Abstract
Background
To assess the effects of radiofrequency ablation (RFA) using an internally-cooled wet (ICW) electrode in ex vivo bovine liver and evaluate the feasibility of the ICW electrode for benign thyroid nodules.
Methods
We developed an 18-gauge ICW electrode with a microhole at the distal tip for tissue infusion of chilled (0 − 4 °C) isotonic saline (rate = 1.5 ml/min). RFA using ICW and IC electrodes were performed in bovine livers (40 pairs, 1-cm active tip, 50 W, 1-min). We compared the morphological characteristics of ablation zones and presence of carbonization. Twenty patients with benign thyroid nodules larger than 5 ml were prospectively enrolled in a clinical study and underwent ultrasound-guided RFA with ICW electrodes. Ultrasound examinations, laboratory data, and symptom and cosmetic scores were evaluated preprocedure and 1 and 6 months after the procedure.
Results
In the ex vivo study, the ICW achieved significantly larger ablation zones than the IC (p<.001). In the clinical study, ICW electrodes were tolerable in all patients. At last follow-up, nodule volume had decreased from 15.6 ± 12.1 ml to 4.1 ± 4.3 ml (p<.001), and the mean volume reduction ratio (VRR) was 73.3 ± 13.7% at 6.0 months follow-up. Cosmetic and symptom scores were reduced from 3.52 ± 1.03 to 2.65 ± 0.88 and 3.10 ± 2.17 to 0.85 ± 0.99 (both p<.001), respectively. After RFA, thyroid function was well preserved in all patients, and mean thyroglobulin level decreased from 36.6 ± 52.1 ng/ml to 26.9 ± 62.2 ng/ml. One patient experienced a temporary voice change that recovered within a week.
Conclusions
We developed a thyroid-dedicated ICW electrode that we showed to be feasible and effective in patients with benign thyroid nodules.
Introduction
Radiofrequency ablation (RFA) is used to treat benign thyroid nodules and thyroid cancers, with many studies reporting promising results, and several guidelines having been introduced [Citation1–3]. For benign thyroid nodules, a conventional monopolar internally-cooled (IC) electrode is the standard device used; however, the small size of the ablation zone with such an electrode is a limitation [Citation4–6], and there is a need to increase the ablation volume in patients with large symptomatic benign thyroid nodules.
Several strategies have been proposed to solve this limitation, including hypertonic saline injection before ablation, continuous infusion of saline through the electrode during ablation, bipolar RFA devices and adjustable RFA electrodes [Citation7–10]. Of these approaches, the continuous infusion of saline through the electrode can be an effective method because the injected saline can increase both electrical and thermal conductivity, and the higher boiling point of the saline can be helpful for avoiding rapid boiling of surrounding tissue adjacent to the electrode [Citation9,Citation11]. Miao et al. reported that a cooled-wet electrode, which allowed simultaneous internal cooling perfusion and interstitial saline infusion, was more efficient at creating large ablation zones in rabbit liver tumors than other monopolar electrodes [Citation12].
It was first suggested in the liver that an IC wet (ICW) electrode makes a larger ablation zone than a conventional IC electrode. Therefore, we considered that ICW electrodes may be potential to be suitable for treating large benign thyroid nodules. Although ICW electrodes have been used for liver tumors [Citation12–14], to the best of our knowledge, no studies have applied an ICW electrode to thyroid nodules. In addition, there are no suitable ICW devices for thyroid application.
Therefore, the primary purpose of this study was to develop a thyroid-dedicated ICW electrode device. The secondary purposes were to assess the effects of this ICW electrode in ex vivo bovine liver and to evaluate the feasibility of an ICW electrode for treatment of benign thyroid nodules through a clinical trial.
Materials and methods
The protocol for the experimental prospective clinical study was approved by the Ethics Committee of the Institutional Review Board of Asan Medical Center (Institutional Review Board number: 2019-0759). Written informed consent was obtained from all patients before RFA.
RF devices
A thyroid-dedicated ICW electrode was developed by modifying an 18-gauge IC electrode. The 18-gauge ICW electrode had a microhole (0.04 mm in diameter) in the distal active tip area, allowing tissue infusion at a rate of 1.5 ml/min (). An 18-gauge IC electrode (RFTP-0710N; RF Medical) with a 1 cm active tip was used for comparison procedures.
Figure 1. The thyroid-dedicated internally-cooled wet (ICW) electrode system. Photograph (A) and illustration (B) of the ICW electrode system show the propulsion of saline through the one microhole in the distal tip (rate = 1.5 ml/min).
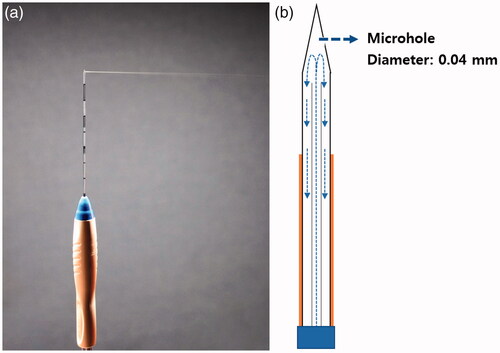
The same 400 kHz generator (V-1000; RF Medical) and peristaltic pump (RFP-300; RF Medical) were used for both the ICW electrode and IC electrode. The peristaltic pump cooled the electrodes internally by delivering chilled (0–4 °C) isotonic saline into the electrodes at a flow rate of 400 revolutions per minute.
Experimental study
A series of RFA zones were induced in freshly excised bovine livers. The temperature of the bovine livers before ablation ranged from 23 °C to 24 °C, and they weighed approximately 7 kg each. The bovine livers were cut into 10 × 10 × 10 cm blocks and the tip of the electrode was inserted into the liver blocks to a depth of 2 cm. An ablation time was 1 min per electrode insertion, with the generator set to deliver 50 W of power using two ablation modes (auto and impedance) for a total of 80 bovine liver blocks (40 using IC electrodes and 40 using ICW electrodes). During ablation, the applied current, power output and tissue impedance were recorded continuously using a computer program (Real Time Graphics Software version 1.2; RF Medical).
Liver blocks containing the RFA zones were dissected along the longitudinal plane passing through the axes of the electrode. As the white central area of the radiofrequency-induced ablation zone was previously shown to correspond with the zone of coagulation necrosis [Citation15], two observers measured the maximum vertical diameter (D1) along the electrode and the transverse diameter (D2) perpendicular to it. The second transverse diameter of the ablation zone (D3) was measured in the perpendicular plane to the plane passing through the axes of the probe (). The measurement was done on ‘central white zone’ except the surrounding ‘red zone’ of hyperemia [Citation16]. The ablation zones were evaluated by calculating the lesion size as D1 × D2. The volumes of the ablation zones were calculated using the following equation: π (D1 × D2 × D3)/6. The formation of carbonization and the RFA parameters used were also evaluated.
Figure 2. Measurement of the ablation zone. Photographs of specimen created by radiofrequency ablation with thyroid-dedicated internally-cooled wet electrode in the electrode insertion axis. (A) The maximum vertical diameter (D1) along the electrode and the perpendicular transverse diameter (D2) were measured. (B) The second transverse diameter of the ablation zone (D3) was measured in the perpendicular plane to the plane passing through the axes of the probe.
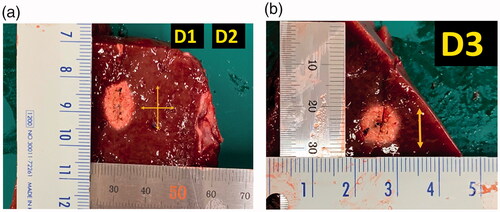
Clinical study
Patients
Between March 2020 and August 2020, 20 patients with benign thyroid nodules underwent US-guided RFA with the ICW electrode. The eligibility criteria for inclusion were as follows: (1) patients between the ages of 20 and 80 years; (2) benign cytology confirmed on evaluation of US-guided fine-needle aspiration or core needle biopsy; (3) a nodule volume larger than 5 ml. The exclusion criteria were as follows: (1) thyroid nodules with suspicious sonographic features; (2) abnormal thyroid function tests.
Pre-procedural assessment
All patients underwent a US examination before RFA. An experienced radiologist (J.H.B., with 24 years of experience in neck US and 18 years of experience in thyroid RFA) conducted all US procedures and US-guided biopsies. The US system (RS 80, Samsung Medison Co., Ltd, Seoul, South Korea) was equipped with a high-frequency linear probe (5–14 MHz). Three orthogonal diameters (the largest diameter and two perpendicular diameters) were measured, and the volume of each nodule was calculated using the equation V=πabc/6, where V is the volume, a is the largest diameter and b and c are the two perpendicular diameters. The nodule composition was graded into four categories on the basis of the proportion of the solid component: (1) solid, no obvious cystic content; (2) predominantly solid, cystic content ≤50%; (3) predominantly cystic, cystic content >50%; and (4) cystic, no obvious solid content. Nodule vascularity was graded into four categories on the basis of a Doppler examination: grade 0, no intra-nodular vascularity; grade 1, peri-nodular vascularity only; grade 2, intra-nodular vascularity <50%; and grade 3, intra-nodular vascularity >50% [Citation17].
Before RFA, a physician recorded a cosmetic score as follows [Citation17]: 1, no palpable mass; 2, no cosmetic problem but a palpable mass; 3, cosmetic problem on swallowing only; and 4, readily and always detected a cosmetic problem. Patient-determined symptom scores were rated using a visual analog scale from 0 to 10 as baseline data.
Laboratory tests were also performed for thyroid function (thyroid stimulating hormone [TSH], triiodothyronine [T3] and free thyroxine [fT4]), calcitonin, thyroglobulin, complete blood count (CBC) and blood coagulation (prothrombin time and activated partial thromboplastin time).
Ablation procedures
Each patient was placed in a supine position with the neck extended. After sterilization, vessels located along the approach route were identified, and 2% lidocaine was injected into the skin puncture and perithyroidal area. All procedures were performed by the same radiologist who evaluated the pre-procedural US images.
RFA was performed using an 18-gauge ICW electrode with a 0.7 or 1.0 cm active tip (RFTSP-0710N; RF Medical), which was the same electrode used for the excised bovine livers in the experimental study. RFA was performed using the trans-isthmic approach and a moving-shot technique, with 30–90 W of radiofrequency power, depending on the size and characteristics of the target nodule and the active tip size. Ablation was terminated when the entire target lesion was covered with hyperechoic microbubbles.
Procedure-related pain was graded into four categories and recorded: grade 0, radiofrequency power did not have to be turned off because the patient experienced no pain; grade 1, radiofrequency power was turned off once or twice to reduce pain levels; grade 2, radiofrequency power was turned off more than three times; and grade 3, the procedure was incompletely terminated because of severe pain. Complications and side effects were defined according to the quality improvement guidelines of the Society of Interventional Radiology and a prior multicenter evaluation of complications [Citation18,Citation19]. Each patient was monitored in the hospital for 1–2 h after RFA.
Follow-up and outcome assessment
Follow-up US was performed at 1- and 6-month after RFA, with changes in nodule size and volume being evaluated. Volume reduction was calculated as: volume reduction ratio (VRR) (%) = ([initial volume − final volume] 100)/initial volume. Therapeutic success was defined as a volume reduction >50% of the initial nodule volume at the 6-month follow-up US examination [Citation7]. The therapeutic success rate was defined as the percentage of successfully treated nodules [Citation20]. Laboratory tests including thyroid function (TSH, T3 and fT4) and thyroglobulin were assessed at 6-month follow-up.
Statistical analysis
Statistical analyses were performed using SPSS for Windows version 23.0 (SPSS, Chicago, IL). The long-axis diameter, short-axis diameter and calculated volumes of the RFA zones are reported as the mean ± standard deviation. Kolmogorov–Smirnov test was used to evaluate the normal distribution of the diameters, calculated volumes of the ablation zones and ablated nodules obtained in each study, and the electrical parameters. The diameter, calculated volume and electrical parameters were compared using Student’s t-test. The incidences of carbonization and power cutoffs in the experimental study were compared using Fischer’s exact test. All p values were two-sided, and p<.05 was considered statistically significant.
Results
RFA parameters and ablation characteristics in the experimental study
The RFA parameters used in the experimental study are listed in . The incidences of cutoffs and lowering of power were significantly lower with the ICW electrode group than with the IC electrode group (cutoffs: 2/20 vs. 20/20, p<.001; lowering of power: 5/20 vs. 20/20, p<.001). Using the auto-mode, the true radiofrequency time (total ablation time – cutoff time) was significantly longer with the ICW electrode than with the IC electrode (58.62 ± 4.2 s vs. 33.28 ± 3.4 s, p<.001). As a consequence, the total delivered energy was much higher with the ICW electrode than with the IC electrode (2931.0 ± 212.4 kJ vs. 1664.2 ± 170.8 kJ, p<.001).
Table 1. Radiofrequency ablation parameters used in the experimental study.
The morphology of the ablation zone was elliptical in all specimens with both the IC and ICW electrodes. There were no carbonizations with either of the electrodes in either the auto-mode or impedance-mode.
After RFA, a well-defined area with a central white discoloration was observed. The mean long-axis diameter, short-axis diameter, vertical diameter and calculated volumes for the ablation zones are summarized in . In the gross specimens, the mean long-axis, short-axis and vertical diameters of the ablation zones in the ICW group were significantly larger than those in the IC group in both auto-mode and impedance-mode (all, p<.001). Therefore, the volumes of the ablation zones were also larger in the ICW group than in the IC group in both auto-mode and impedance-mode (auto-mode: 2189.0 ± 439.0 mm3 vs. 891.7 ± 140.7 mm3, p<.001; impedance-mode: 2425.9 ± 552.4 mm3 vs. 886.3 ± 209.8 mm3, p<.001). With the impedance mode, the volumes of the RF ablation zones were about 2.73 times larger in the ICW group than in the IC group.
Table 2. Radiofrequency ablation size and volume in the experimental study.
Patient demographics and clinical characteristics
The baseline characteristics of the patients and their nodules are summarized in . The mean size and mean volume of the index nodule were 3.9 ± 1.1 cm and 15.6 ± 12.1 ml, respectively. The RFA parameters used in the clinical study are listed in .
Table 3. Baseline characteristics of patients and nodules included in the clinical study.
Table 4. Radiofrequency ablation parameters used in the clinical study.
The laboratory findings in the clinical study were as follows. The CBC and blood coagulation tests of all patients were normal at pretreatment evaluation. The initial mean TSH, fT4 and T3 were 2.1 ± 1.8 μU/ml (0.08–8.2), 1.3 ± 0.3 ng/dl (1.0–2.5) and 149.7 ± 23.0 ng/dl (116–202), respectively. The initial calcitonin and thyroglobulin were 2.1 ± 1.2 pg/ml (1.5–5.2) and 36.6 ± 52.1 ng/ml (1.5–233), respectively. In the initial laboratory test, eight patients had an elevated thyroglobulin level with a mean of 74.8 ± 67.4 ng/ml (range: 32.5–233). After RFA, thyroid function was well preserved in all patients, and the mean thyroglobulin level had significantly decreased to 26.9 ± 62.2 ng/ml at the 6-month follow-up (p=.046). Five out of the eight patients who had an initial elevated thyroglobulin level were normal on a 6-month follow-up after RFA.
Treatment outcomes in the clinical study
shows changes in size and volume over time after treatment. After RFA, significant reductions in the mean volume of the ablated nodules were noted at 1- and 6-month follow-up (p<.001). After the procedures, both symptom and cosmetic scores showed significant decreases at 1- and 6-month follow-up (all p<.001).
Table 5. Ultrasound features and cosmetic and symptom scores in patients before and after the procedures.
Safety
Several patients who underwent RFA reported mild pain at the ablated site, or pain radiating to the head, ear, shoulder or teeth during the procedure. However, the pain was reduced when the generator output was reduced or turned off during ablation. All patients tolerated the procedure. Transient voice change was observed in one patient, but the patient had recovered spontaneously after 1 week. No patient experienced a significant complication that needs hospitalization or delayed complication during the follow-up.
Discussion
In this study, we developed a thyroid-dedicated ICW electrode and tested it in an experimental and prospective clinical study. We demonstrated that the thyroid-dedicated ICW electrode is efficacious, technically safe and feasible. In terms of efficacy, the induced energy (kJ) delivered by the ICW electrode was higher than that delivered by the IC electrode in all experimental RF sessions, and the ICW electrode, therefore, created a larger ablation zone than the IC electrode. In addition, in the clinical study, the RFA was tolerated by all patients without major complications which need hospitalization. We achieved a therapeutic success rate of 100% at 6-month follow-up with a mean VRR of 73.3% ± 13.7%. To our knowledge, this study is the first clinical pilot study to evaluate the efficacy, safety, and feasibility of a thyroid-dedicated ICW electrode.
The effectiveness of the ICW electrode can be explained by the effect of the saline perfusion, which minimizes any rapid increase in impedance. The relatively small ablation size of the conventional monopolar RFA system is related to decreased current density, with a rate proportional to the inverse square of the distance from the electrode [Citation4,Citation5]. That is because the resulting heating in the immediate vicinity of the electrode leads to rapid charring and decreased current delivery. However, the ICW electrode overcomes these problems by infusing isotonic NaCl solution, which increases both the electrical and thermal conductivity. The infused chilled (0–4 °C) isotonic NaCl solution also lowers the tissue temperature around the electrode and prevents charring at the electrode-tissue interface. Consequently, the ICW electrode allows a low impedance to be maintained during the procedure [Citation9,Citation11].
Liver-dedicated ICW electrodes had multiple side holes with the relatively proximal position. However, the position and number of the side holes of liver-dedicated ICW electrodes are not optimal for application to benign thyroid nodules with moving shot technique [Citation10,Citation21]. During the moving shot technique, saline flowing from the proximal side holes flows to the dependent portion by gravity, ablating an unexpected ablation zone. Therefore, we developed a thyroid-dedicated ICW with an effective design; one co-axial lumen, one distal microhole and positioning of the microhole in the distal tip portion. In thyroid RFA, the distal positioning of a microhole is important, as the moving-shot technique is the standard technique, and tissue coagulation starts at the distal portion of the electrode tip during the moving shot [Citation22,Citation23].
In our ex vivo study, the thyroid-dedicated ICW electrode created a larger ablation zone than the IC electrode, and in our clinical study, we achieved a mean VRR of 73.3 ± 13.7% at 6-month follow-up after a single-session of RFA. This result is similar or slightly superior to the 12-month results reported in a recent systematic review (67–75% VRR)[Citation2].
In all patients, thyroid function was well preserved after RFA. Among the eight patients who had an initially elevated serum thyroglobulin level, five had the level return to the normal range at 6-month follow-up. Additionally, the mean pretreatment serum thyroglobulin level was significantly lower at 6-month follow-up after RFA (36.6 ± 52.1 vs. 24.8 ± 55.5, p = 0.046). In previous studies, a decrease in thyroglobulin level was attributed to the reduction of nodule volume through RFA [Citation24,Citation25].
Our study has several limitations. First, in the bovine liver experimental study, all the ablations involved normal liver parenchyma, not thyroid tissue, not tumor tissue and they were performed ex vivo. The bovine thyroid tissue was too small to be used in an experimental study, and the experimental study was conducted on the ex-vivo bovine liver, referring to the point that had been studied on the liver in the previous thyroid RFA experimental studies. Greater variations in the ablation shape can be found in vivo because of the blood flow-related heat-sink effect, which results in rapid heat exchange. Second, increased ablation zone with thyroid-dedicated ICW electrode may increase the risk of complication, especially in the case of the target nodule with a narrow safety margin with important neck structures. In our study, all cases were performed by an experienced radiologist. Therefore, this thyroid-dedicated ICW electrode is applicable when the size of the nodule is large enough, and careful manipulation is needed when the RFA is done by a physician with less expertise. Third, although we continuously measured the tissue temperature and monitored the impedance before and during RFA in each session, we did not set the electrical conditions to be similar to in vivo studies (e.g., by lowering tissue impedance by instilling saline before RFA). Consequently, it is unclear to what extent the results obtained in this study accurately represents the real situation. Fourth, we only focused on comparing electrical parameters and ablation size between the two different electrodes; the optimization of the electrode profile, including the saline infusion rate, should be validated in further studies. Lastly, although we achieved a VRR of more than 70% at 6 months after RFA, long-term follow-up results are necessary to evaluate the actual clinical impact of the ICW electrodes. Further studies with long-term follow-up are needed to validate the efficacy and feasibility of the thyroid-dedicated ICW electrode in clinical practice. In addition, this study was a clinical pilot study, and only a small number of patients (n = 20) were enrolled in the study. Therefore, future studies with a larger number of patients are needed.
In conclusion, in the ex vivo study, the thyroid-dedicated ICW achieved significantly larger ablation zones than the IC. In the clinical study, the thyroid-dedicated ICW was feasible and effective in patients with benign thyroid nodules.
Disclosure statement
No competing financial interests exist.
Additional information
Funding
References
- Lee M, Baek JH, Suh CH, et al. Clinical practice guidelines for radiofrequency ablation of benign thyroid nodules: a systematic review. Ultrasonography. 2021;40(2):256–264.
- Monpeyssen H, Alamri A, Ben Hamou A. Long-term results of ultrasound-guided radiofrequency ablation of benign thyroid nodules: state of the art and future perspectives—a systematic review. Front Endocrinol (Lausanne). 2021;12:622996.
- Lee JY, Baek JH, Ha EJ, et al. 2020 Imaging guidelines for thyroid nodules and differentiated thyroid cancer: Korean society of thyroid radiology. Korean J Radiol. 2021;22(5):840–860.
- Gazelle GS, Goldberg SN, Solbiati L, et al. Tumor ablation with radio-frequency energy. Radiology. 2000;217(3):633–646.
- McGhana JP, Dodd GD. 3rd. Radiofrequency ablation of the liver: current status. AJR Am J Roentgenol. 2001;176(1):3–16.
- Ha EJ, Baek JH, Baek SM. Minimally invasive treatment for benign parathyroid lesions: treatment efficacy and safety based on nodule characteristics. Korean J Radiol. 2020;21(12):1383–1392.
- Jung SL, Baek JH, Lee JH, et al. Efficacy and safety of radiofrequency ablation for benign thyroid nodules: a prospective multicenter study. Korean J Radiol. 2018;19(1):167–174.
- Lee J, Shin JH, Hahn SY, et al. Feasibility of adjustable electrodes for radiofrequency ablation of benign thyroid nodules. Korean J Radiol. 2020;21(3):377–383.
- Lobo SM, Afzal KS, Ahmed M, et al. Radiofrequency ablation: modeling the enhanced temperature response to adjuvant NaCl pretreatment. Radiology. 2004;230(1):175–182.
- Ni Y, Miao Y, Mulier S, et al. A novel “cooled-wet” electrode for radiofrequency ablation. Eur Radiol. 2000;10(5):852–854.
- Ahmed M, Lobo SM, Weinstein J, et al. Improved coagulation with saline solution pretreatment during radiofrequency tumor ablation in a canine model. J Vasc Interv Radiol. 2002;13(7):717–724.
- Miao Y, Ni Y, Mulier S, et al. Treatment of VX2 liver tumor in rabbits with “wet” electrode mediated radio-frequency ablation. Eur Radiol. 2000;10(1):188–194.
- Kim SK, Gu MS, Hong HP, et al. CT findings after radiofrequency ablation in rabbit livers: comparison of internally cooled electrodes, perfusion electrodes, and internally cooled perfusion electrodes. J Vasc Interv Radiol. 2007;18(11):1417–1427.
- Lee JM, Han JK, Kim SH, et al. Optimization of wet radiofrequency ablation using a perfused-cooled electrode: a comparative study in ex vivo bovine livers. Korean J Radiol. 2004;5(4):250–257.
- Patterson EJ, Scudamore CH, Owen DA, et al. Radiofrequency ablation of porcine liver in vivo: effects of blood flow and treatment time on lesion size. Ann Surg. 1998;227(4):559–565.
- Ahmed M, Solbiati L, Brace CL, et al. Image-guided tumor ablation: standardization of terminology and reporting criteria-a 10-year update. Radiology. 2014;273(1):241–260.
- Kim JH, Baek JH, Lim HK, et al. 2017 Thyroid radiofrequency ablation guideline: Korean society of thyroid radiology. Korean J Radiol. 2018;19(4):632–655.
- Burke DR, Lewis CA, Cardella JF, et al. Quality improvement guidelines for percutaneous transhepatic cholangiography and biliary drainage. J Vasc Interv Radiol. 2003;14(9 Pt 2):S243–S6.
- Park HS, Baek JH, Park AW, et al. Thyroid radiofrequency ablation: updates on innovative devices and techniques. Korean J Radiol. 2017;18(4):615–623.
- Mauri G, Pacella CM, Papini E, et al. Image-Guided thyroid ablation: proposal for standardization of terminology and reporting criteria. Thyroid. 2019;29(5):611–618.
- Kim JH, Kim PN, Won HJ, et al. Percutaneous radiofrequency ablation using internally cooled wet electrodes for the treatment of hepatocellular carcinoma. AJR Am J Roentgenol. 2012;198(2):471–476.
- Baek JH, Lee JH, Valcavi R, et al. Thermal ablation for benign thyroid nodules: radiofrequency and laser. Korean J Radiol. 2011;12(5):525–540.
- Zhang B, Moser MA, Zhang EM, et al. A review of radiofrequency ablation: large target tissue necrosis and mathematical modelling. Phys Med. 2016;32(8):961–971.
- Cakir B, Topaloglu O, Gul K, et al. Effects of percutaneous laser ablation treatment in benign solitary thyroid nodules on nodule volume, thyroglobulin and anti-thyroglobulin levels, and cytopathology of nodule in 1 yr follow-up. J Endocrinol Invest. 2006;29(10):876–884.
- Valcavi R, Riganti F, Bertani A, et al. Percutaneous laser ablation of cold benign thyroid nodules: a 3-year follow-up study in 122 patients. Thyroid. 2010;20(11):1253–1261.