant.
3. Results
Thermal responses of ex vivo pancreatic tissue to single and multiple CILAs were quantitatively evaluated at various power levels. Numerical simulation presented a slightly circular ablation shape around a diffusing applicator after single CILA at 7 W for 143 s (top; ). The highest temperature occurred around the glass cap surface of the applicator. Experimental measurements with a thermocouple (MP in ; 5 mm away from fiber axis) showed that the interstitial temperature elevation (ΔT) of the tissue increased with irradiation time, regardless of the power level (bottom; ). Each power level delivered a total of 1000 J, and the corresponding irradiation times were 200, 143, and 100 s at 5, 7, and 10 W, respectively. The maximum temperature increased with the applied power level. At 7 W, the maximum temperature elevation in the tissue reached 37 ± 6 °C, indicating that the threshold temperature of the irreversible tissue injury occurred 5 mm away from the diffusing applicator. Both numerical results and experimental data showed a good agreement in the temperature developments of the pancreatic tissue during CILA (deviation ≤ 15%). presents the cross-sectional US images of the pancreatic tissue after delivery of 1000 J at various power levels. US images evidently identified the positions of the diffusing applicator and the thermocouple inside the tissue (). After the laser irradiation, all the power levels showed that the irreversible tissue ablation formed circumferentially around the diffusing applicator. The yellow dotted lines in represent changes in tissue elasticity caused by bubble generation and coagulation necrosis (whitish region). The coagulation area was estimated to be 55, 69, and 114 mm2 for 5, 7, and 10 W, respectively. The extent of coagulation necrosis increased with irradiation time, which was qualitatively confirmed by US monitoring (). The US images showed a temporal development of the whitish regions due to contrast changes, resulting from 7-W CILA on the pancreatic tissue. The top image in illustrates the simulated temperature distributions on the transverse tissue surface after four sequential CILAs in the order of D1, D2, D3, and D4. Each irradiation delivered 1000 J (i.e., 7 W for 143 s; 4000 J for four sequential irradiations). The white boundary indicated a critical temperature (i.e., 60 °C) to define the tissue necrosis region. The numerical simulation yielded a circular shape around the diffusing applicator, indicating uniform ablation during CILA resulting from the cylindrical light emitted from the diffusing applicator. The mean values of the interstitial temperature elevation (ΔT measured at MP in and S1; 5 mm away from applicator axis) increased with the irradiation time during individual CILAs at 7 W (bottom; ). The initial temperatures for individual CILAs were slightly different. Overall, all CILAs elevated the interstitial tissue temperature above 35 °C at the end of the laser irradiation (irradiation time = 143 s).
Figure 3. Quantitative evaluations on thermal responses of ex vivo pancreatic tissue to CILA at various power levels: (a) numerical simulations on spatial development of temperature in tissue after single CILA at 7 W for 143 s (top; MP = measured point at r = 5 mm; P and D = proximal and distal ends) and experimental validations of interstitial temperature elevations (ΔT) at MP measured by thermocouple during single CILA at various power levels (bottom; 5, 7, and 10 W), (b) cross-sectional US images of tissue captured at various power levels (5, 7, and 10 W) during CILA (DA: diffusing applicator; TC: thermocouple; CN: coagulation necrosis), and (c) numerical simulations on temperature distributions in tissue for four sequential CILAs (top) and real-time monitoring of temperature elevations (ΔT) by individual CILA at 7 W for 143 s (bottom; D1∼D4: inserting positions of DA). Note that the values in right bottom corners in (b) represent the estimated volumes of CN.
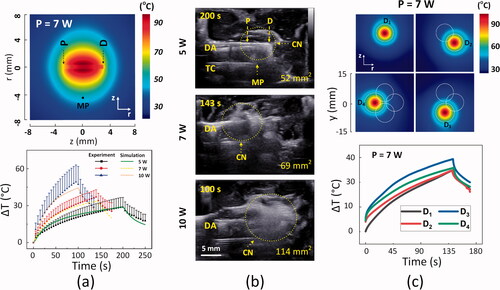
To emulate overlapping treatment of PC in clinical situations, we performed multiple CILAs treatments to ablate a simulated tumor of 2-cm diameter (4000 J delivery for four CILAs). shows collective thermal response of the pancreatic tissue after multiple CILAs at 7 W. Both numerical simulations (top) and experimental testing (bottom) validated that the combined photothermal effects from multiple CILAs generated the overall rectangular ablation, completely encompassing the target tumor at various power levels (transverse view; and S2). In spite of the identical energy delivery, the transverse ablation areas after multiple CILAs increased slightly with the applied power (293 ± 18, 336 ± 23 and 415 ± 58 mm2 for 5, 7, and 10 W, respectively; ). No tissue carbonization and no fiber degradation were observed during/after the testing. All individual CILAs created a comparable extent of ablation area (Ai) in both the numerical results and the experimental data at various power levels (p = 0.32; top; and S2). The total ablation volume (Vt) from multiple CILAs exhibited a linear dependence on the applied power (R2 = 0.99; bottom; ). Multiple CILAs presented the therapeutic capacity of coagulating a wide range of tumor sizes retrieved from clinical studies (i.e., red area [Citation1,Citation7,Citation25]) at various power levels. compares collective ablation performance of the multiple CILAs treatment from numerical simulations and ex vivo tests at various power levels.
Figure 4. Collective thermal responses of pancreatic tissue after multiple CILAs at 7 W for 143 s: (a) transverse images (xy plane) captured from middle position of tissue after four sequential treatments with 4000 J (each treatment energy = 1000 J; sequence of diffusing applicator = D1, D2, D3, and D4) from simulation (top) and experiment (bottom; CN = coagulation necrosis and T: tumor), (b) quantitative comparison of transverse ablation areas (Ai) from individual applicators between simulation and experiment (top; N = 5) and total ablation volumes (Vt) after multiple CILAs at 5, 7, and 10 W (bottom; total energy = 4000 J; N = 5). Note that a red region in (c) represents a range of pancreatic adenocarcinoma volume retrieved from the literature [Citation1,Citation7,Citation25].
![Figure 4. Collective thermal responses of pancreatic tissue after multiple CILAs at 7 W for 143 s: (a) transverse images (xy plane) captured from middle position of tissue after four sequential treatments with 4000 J (each treatment energy = 1000 J; sequence of diffusing applicator = D1, D2, D3, and D4) from simulation (top) and experiment (bottom; CN = coagulation necrosis and T: tumor), (b) quantitative comparison of transverse ablation areas (Ai) from individual applicators between simulation and experiment (top; N = 5) and total ablation volumes (Vt) after multiple CILAs at 5, 7, and 10 W (bottom; total energy = 4000 J; N = 5). Note that a red region in (c) represents a range of pancreatic adenocarcinoma volume retrieved from the literature [Citation1,Citation7,Citation25].](/cms/asset/fad5688f-f593-47c2-b17a-05963619b309/ihyt_a_1972171_f0004_c.jpg)
Table 2. Volumes of multiple CILAs at various conditions (total energy = 4000 J; N = 5).
To evaluate a therapeutic capability of ablating pancreatic tissue, in vivo mini-pig models were tested with 1064 nm laser light at 5 W. After in vivo laparotomy, both single and multiple CILAs at 5 W were applied to the pancreatic tissue for comparison. shows a HE-stained histology image of the transversely sectioned pancreatic tissue (xy plane) after single CILA (1000 J delivered at 5 W). A yellow-dotted line represents the boundary of the coagulation necrosis lesion in the tissue. No carbonization was observed. The small hole at the center depicts the insertion of the diffusing applicator. Coagulation necrosis (pinkish color) was vividly observed in a circular shape (top; 40×), representing circumferentially uniform ablation during single CILA attributed to radial light emissions (). A higher magnification photograph (bottom; 400×) shows the well-demarcated border between coagulation and native tissues. The coagulation lesion involved loss of cellular integrity, whereas the native pancreatic tissue exhibited no cellular deformation. represents a HE-stained histology image of the transversely sectioned tissue (xy plane) after the multiple CILAs treatment (3000 J delivery for three CILAs). Experimental results confirmed that multiple irradiations created the overall circular ablation as a result of the combined photothermal effects. Furthermore, histological analysis verified the evident preservation of biliary duct, vascular structures, and connective tissue after 5-W CILA (). compares ablation volumes between single and multiple CILAs at 5 W. At each power level, multiple CILAs created larger ablation volumes than single CILA (p < 0.05 for 5 W). The ablation volumes from multiple CILAs at 5 W was 1.6 ± 0.3 cm3, respectively, which was 150% larger than those from single CILA at 5 W (0.7 ± 0.2 cm3 at 5 W; p < 0.05; ).
Figure 5. Histological assessments of irreversible thermal ablation on in vivo pancreatic tissue after laparotomy: HE-stained histology images of transverse section (xy plane) from pancreatic tissue treated with (a) single CILA and (b) multiple CILAs at 5 W for 200 s (top: 40 and bottom: 400
CN = coagulation necrosis; NT = native tissue) and (c) comparison of ablation volumes after single and multiple CILAs at 5 W (*p < 0.05; N = 3 per group).
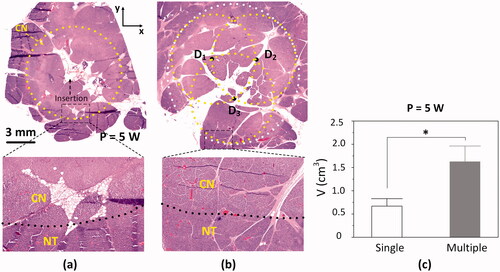
4. Discussion
The current study numerically and experimentally validates the therapeutic capacity of multiple CILAs using a diffusing applicator with the aim to develop a novel laser treatment of pancreatic tumors under EUS guidance. Uniform radial emissions along with the diffusing applicator induced elliptical temperature distributions in the simulations (), which generated uniform ablation volumes in the experiment (). Unlike RFA, multiple CILAs using 1064 nm laser light with a deep penetration depth (i.e. ≥4 mm for LA [Citation27,Citation28] and 0.14 mm for RFA [Citation29]) can ablate a wide range of volumes in the pancreas (2.4 to 6.0 cm3; and SCitation2) owing to the collective thermal effects resulting from application of multiple CILAs. US monitoring visualized the location of each diffusing applicator in the pancreatic tissue and identified the ablated lesion in terms of hyperechoic changes due to micro-bubble generation ( and S1). The multiple CILAs treatment induced insignificant fiber degradation, and no technical difficulties were encountered during fiber insertion and the in vivo tests. Therefore, multiple CILAs with augmented thermal effects and minimal fiber degradation can be beneficial to treating pancreatic tumors in a predictable and reliable manner.
US guidance is considered as a gold standard for monitoring placement of a surgical device and coagulation necrosis during thermal ablation [Citation30,Citation31]. Hyperechoic changes in transverse US images resulted from thermal ablation around the diffusing applicator, which was induced after light absorption by pancreatic tissue ( and S1). For the treatment of a large tumor in clinical scenarios, multiple sequential CILAs may be required to span the entire tumor morphology and to treat irregularly shaped tumors. However, two consecutive CILAs often cause signal interference during US monitoring. The ablation process typically generates multiple bubbles and induces contrast changes in the US images. Thus, CILA has a refractory period of approximately 10 min to achieve the same initial temperature in the tissue and to ensure clear US monitoring for minimizing undesirable outcomes. For clinical translations, simultaneous multiple CILAs treatments must be conducted to evaluate concurrent thermal effects and to decrease treatment time as well as the potential risk of complications in a large-pig model under US guidance. Additional physical quantities, such as temperature at multiple locations, tissue elasticity, and structural changes, should be measured by using a fiber brag grating sensor and US-based elastography. The measured quantities can be compared to the numerical modeling in order to better predict the tissue responses to each CILA and to monitor the laser-tissue interactions in real time. Finally, multiple CILAs should be combined with color Doppler imaging to prevent undesirable thermal injury to the surrounding ducts and vessels as well as to warrant safety during the procedure.
Histological analysis demonstrated a well-defined distinction between necrosis and native tissue after single and multiple CILAs ( and SCitation3). A major cause for local tumor recurrence is heat sink effect as the tumor is often located near large blood vessels, leading to incomplete ablation [Citation32,Citation33]. None or minimal thermal damage to blood vessels and adjacent organs is critical to guarantee the safety of CILA in the pancreatic tumor treatment. Lehmann et al. reported that flow rates in blood vessels can contribute to the heat sink effect during RFA as minimal vascular flows cause strong thermal effects [Citation32]. Sutter et al., showed that the advantage of IRE is capable of preserving major tissue structures, such as hepatic arteries, hepatic veins, portal veins, and intrahepatic bile ducts [Citation34]. In addition, Hank et al. reported that pancreatic tumor resection should ablate a 1-mm margin of normal tissue beyond the tumor for the complete tumor removal [Citation35]. The current findings confirmed the preservation of the surrounding tissues, such as biliary duct, vascular structures, and connective tissue after multiple CILAs with 1 ∼ 2 mm margin although the surrounding tissues were close to the ablated lesion (). The structures of the biliary duct and the blood vessels were maintained possibly due to abundance of collagenous connective tissue and elastic fiber contents [Citation36]. The critical temperature threshold of the elastic fiber tissue is higher than that of normal pancreatic tissue (i.e., 70–80 °C for elastic fiber and 60 °C for the pancreas) [Citation22]. Another explanation is that multiple layered tissue structures (i.e. mucosa, submucosa, muscular, and surrounding connective layers) can disturb photon propagation through the gap junctions, resulting in minimal thermal damage. Further histological studies in in vivo porcine models will be performed to investigate the safety of the proposed multiple CILAs method in terms of acute and chronic wound healing and potential injury to the peripheral tissue structures.
Although multiple CILAs offer potential advantages to thermal ablation of pancreatic tissue, including enhanced treatment capability on various sizes of PC and minimal thermal damage to ductal and vascular structures, experimental limitations still exist. Although histology analysis was employed to determine the extent of ablation zone for acute studies, the accuracy of the HE assessments could be limited by inherent measurement errors due to tissue shrinkage after formalin fixation (∼11%) and slight oblique cross-sectioning of the treated tissue [Citation37]. Both numerical and experimental results showed a good agreement in ablation volumes for both single and multiple CILAs. The numerical simulation is considered as an effective tool to predict the thermal outcomes as well as to determine treatment dosage for CILAs with accuracy for clinical translations. However, the current numerical model merely validated ex vivo findings and considered no changes in the optical properties of the pancreatic tissue during CILA due to lack of the properties for coagulated pancreatic tissue. An adding-doubling method in conjunction with double integrating spheres will thus be implemented to estimate the changes in the optical properties (absorption and scattering coefficients and anisotropic factor) of native and coagulated pancreatic tissues for better numerical modeling of multiple CILAs. In addition, ex vivo and in vivo porcine pancreatic tissues hardly reflect the pathological features of human PC and pancreatic tumors. Scott et al. reported that segmenting CT scans could generate three-dimensional (3 D) models to enhance the accuracy of applicator positioning and treatment parameters during thermal therapy of pancreatic tumor [Citation38,Citation39]. Therefore, the numerical simulation using the dynamic physical properties of human PC and solid tumors in 3 D patient-specific models must be established to determine the optimal treatment dosage and to predict the corresponding treatment volume for individual clinical application. Several blood vessels surrounding PC can play a central role in cooling the interstitial tissue temperature via convective hear transfer and in determining the extent of the irreversible ablation volume in the target tissue. For clinical translations, further in vivo studies must be conducted in tumor-developed animal models to validate the efficacy of multiple or larger blood vessels on the development of the interstitial tissue temperature, the extent of thermal injury, heat-shrink effect, and wound healing during/after multiple CILAs. Moreover, the performance of a smaller diffusing applicator in conjunction with an EUS endoscope will be assessed in terms of its mechanical and structural integrity, flexible insertion into tumor, fiber durability, and fiber degradation during multiple CILAs. Finally, a combination of CILAs with adjuvant chemotherapy and immunotherapy will be explored as an exciting avenue to develop the novel surgical treatment for local tumor destruction.
5. Conclusion
A diffusing applicator with a cylindrical light distribution created a predictable and symmetrical ablation volume without carbonization in ex vivo and in vivo tests. Both numerical and experimental results indicate a therapeutic capacity of multiple CILAs to ablate pancreatic tissue in various sizes. Further investigations using EUS-guided CILA in in vivo porcine models are expected to warrant the efficacy and safety of the proposed laser treatment by assessing acute and chronic responses of pancreatic tissue and wound repair.
Supporting information
Additional supporting information may be found online in the Supporting Information section.
Supplemental Material
Download PDF (959.6 KB)Acknowledgments
The authors thank Seyeon Park for her language editing.
Disclosure statement
No potential conflict of interest was reported by the author(s).
Additional information
Funding
References
- Bengtsson A, Andersson R, Ansari D. The actual 5-year survivors of pancreatic ductal adenocarcinoma based on real-world data. Sci Rep. 2020;10(1):16425–16429.
- Bray F, Ferlay J, Soerjomataram I, et al. Global cancer statistics 2018: GLOBOCAN estimates of incidence and mortality worldwide for 36 cancers in 185 countries. CA Cancer J Clin. 2018;68:394–424.
- Lakhtakia S, Ramchandani M, Galasso D, et al. EUS-guided radiofrequency ablation for management of pancreatic insulinoma by using a novel needle electrode (with videos). Gastrointest Endosc. 2016;83(1):234–239.
- Song TJ, Seo DW, Lakhtakia S, et al. Initial experience of EUS-guided radiofrequency ablation of unresectable pancreatic cancer. Gastrointest Endosc. 2016;83(2):440–443.
- Timmer FE, Geboers B, Ruarus AH, et al. Irreversible electroporation for locally advanced pancreatic cancer. Tech Vasc Interv Radiol. 2020;23(2):100675.
- Martin RC, McFarland K, Ellis S, et al. Irreversible electroporation in locally advanced pancreatic cancer: potential improved overall survival. Ann Surg Oncol. 2013;20 Suppl 3:S443–S449.
- Di Matteo FM, Saccomandi P, Martino M, et al. Feasibility of EUS-guided Nd:YAG laser ablation of unresectable pancreatic adenocarcinoma. Gastrointest Endosc. 2018;88(1):168–174. e161.
- Matteo FD, Martino M, Rea R, et al. EUS-guided Nd:YAG laser ablation of normal pancreatic tissue: a pilot study in a pig model. Gastrointest Endosc. 2010;72(2):358–363.
- Saccomandi P, Lapergola A, Longo F, et al. Thermal ablation of pancreatic cancer: a systematic literature review of clinical practice and pre-clinical studies. Int J Hyperthermia. 2018;35(1):398–418.
- Keane MG, Bramis K, Pereira SP, et al. Systematic review of novel ablative methods in locally advanced pancreatic cancer. World J Gastroenterol. 2014;20(9):2267–2278.
- Jourabchi N, Beroukhim K, Tafti BA, et al. Irreversible electroporation (NanoKnife) in cancer treatment. Gastrointest Intervent. 2014;3(1):8–18.
- Lyu T, Wang X, Su Z, et al. Irreversible electroporation in primary and metastatic hepatic malignancies: a review. Medicine (Baltimore)). 2017;96(17):e6386.
- O’Brien TJ, Passeri M, Lorenzo MF, et al. Experimental high-frequency irreversible electroporation using a single-needle delivery approach for nonthermal pancreatic ablation in vivo. J Vasc Intervent Radiol. 2019;30(6):854–862. e857.
- Jiang T. A, Chai W. Endoscopic ultrasonography (EUS)-guided laser ablation (LA) of adrenal metastasis from pancreatic adenocarcinoma. Lasers Med Sci. 2018;33(7):1613–1616.
- Barret M, Leblanc S, Rouquette A, et al. EUS-guided pancreatic radiofrequency ablation: preclinical comparison of two currently available devices in a pig model. Endosc Int Open. 2019;7(2):E138–E143.
- Di Matteo F, Martino M, Rea R, et al. US-guided application of Nd:YAG laser in porcine pancreatic tissue: an ex vivo study and numerical simulation. Gastrointest Endosc. 2013;78(5):750–755.
- Saccomandi P, Schena E, Caponero MA, et al. Theoretical analysis and experimental evaluation of laser-induced interstitial thermotherapy in ex vivo porcine pancreas. IEEE Trans Biomed Eng. 2012;59(10):2958–2964.
- Truong VG, Park S, Tran VN, et al. Spatial effect of conical angle on optical-thermal distribution for circumferential photocoagulation. Biomed Opt Express. 2017;8(12):5663–5674.
- Truong VG, Tran VN, Hwang J, et al. Effect of spatial light distribution on the thermal response of vascular tissue. Biomed Opt Express. 2018;9(7):3037–3048.
- Tran VN, Lee HS, Truong VG, et al. Concentric photothermal coagulation with basket‐integrated optical device for treatment of tracheal stenosis. J Biophotonics. 2018;11:e201700073.
- Yang D, Converse MC, Mahvi DM, et al. Expanding the bioheat equation to include tissue internal water evaporation during heating. IEEE Trans Biomed Eng. 2007;54(8):1382–1388.
- Welch AJ, Van Gemert MJ. Optical-thermal response of laser-irradiated tissue. Vol. 2. New York (NY): Springer; 2011.
- Hanson KP, Zhang Y, Jiang JJ. Ex vivo canine vocal fold lamina propria rehydration after varying dehydration levels. J Voice. 2011;25(6):657–662.
- Agarwal B, Correa AM, Ho L. Survival in pancreatic carcinoma based on tumor size. Pancreas. 2008;36(1):e15–e20.
- Forssell H, Pröh K, Wester M, et al. Tumor size as measured at initial X-ray examination, not length of bile duct stricture, predicts survival in patients with unresectable pancreatic cancer. BMC Cancer. 2012;12:429.
- Truong VG, Jeong S, Park J-S, et al. Endoscopic ultrasound (EUS)-guided cylindrical interstitial laser ablation (CILA) on in vivo porcine pancreas. Biomed Opt Express. 2021;12(7):4423–4437.
- Kerbage Y, Betrouni N, Collinet P, et al. Laser interstitial thermotherapy application for breast surgery: Current situation and new trends. Breast. 2017;33:145–152.
- Ishikawa T, Zeniya M, Fujise K, et al. Clinical application of Nd:YAG laser for the treatment of small hepatocellular carcinoma with new shaped laser probe. Lasers Surg Med. 2004;35(2):135–139.
- Kreindel M, and Mulholland S. The basic science of Radiofrequency-Based devices. IntechOpen. 2021. DOI: https://doi.org/10.5772/intechopen.96652
- Maybody M. An overview of image-guided percutaneous ablation of renal tumors. Semin Intervent Radiol. 2010;27(3):261–267.
- Ahmed M, Solbiati L, Brace CL, Standard of Practice Committee of the Cardiovascular and Interventional Radiological Society of Europe, et al. Image-guided tumor ablation: standardization of terminology and reporting criteria-a 10-year update. Radiology. 2014;273(1):241–260.
- Lehmann KS, Poch FG, Rieder C, et al. Minimal vascular flows cause strong heat sink effects in hepatic radiofrequency ablation ex vivo. J Hepatobiliary Pancreat Sci. 2016;23(8):508–516.
- De Vita E, De Landro M, Massaroni C, et al. Fiber optic sensors-based thermal analysis of perfusion-mediated tissue cooling in liver undergoing laser ablation. IEEE Trans Biomed Eng. 2021;68(3):1066–1073.
- Sutter O, Calvo J, N'Kontchou G, et al. Safety and efficacy of irreversible electroporation for the treatment of hepatocellular carcinoma not amenable to thermal ablation techniques: a retrospective single-center case series. Radiology. 2017;284(3):877–886.
- Hank T, Hinz U, Tarantino I, et al. Validation of at least 1 mm as cut-off for resection margins for pancreatic adenocarcinoma of the body and tail. J Br Surg. 2018;105(9):1171–1181.
- Liao J, B, Xu, Zhang R, et al. Applications of decellularized materials in tissue engineering: advantages, drawbacks and current improvements, and future perspectives. J Mater Chem B. 2020;8(44):10023–10049.
- Tran T, Sundaram CP, Bahler CD, et al. Correcting the shrinkage effects of formalin fixation and tissue processing for renal tumors: toward standardization of pathological reporting of tumor size. J Cancer. 2015;6(8):759–766.
- Scott SJ, Adams MS, Salgaonkar V, et al. Theoretical investigation of transgastric and intraductal approaches for ultrasound-based thermal therapy of the pancreas. J Ther Ultrasound. 2017;5:10–16.
- Adams MS, Scott SJ, Salgaonkar VA, et al. Thermal therapy of pancreatic tumours using endoluminal ultrasound: Parametric and patient-specific modelling. Int J Hyperthermia. 2016;32(2):97–111.