Abstract
Purpose
To compare the accuracy and safety of robotic laser position (RLP) versus freehand for antenna CT-guided microwave ablation (MWA) of single hepatocellular carcinoma (HCC) (diameter < 3 cm).
Materials and methods
This retrospective study was conducted between May 2020 and June 2021. A total of 40 patients with early HCC who underwent CT-guided MWA were divided into two groups: a freehand group (n = 20) and a RLP group (n = 20). Based on in-plane and out-of-plane data, the actual puncture point error (APPE), number of repositioning procedures, and operative duration were compared using the Mann–Whitney U test. Ablation-related complications were compared using the Chi-squared test.
Results
The mean diameter of HCC patients who received MWA was 2.4 ± 0.5 cm. For in-plane APPE, APPE was comparable between the two groups (p = 0.299). However, for the out-of-plane position, the APPE in the freehand group was higher than that in the RLP group (p = 0.027). The number of repositioning procedures was 0 (range, 0–0) for RLP-guided procedures and 3 (range, 2–5) for freehand procedures, showing a statistically significant difference between the two groups (p < 0.001). The mean operative duration for freehand procedures was 39 min, compared with 26 min for RLP-guided procedures, showing a significant difference (p = 0.013). No deaths or major complications were directly related to MWA. Minor complications in the freehand group were comparable with those in the RLP group (p = 0.313).
Conclusion
RLP guidance significantly reduces the number of antenna repositioning procedures in MWA and improves puncture accuracy for target HCC out-of-plane. In addition, the operative duration of robotic guidance was shorter than that of freehand guidance.
Introduction
Hepatocellular carcinoma (HCC) is the fifth most common malignancy resulting from hepatitis viral infections (hepatitis B virus [HBV] or hepatitis C virus [HCV]) and the third leading cause of cancer-related deaths globally [Citation1–3]. The development of ablation technology is of extensive value in the treatment of HCC and is considered the third major treatment option following surgical resection (SR) and liver transplantation (LT) [Citation4–6]. Among these ablation technologies, microwave ablation (MWA) is a well-received method owing to the higher intratumoural temperature and a more massive region of cell necrosis, with a comparable 5-year overall survival for early-stage HCC similar to SR [Citation7–9]. Unfortunately, the 5-year recurrence rate of approximately 35–50% after MWA remains a great challenge in clinical practice [Citation9].
The precise placement is a key step to an effective MWA procedure. The final position of the antenna entering the tumor plays a vital role in the complete ablation and control of recurrence. Currently, the guidance modalities of MWA mainly include the following two types: computer tomography (CT) guidance and ultrasound guidance [Citation10,Citation11]. For CT guidance, the radiation dose for patients on number of scans needed to both (1) localize/visualize the target lesion and (2) on the number of repositioning attempts needed to accurately place the MW antenna in the target lesion. Furthermore, relatively high radiation exposure to both patient and procedural personnel is expected. Moreover, multiple adjustments of the needle position under a CT scan also increase the risk of bleeding. Although it does not have the above problems in ultrasound guidance, the low spatial resolution and vulnerability to ribs or airborne organs will weaken the accuracy of puncture and ablation. Moreover, using both CT and US guidance together can help to improve antenna placement accuracy.
Needle navigation is improving along with the progress of materials and mathematics [Citation12–16], undergoing the process from single image guidance to multimodal image fusion guidance and even robotic navigation. Although CT- or ultrasound-guided freehand puncture is currently the most common method, there is a concern for both intraoperative bleeding risk and low operator experience reducing puncture accuracy. Therefore, several researchers have investigated the robotic guide system to assist interventional radiologists in a precise puncture. For example, Beyer et al. used a novel robotic system (MAXIO)-assisted thermoablation therapy for patients with liver cancer [Citation17]. Their results found that the robotic guided approach improved the accuracy of localizing the target tumor and reduced the radiation dose in patients compared with manual approaches. Recently, Wouter J. Heerink et al. designed a prospective, nonblinded randomized controlled trial that compared the accuracy and safety between robot-guided and freehand [Citation18], further suggesting that the robot-guided ablation approach had advantages, including convenient, fast, safe, and accurate performance.
Here, our study aimed to compare the accuracy, safety, and operative comfort of a self-designed RLPS guidance system for antenna placement versus freehand antenna placement for patients with HCC who underwent CT-guided MWA.
Materials and methods
Study design
This retrospective study protocol was approved by the institutional review board (approval number: 202066) of People’s Hospital of Jianyang City and was conducted following the principles of the 1975 Declaration of Helsinki. The requirement for written informed consent was waived because of the retrospective nature of this study.
From May 2020 to June 2021, a total of 105 patients with early-stage HCC (according to Barcelona Clinic Liver Cancer [BCLC] staging) who subsequently underwent computer tomography (CT)-guided percutaneous MWA were reviewed. HCC was diagnosed according to the guidelines of the American Association for the Study of Liver Diseases (AASLD) and the European Association for the Study of the Liver (EASL) [Citation19,Citation20]. Furthermore, some suspected cases were confirmed by imaging (CT or ultrasound)-guided needle biopsy. The patients with an early-stage HCC recruitment pathway are shown in . The inclusion criteria were as follows: (i) age 18–75 years old; (ii) Eastern Cooperative Oncology Group performance scores < 2 scores and Child–Turcotte–Pugh grade A or B; (iii) single tumor with maximum diameter < 3 cm; and (iv) absence of vascular invasion or extrahepatic metastases. The exclusion criteria were as follows: (i) history of other malignancies; (ii) those who underwent procedures combined with other treatments before MWA; (iii) severe coagulation disorders (i.e. prothrombin time > 25 s, prothrombin activity < 40%, and platelet count < 50 cells × 109/L); (iv) missing clinical and CT data; and (v) loss to follow-up after MWA.
The operative modalities (freehand and RLPS guidance) of MWA were selected per person according to block randomization. After screening according to the inclusion and exclusion criteria, 40 patients were included in our study (20 in freehand and 20 in RLPS guidance). The equipment and procedure of CT-guided MWA are shown in the Supplementary information. All ablative procedures were performed by two interventional radiologists (S. Z. and J. J. Z. who both had 10 years of experience on MWA).
The robotic architecture
Robotic-guided procedures were performed with the RapidNav Robotic Laser Positioning System (Robotic Laser Positioning System (RLPS); Readitec Medical Systems (Chengdu) Co., Ltd, China), which consists of a circular rail as the main structure parallel to the CT scanning plane (). This specified circular rail is installed on two bearings, which hold the circular rail and make it possible to rotate to match the pitch of CT gantry and multiplanar reformation (MPR) reconstruction. In this design, out-of-plane procedures can be guided accurately. There is a set of cross modules installed on a movable basement that can slide on the circular rail. This setting provides 400 mm of movement from left to right and 200 mm of movement from head to feet. A cross-line laser and a point laser are installed at the bottom level. Practitioners use a laser path to insert the needle. A linear track to hold the needle is also optional and can be installed under the bottom level for needle paths with large angles. With the RLPS installed in front/on the back of the CT, the distance between the CT scan plane and the circular rail rotating plane can be measured. To confirmed the gross tumor region, a physician will first perform a 5-mm enhanced CT scan to cover the liver and two extra 50 mm areas toward both the head and the feet during the procedure. The needle insertion path is planned on the treatment planning software (AccuSeed, provided by a third party). As the RLPS is connected with its planning software through an Ethernet cable, the needle path’s relative coordinates will be executed by the RLPS’s controlling processor and its four motors. Once all movements are done, the motors will be locked electrically. The laser lighted up for the practitioner to insert the needle. To place the needle on the same path as the laser, the practitioner must point the needle tip on the laser dot as projected on the patient’s skin. Then, the needle was moved to let the laser dot appear at the center of the needle’s tail. This is when the needle is on the same path as the point laser, and the RLPS guides the needle’s insertion.
Figure 2. The needle position system consists of a circular rail as the main structure that paralleled with the CT scanning plane. ① A circular orbit for assisting needle position; ② a semicircular supporting structure with control panel fixed to the CT bed; ③ a movable collimator for guidance on the axial CT images; ④ a laser cross-line.
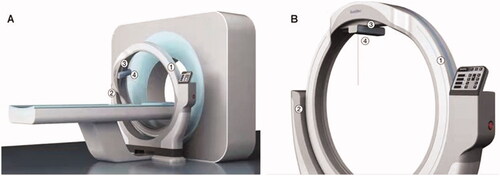
The operating process of robot-guided MWA
A supine or prone position was selected according to the intrahepatic lesion’s location in the liver, and local anesthesia with 1% lidocaine anesthesia were performed for all patients. On this basis, a vacuum mattress was used to avoid patient movement. The operations were performed with a 64–multidetector row CT system (Philips Medical Systems, Best, The Netherlands). The scanning layer was thick using 1 mm thin layers, and all patients underwent preoperative respiratory training before MWA. The flowchart of robot-guided MWA is shown in , which mainly included three parts as follows: (i) preablation planning; (ii) intraoperative navigation, and (iii) postablation assessment. First, a virtual antenna simulates the needle trajectory in the liver structure with an ellipsoidal virtual thermal field appearing at the arrow’s tip on the selected CT image. The thermal field shape is formed based on the time and power of the actual MWA process. According to the preablation planning, intraoperative navigation of the robot was performed as soon as. A laser beam with a fixed angle was used to guide the interventional radiologists to puncture, avoiding the collision with the ribs and major vessels. After the above operation, one tumor map was used to evaluate the complete ablation and ablation margin. The application scenario of robot-assisted CT-guided MWA for a patient with HCC is shown in .
Figure 4. A 56 years, male patients with HCC (the lesion located on the liver segment V, and the maximum tumor diameter is 2.4 cm) who underwent robotic position for antenna in CT-guided microwave ablation was shown. In order to show the target more clearly on the CT image, this patient received hepatic artery embolization before ablation, and the location of the tumor in the target area was determined by lipiodol deposition. (A) Pre-ablation planning; (B) robotic position; (C) the laser cross-line to determine entry point; (D) an interventional radiologist perform the puncture by the robot guidance; (E) the MW antenna was inserted into the tumor according to the pre-ablation planning (yellow arrow); (F) according to the artificial intelligence algorithm, the preoperative tumor shape (yellow arrow) is transferred intraoperatively; (G) the MW antenna was fixed, ablation was performed; (H) the ablation area was outlined on CT image; (I) the ablation area (yellow arrow) was clear and no recurrence was found 1 month after operation.
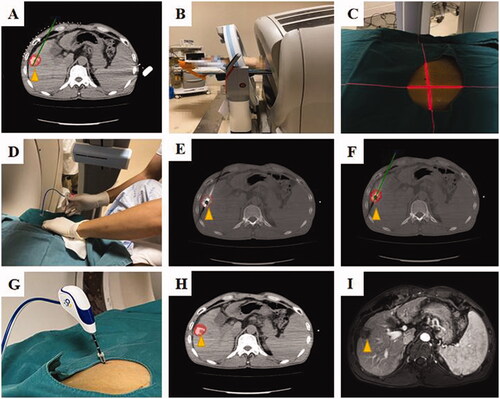
Follow-up and data collection
All patients were reviewed and evaluated as of the last follow-up date (30 October 2021). If a thorough MWA procedure was accomplished, then serum alpha-fetoprotein (AFP) and contrast-enhanced magnetic resonance images were examined again at 1 and 3 months after MWA and at approximately 3- to 6-month intervals. Incomplete ablation was defined as irregular peripheral enhancement in a scattered, nodular, or abnormal pattern around the ablative area. Technical success was defined as the absence of contrast enhancement on follow-up imaging in any liver area after MWA for one month [Citation21]. In this study, we collected various clinical variables related to HCC patients who underwent MWA. The characteristics of the patients and tumors included demographic variables (age, sex, etiology, cirrhosis, liver function), tumor features (maximal tumor diameter, location in liver segment, depth of tumor and important surrounding structure), ablation parameters (ablation duration and ablation power), and laboratory findings.
Outcome and comparisons
Based on the proposed measure method by Wouter J. Heerink et al., the operation was grouped into in-plane and out-of-plane [Citation18]. Measurements were performed by two radiologists with 10 years of experience (S. Z. and J. J. Z.). If there was a disagreement, a more senior expert (F. S.) made the final judgment. The microwave antenna with an angle greater than 5° from the axial plane was considered to be out of plane. In contrast, it is considered as in plane. Our study's outcome comparisons between the freehand and RLPS guidance groups included accuracy, safety, and operative comfort. The assessment of the accuracy of antenna positioning included (i) needle point error on skin (NPES); (ii) actual puncture point error (APPE); (iii) the number of repositionings, and (iv) angle error of the first puncture. The safety assessment included (i) radiation dose and (ii) ablation-related complications based on the International Working Group on Image-Guided Tumor Ablation standards of terminology and reporting criteria [Citation21]. The assessment of operative comfort included (i) operative duration and (ii) the NASA-tolerance load index (TLX) method. The measurement methods are shown in the Supplementary information.
Statistical analysis
All statistical analyses were performed using SPSS 23.0 (SPSS, Chicago, IL). Continuous variables are given as the mean ± standard deviation or median with interquartile range and were compared by Student’s t test or Mann–Whitney U test. Categorical variables are presented as frequencies with percentages and were compared by the Chi-squared test. All tests of significance were two-sided, and a p value < 0.05 was interpreted to carry statistical significance.
Results
Patient and tumor characteristics
A total of 40 consecutive patients with single HCC (8 females and 32 males; mean age, 55.2 ± 10.0 years) with a mean maximum tumor diameter of 2.4 ± 0.5 cm were screened in our study. Among them, 20 patients received MWA by freehand, and 20 patients received MWA by RLPS guidance. Specifically, five of the robot-guided approaches (in five patients, two had missing preablation data, two were technically impossible due to steep cranial angulation and one patient refused treatment at the final time) were excluded from this study. The baseline characteristics of the patients with HCC between the freehand and RLPS guidance groups are summarized in . The distribution of all clinical variables showed no significant difference between the two groups.
Table 1. Baseline characteristics of patients with HCC who underwent MWA.
Accuracy comparison between freehand and RLPS guidance
In this study, all MWA procedures were conducted for early-stage HCC (single HCC with diameter < 3 cm) to confirm the accuracy of the robot-guided approach. shows the comparison of accuracy between the freehand group and the RLPS guidance group. According to in-plane and out-of-plane procedures, the enrolled patients were stratified. Twelve of the 20 freehand procedures were out of plane, compared with fourteen of the 20 robot-guided procedures. For the in-plane, the median NPES was 0.5 mm in the freehand group and 0.4 mm in the RLPS guidance group, showing no significant difference (p = 0.232). Similarly, for the out-of-plane, the median NPES was 0.4 mm in the freehand group and 0.3 mm in the RLPS guidance group, showing no significant difference (p = 0.547). For the in-plane guidance group, the median APPE was 4.2 mm in the freehand group and 3.8 mm in the RLPS guidance group, showing no significant difference (p = 0.299). However, for the out-of-plane, the median APPE was 4.8 mm in the freehand group and 3.9 mm in the RLPS guidance group, showing a significant difference (p = 0.027). There was no statistically significant difference in the angle error of the first puncture between the freehand group and RLPS guidance group in the in-plane (all, p > 0.05). However, for the out-of-plane, the horizontal (x–y) of the freehand group was significantly higher than that of the RLPS guidance group (p = 0.012). Moreover, the complete ablation rate was 100% in both groups.
Table 2. The comparison of technical outcomes between freehand and robot.
Operational comfort comparison between freehand and RLPS guidance
compares operative comfort between the freehand group and RLPS guidance group. Overall, the depth from the skin of the target tumor was 5.7 ± 0.8 cm in the freehand group and 5.5 ± 1.0 cm in the RLPS guidance group, showing no significant difference (p = 0.559). The mean operative duration for freehand procedures was 39 min, compared with 26 min for robotic procedures (p = 0.013). The total procedure duration (operative duration plus ablation duration) was 1 h 40 min and 1 h 16 min for freehand and RLPS guidance, respectively (p = 0.776). The number of repositioning procedures was 0 (range, 0–0) for robot-guided procedures and 3 (range, 2–5) for freehand procedures. There was a statistically significant difference between the two groups (p < 0.001). Accordingly, the radiation dose of the freehand group was significantly higher than that of the RLPS guidance group (p < 0.001). Moreover, there was a statistically significant difference in the NASA-TLX score between the freehand and RLPS guidance groups, suggesting that the MWA procedure was more comfortable with less stress when using RLPS guidance. The technical success rate in the freehand group was 95% (19/20), possibly because a female patient had a tumor abutting the gallbladder and the portal vein in liver segment V and finally obtained an ablation margin of less than 5 mm. However, the technical success rate in the RLPS guidance group was 100% (20/20).
Table 3. Operational comfort and safety comparison between freehand and robot.
Post-ablation complications
No deaths or major complications were directly related to MWA in the two groups. Minor complications were observed in 6 of the 20 patients (30.0%) in the freehand group and 4 of the 20 patients (20.0%) in the RLPS guidance group (). There was a statistically significant difference (p = 0.313). Among them, six patients, including one with diarrhea, two with fever, one with intrahepatic bleeding, one with mild abdominal pain, and one with right shoulder back pain, were found in the freehand group within 12 days post-ablation. Four patients, including one with diarrhea, two with fever, one with mild abdominal pain, and one with vomiting, were included in the RLPS guidance group within 18 days post-ablation.
Discussion
CT-guided MWA is widely used as a minimally invasive therapeutic procedure for HCC. However, CT guidance is a time-consuming procedure, even if experienced interventional radiologists perform it. In addition, significant radiation exposure in HCC patients occurs during the process of repositioning by CT guidance. Preablation planning plays a vital role in ablative effects and outcomes [Citation22–24]. Still, it is difficult for inexperienced interventional radiologists to master the precise and safe puncture route and ablation scheme. Therefore, the development of robotic guidance platforms to overcome these limitations of CT guidance remains urgently required.
Over the past decade, many interventional robots for biopsy or ablation have been developed and designed. For example, Hyung Jin Won et al. used a master–slave type robot for CT-guided needle intervention using an optical tracking system [Citation25]. This robot can perform needle placement outside the body accurately and quickly. However, liver breathing movement remains a great challenge. Ping Liang et al. designed and developed a multimodality imaging-compatible insertion robot for ablation, which can effectively solve the phenomenon of liver movement and deformation during the process of puncture, thus improving the puncture accuracy [Citation26]. However, the whole procedure takes much time. Drawing on the experience of these experts, our self-developed robotic system named RLPS-assisted CT-guided ablation has many advantages, including rapid installation, convenient operation, accurate guidance and safe control. To test the robot’s efficacy, we performed a robot-guided MWA procedure for HCC in 20 patients and compared the outcomes of the freehand group and the RLPS guidance group.
In this study, we compared the accuracy, safety, and comfort between a freehand group and a RLPS guidance group. First, the depth of the target tumor was comparable between the freehand group and RLPS guidance group. On this basis, we found that the NPES was not significant between the two groups (0.5 mm versus 0.4 mm in plane and 0.4 mm versus 0.3 mm out-of-plane). The APPE in the in-plane was also not significantly different between the two groups. Still, the APPE and horizontal (x–y) angle error of the first puncture were significantly different in the out-of-plane, suggesting that RLPS guidance had a more precise puncture path than freehand guidance. Our robotic guidance system (RLPS) obtained a comparable accuracy (APEE, 3.9 mm of out of plane) compared to previous studies. Previously, L. P. Beyer et al. reported the MAXIO robotic system (Perfint Healthcare, Chennai, India) for needle positioning in 34 patients with liver tumors in 2015, and the mean puncture deviation was 3.1 ± 2.5 mm [Citation17]. Engstrand et al. used the CAS-One system (CAScination, Bern, Switzerland) for CT-guided percutaneous MWA of patients with 28 liver tumors, and the lateral accuracy was 4.0 mm [Citation27]. Recently, Wouter J. Heerink et al. designed a prospective randomized controlled trial. They used the needle positioning system (NPS; DEMCON Advanced Mechatronics) to guide MWA for liver tumors, and the lateral targeting error was 5.9 ± 2.9 mm for robotic procedures, outperforming freehand [Citation18].
Furthermore, a larger angle error of the first puncture by freehand than RLPS guidance results in more repositioning sessions. Therefore, the number of repositions in the freehand group was greater than that in the RLPS guidance group. Owing to repositioning sessions by freehand, we hypothesized that as the needle is randomly adjusted in the liver parenchyma, the target tumor is biased from its initial position. Less repositioning is helpful to improve the APPE and reduce the incidence of intrahepatic hemorrhage. Similarly, the patients in the freehand group received more radiation doses than those in the RLPS guidance group. Moreover, no deaths or major complications were directly related to MWA in the two groups. Only several minor complications occurred after MWA, and the primary reason may be related to heat-induced stimuli rather than a puncture. Finally, complete ablation was obtained for all patients with small HCC between the two groups. These results suggest greater safety for HCC patients using RLPS guidance.
In our study, we also evaluated the operative duration and the subjective attitude toward the procedure using the NASA-TLX method. The tumor size and depth were equal in both groups. The operative duration using freehand was 39 min longer on average than robotic guidance (20 min, p = 0.013). This can be attributed to repositioning sessions for freehand. It is important to note that we performed breathing training for each patient before treatment to reduce the impact of the liver breathing movement, and this time consumption was not included in our study. However, it did not significantly affect the total procedure times (1 h 40 min and 1 h 16 min for freehand and robotic procedures, respectively). Moreover, the NASA-TLX score suggests that robotic guidance is much easier to navigate the procedure, and it is a great experience for interventional radiologists. This technique makes the MWA process straightforward, especially for interventional radiologists who lack experience with MWA.
There are some limitations to our study. First, the risk of selection bias is unavoidable in observational studies. Second, the study cohort was a single-center, retrospective study, and the sample size was relatively small. Multicentre, large cohort, and prospective studies are necessary to verify our results. Finally, at present, there is no universally recognized evaluation criterion for precise measurement of puncture deviation, so there may be some imbalances in the inclusive population of the two treatment groups, which may cause biased comparative results of outcomes.
In conclusion, the RLPS guidance system in percutaneous MWA of HCC is a fast, reliable and effective alternative to manual CT guidance. The RLPS guidance system can increase precision and reduce radiation dose for interventional radiologists and HCC patients without increasing complication risk. We would like to state that robot assistance should be thoroughly evaluated for other CT-guided interventions, such as biopsies or other ablation techniques, to make the best of this technique and minimize radiation exposure to patients and interventional radiologists.
Author contributions
Y. R. participated in the data analysis and drafted the manuscript. G. S. W. conceived of the study and carried out the editorial support for this manuscript. S. Z., J. J. Z., and Q. G. participated in the ablation procedure. X. X. C. and F. H. Q. provided assistance in data analysis. F. S. reviewed and confirmed the manuscript. All authors read and approved the final manuscript.
Supplemental Material
Download PDF (166.7 KB)Disclosure statement
No potential conflict of interest was reported by the author(s).
Additional information
Funding
References
- Forner A, Reig M, Bruix J. Hepatocellular carcinoma. Lancet. 2018;391(10127):725–1314.
- Siegel RL, Miller KD, Jemal A. Cancer statistics, 2020. CA A Cancer J Clin. 2020;70(1):7–30.
- Sung H, Ferlay J, Siegel RL, et al. Global cancer statistics 2020: GLOBOCAN estimates of incidence and mortality worldwide for 36 cancers in 185 countries. CA Cancer J Clin. 2021;1:1–5.
- Benson AB 3rd, D'Angelica MI, Abbott DE, et al. NCCN guidelines insights: hepatobiliary cancers, version 1.2017. J Natl Compr Canc Netw. 2017;15(5):563–573.
- Marrero JA, Kulik LM, Sirlin CB, et al. Diagnosis, staging, and management of hepatocellular carcinoma: 2018 practice guidance by the American Association for the Study of Liver Diseases. Hepatology. 2018;68(2):723–750.
- Ozer Etik D, Suna N, Boyacioglu AS. Management of hepatocellular carcinoma: prevention, surveillance, diagnosis, and staging. Exp Clin Transplant. 2017;15(Suppl 2):31–35.
- Yu J, Yu XL, Han ZY, et al. Percutaneous cooled-probe microwave versus radiofrequency ablation in early-stage hepatocellular carcinoma: a phase III randomised controlled trial. Gut. 2017;66(6):1172–1173.
- Yu J, Liang P. Status and advancement of microwave ablation in China. Int J Hyperthermia. 2017;33(3):278–287.
- Takahashi H, Kahramangil B, Berber E. Local recurrence after microwave thermosphere ablation of malignant liver tumors: results of a surgical series. Surgery. 2018;163(4):709–713.
- An C, Cheng Z, Yu X, et al. Ultrasound-guided percutaneous microwave ablation of hepatocellular carcinoma in challenging locations: oncologic outcomes and advanced assistive technology. Int J Hyperthermia. 2020;37(1):89–100.
- Xie L, Cao F, Qi H, et al. Efficacy and safety of CT-guided percutaneous thermal ablation for hepatocellular carcinoma adjacent to the second porta hepatis. Int J Hyperthermia. 2019;36:1122–1128.
- Hamzé N, Peterlík I, Cotin S, et al. Preoperative trajectory planning for percutaneous procedures in deformable environments. Comput Med Imaging Graph. 2016;47:16–28.
- Sutter O, Fihri A, Ourabia-Belkacem R, et al. Real-time 3D virtual target fluoroscopic display for challenging hepatocellular carcinoma ablations using cone beam CT. Technol Cancer Res Treat. 2018;17:1533033818789634–1533033818789634.
- Petersen BK, Yang J, Grathwohl WS, et al. Deep reinforcement learning and simulation as a path toward precision medicine. J Comput Biol. 2019;26(6):597–604.
- Dey N, Li S, Bermond K, et al. Multi-modal image fusion for multispectral super-resolution in microscopy. Proc SPIE Int Soc Opt Eng. 2019;10:109–126.
- Chun KR, Schulte-Hahn B, Windhorst V, et al. Value of robotic navigation and ablation. Minerva Cardioangiol. 2010;58(6):649–656.
- Beyer LP, Pregler B, Niessen C, et al. Robot-assisted microwave thermoablation of liver tumors: a single-center experience. Int J Comput Assist Radiol Surg. 2016;11(2):253–259.
- Heerink WJ, Ruiter S, Pennings JP, et al. Robotic versus freehand needle positioning in CT-guided ablation of liver tumors: a randomized controlled trial. Radiology. 2019;290(3):826–832.
- European Association for the Study of the Liver. EASL clinical practice guidelines: management of hepatocellular carcinoma. J Hepatol. 2018;69:182–236.
- Heimbach JK, Kulik LM, Finn RS, et al. AASLD guidelines for the treatment of hepatocellular carcinoma. Hepatology. 2018;67(1):358–380.
- Ahmed M, Solbiati L, Brace CL, et al. Image-guided tumor ablation: standardization of terminology and reporting criteria-a 10-year update. Radiology. 2014;273(1):241–260.
- Xiaoyu T, Pengqian Y, Kah-Bin L, et al. Robust path planning for flexible needle insertion using Markov decision processes. Int J Comput Assist Radiol Surg. 2018;50:124–128.
- Zhang M, Zhou Z, Wu S, et al. Simulation of temperature field for temperature-controlled radio frequency ablation using a hyperbolic bioheat equation and temperature-varied voltage calibration: a liver-mimicking phantom study. Phys Med Biol. 2015;60(24):9455–9471.
- Liu S, Xia Z, Liu J, et al. Automatic multiple-needle surgical planning of robotic-assisted microwave coagulation in large liver tumor therapy. PLoS One. 2016;11(3):*e0149482.
- Won HJ, Kim N, Kim GB, et al. Validation of a CT-guided intervention robot for biopsy and radiofrequency ablation: experimental study with an abdominal phantom. Diagn Interv Radiol. 2017;23(3):233–237.
- Li D, Cheng Z, Chen G, et al. A multimodality imaging-compatible insertion robot with a respiratory motion calibration module designed for ablation of liver tumors: a preclinical study. Int J Hyperthermia. 2018;34(8):1194–1201.
- Engstrand J, Toporek G, Harbut P, et al. Stereotactic CT-guided percutaneous microwave ablation of liver tumors with the use of high-frequency jet ventilation: an accuracy and procedural safety study. Am J Roentgenol. 2017;208(1):193–200.