Abstract
Objective
The in-house developed 70 MHz AMC-4 locoregional hyperthermia system has been in clinical use since 1984. This device was recently commercialized as the Alba 4D (Medlogix®, Rome, Italy), with a similar geometrical 4-waveguide design. At the time of this study a hybrid Alba 4D was installed at our center, which incorporated elements of the AMC-4. This study aims to compare clinical performance of both devices.
Methods
During one year after clinical acceptance of the hybrid Alba 4D, both devices were used for treatment delivery in patients scheduled for locoregional hyperthermia. Each patient started with the AMC-4, next sessions were allocated to either device. Possible differences between Alba 4D and AMC-4 sessions in power, achieved temperature T0, T10, T50, T90, T100, treatment time and complaints per session, were evaluated using linear mixed models (LMMs) for repeated measures with patient as random effect.
Results
From March 2018 to April 2019, eleven patients with cervical, pancreatic, vaginal carcinoma and uterine leiomyosarcoma received 27 locoregional hyperthermia sessions with the Alba 4D and 34 sessions with the AMC-4. Median number of sessions per patient was 5 (range 3–13). Treatment results for both devices were not significantly different: T50 was 40.5 ± 1.0 °C vs. 40.8 ± 0.7 °C, applied power was 500 ± 79 W vs. 526 ± 108 W, for the Alba 4D vs. AMC-4, respectively.
Conclusion
Results of the first patients treated with the hybrid Alba 4D demonstrated comparable clinical performance of the Alba 4D and AMC-4 locoregional hyperthermia systems, and both devices are expected to yield similar favorable clinical results.
Introduction
Hyperthermia aims at heating the tumor to 40 − 44 °C for 1 h. Effectiveness of radiotherapy and chemotherapy can be enhanced significantly by adding hyperthermia to the standard treatment schedule, without increasing toxicity. This benefit of locoregional heating using phased-array systems has been demonstrated for various tumors in the pelvis and abdomen, among which cervical, rectal and bladder cancer [Citation1–8].
Deep-seated tumors in the pelvis and abdomen that are located more than four centimeter (cm) from the skin surface, can be heated adequately with radiative locoregional hyperthermia [Citation9,Citation10]. Radiative locoregional systems are phased arrays of 4 − 12 antennas positioned around the pelvis or abdomen of the patient, operating at 70 − 140 MHz. Electromagnetic energy from the antennas is coupled into the patient via water boluses. Phase-amplitude steering allows focusing of the electromagnetic energy deposition at the tumor location. Schneider et al. [Citation11] evaluated the performance of several radiative locoregional heating systems, which showed that all devices were able to realize a similar central specific absorption rate (SAR) focus, which could also be moved in any direction by phase steering. Presently, several radiative locoregional hyperthermia systems are in clinical use, for example, the BSD-2000 with Sigma 60 applicator (Pyrexar, Salt Lake City, UT), the BSD-2000 with Sigma Eye applicator (Pyrexar, Salt Lake City, UT), the AMC-4 and the AMC-8 (AMC, Amsterdam, the Netherlands). At the Amsterdam UMC, location Academic Medical Center (AMC) the 70 MHz AMC-4 locoregional hyperthermia system has been in clinical use since 1984 () [Citation12]. The most recent version can be used either as a double ring of eight waveguides (AMC-8) or as a single ring of four waveguides (AMC-4) [Citation13]. The AMC-4 system has been applied in the hyperthermia treatment of more than 1000 patients who received in total more than 5000 treatment sessions. Recently, the AMC-4 system has been commercialized as the Alba 4D (Medlogix®, Rome, Italy) utilizing the same geometrical four-waveguide design (). At the time of this study, a hybrid Alba 4D that incorporates elements of the AMC-4 system was clinically available at our institute.
Figure 1. The in-house developed AMC-4/8 system (left), which can be used as a single ring of four antennas (AMC-4) or two rings of eight antennas (AMC-8) and the Alba 4D (right; Medlogix®, Rome, Italy) locoregional hyperthermia systems.
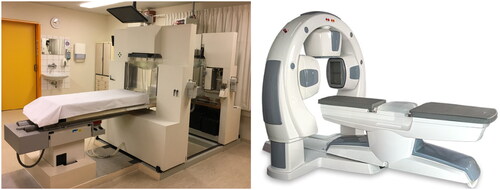
The heating performance of the hybrid Alba 4D was compared to the performance of the AMC-4 using patient-mimicking phantoms, where the measured electric field distributions confirmed that the phase control of the focal point was similar for both devices [Citation14]. This shows that the device is technically capable of achieving focused and controlled heating in a target volume, and thus the Alba 4D can be considered as a suitable locoregional hyperthermia system as defined in the European Quality Assurance guidelines [Citation10,Citation15]. As a next step, this study aims to evaluate the clinical performance of the hybrid Alba 4D locoregional hyperthermia system compared to the AMC-4 locoregional hyperthermia system in a prospective cohort of 11 patients treated with both devices. Thus, in this study, we effectively investigated the impact of the differences in waveguides, water boluses and physical structure of both devices.
Materials and methods
After clinical acceptance of the Alba 4D system [Citation14], we have treated patients with locoregional hyperthermia on both the AMC-4 and Alba 4D during one year. Patients who were scheduled for locoregional hyperthermia were asked for their participation and gave informed consent. Patients treated with hyperthermia and simultaneous chemotherapy were excluded; for these patients, it is mandatory in our institute to have a dedicated bathroom and shower adjacent to the treatment room, which was not the case in the temporary Alba 4D room. Patients were scheduled to receive treatment sessions with both the AMC-4 and the Alba 4D. For each patient, the first session was with the AMC-4 and the next sessions were allocated to either device by the researcher. We aimed to use a repeated measures design with different sequences. To prevent bias patients were randomized for a variety of possible treatment sequences with the AMC-4 (1) or Alba 4 D (2): 11122; 11212; 11221; 12211; 12121; 12112.
We investigated whether sessions with the Alba 4D and AMC-4 differed in applied power, achieved temperature and treatment-related complaints. Furthermore, we evaluated the experiences of the patients and hyperthermia staff with both devices. All subjects gave written informed consent for participation in the study. The study was conducted in accordance with the Declaration of Helsinki, and we received a waiver from the Medical Ethics Committee of the AMC to conduct this clinical comparison study (W16_370 #16.435).
Locoregional hyperthermia systems
Both the AMC-4 system and Alba 4D system () consist of a ring of four 70 MHz waveguides with an aperture of 20 × 34 cm. The Alba 4D system used during this study was a hybrid AMC-4/Alba 4D system where the AMC-4 software, thermometry, direct digital synthesis (DDS), radiofrequency (RF) generator (SSB Electronic, Iserlohn, Germany) and amplifiers (Restek®, Italy, Rome) were used. Thus, the system efficiency is similar. The waveguides of both AMC-4 and Alba 4D are connected to an eight channel DDS-based phase and amplitude-controlled RF generator system. The final solid state 70 MHz amplifiers each provide 500 Watt (W) maximum output power. Typical clinical power levels vary between 100 and 200 W per waveguide, resulting in a total power of 400–800 W. A double-slug tuner between the amplifier and antenna is used for tuning [Citation14]. The phase accuracy is 3° and the power accuracy is 10 W [Citation13].
The AMC-4 utilizes four water boluses (one closed bottom bolus, three open) filled with circulating demineralized water, which are positioned between each waveguide and the patient’s skin to cool the skin and to improve electric coupling without absorbing power in the bolus water. The Alba 4D utilizes two closed water boluses filled with circulating demineralized water, the bottom bolus and one large U-shaped bolus that couples the energy of the left, right and top waveguide. Both systems allow manual adjustment of the waveguide positions to ensure 5 cm water bolus between the patient and waveguides for each individual patient. With 5 cm water bolus, patients as thin as 18 cm in the ventral-dorsal direction can fit inside the AMC-4, versus patients as thin as 16 cm in the Alba 4D. Depending on the patient size, three different top boluses can be selected. The boluses are 40 cm in the cranio-caudal direction, the width of these boluses is 90, 102.5 and 115 cm for the small (S), medium (M) and large (L) bolus, respectively. The water circulating in the boluses is kept at approximately 13 °C to provide skin cooling.
Hyperthermia treatment
Hyperthermia was given once a week during the period of external radiotherapy or chemotherapy, within 1 h after irradiation or the day after chemotherapy. Thermometry catheters were introduced in the natural cavities, such as the bladder, vagina, rectum and duodenum, depending on the tumor site. Multisensor copper constantan thermocouple probes (Volenec CS, Hradec Králové, Czech Rebuplic) were inserted in these catheters. All probes had 14 sensors. Spacing varied from 20 mm (duodenal probes) to 5 mm (bladder, vagina, rectum). Temperatures were measured every 30 s after a 5 s power off to avoid electromagnetic disturbance [Citation16]. Patients were treated in supine position. Patients were positioned in cranio-caudal direction such that the tumor center was aligned with the aperture midplane. In case of the hybrid Alba 4D, lasers were used to enable accurate positioning. Initial phase settings of the first session were determined by the hyperthermia operator based on the patient anatomy and her experience in treating patients with locoregional hyperthermia. Directly before or after the first hyperthermia session a CT scan was made in hyperthermia treatment position on a water bolus and mattresses [Citation17]. The tumor was delineated by a radiation oncologist, other tissues were automatically segmented as bone, muscle, fat and air based on Hounsfield units. Hyperthermia treatment planning was performed using our in-house developed software Plan2Heat [Citation17]. Electromagnetic fields were calculated for each individual antenna and combined using superposition. By solving the Pennes bio heat equation at steady state the temperature distribution in the patient was calculated [Citation17]. The optimal phase settings for focusing to the target location as determined by hyperthermia treatment planning were used as the initial phase settings for the second and consecutive hyperthermia sessions. This initial phase optimization mimics a robust phase sweep optimization procedure, which determines pair-wise the optimal phase per waveguide, using the top waveguide as a reference (i.e., phase 0°) [Citation18,Citation19]. During treatment, adaptive treatment planning is available to find alternative settings in case of hot spots, to effectively suppress the hot spot temperature and maintain adequate tumor heating [Citation20].
At the start of each treatment session, the focusing ability of the phase settings was verified using temperature rise measurements after a 60 s power pulse of 600 W equally divided over 4 antennas. Three different phase settings were applied, i.e., the proposed phase setting and the setting with a + 40° and −40° shift in the direction of the bottom antenna with respect to the top antenna. The phase setting with the steepest temperature rise in the tumor, while maintaining acceptable temperature levels in the normal tissue, was applied during the session [Citation14,Citation17].
Patients were carefully instructed to report any unpleasant sensation which might be suggestive of hotspots, such as a burning sensation, a feeling of pressure, or any pain. Treatment settings, such as power and (rarely) phase, were adjusted depending on observed temperatures and information from the patients. Power outputs were increased up to the tolerance of the patient. The aim was to continue treatment for 60 min after half of the tumor-indicative temperature sensors had reached 41 °C, or for a maximum duration of 90 min [Citation7,Citation17].
Statistical analysis
The following treatment characteristics were evaluated for sessions with the Alba 4D and AMC-4: achieved invasive temperature and average applied power. The achieved temperature distribution was characterized using the T0 (maximum), T10, T50, T90 and T100 (minimum) of all invasive temperature sensors, calculated with Matlab® version R2021a (MathWorks, Natick, MA). The T10, T50 and T90 being the temperatures exceeded by 10, 50 and 90% of all measurement points during the steady state of the hyperthermia session, respectively. We evaluated patient complaints during treatment. Furthermore, we assessed the experiences of patients and staff with both devices. Differences between the Alba 4D and AMC-4 in terms of these treatment characteristics were tested using linear mixed models (LMMs) for repeated measures. Potentially, the temperature can be influenced by different patient characteristics (age, perfusion, BMI, etc.), but also by the week of treatment (patients get tired, or patient conditions improve during treatment). Therefore, treatment characteristics may vary between patients and treatments, and we used in all mixed-effect models a random intercept for patient and random slope for treatment week. Parameter estimates and 95% Wald confidence intervals (CIs) were reported for fixed effects. We used R version 3.3.1 (R Foundation, Vienna, Austria) with package lme4 version 1.1-21 (Vienna, Austria) to perform the statistical tests and to estimate the parameters in the mixed-effect models.
Results
From March 2018 to April 2019, thirteen patients signed the informed consent form. In total, eleven patients did receive hyperthermia treatment with both locoregional hyperthermia systems. Two patients did not receive Alba 4D treatments as planned because at that moment not all hyperthermia staff had been adequately trained for the Alba 4D, and because of technical issues with the Alba 4D or thermometry during that session. Only the results of the patients treated with both locoregional hyperthermia systems are reported.
Eleven patients received in total 61 locoregional hyperthermia treatment sessions; 34 sessions with the AMC-4 and 27 sessions with the Alba 4D (). Although we aimed to randomize sessions between the two devices, due to technical issues with either the AMC-4, Alba 4D or thermometry, sessions in four patients were changed to the other device on the day of treatment (Patient 1, 4, 5 and 9). Patient 1 was the first patient on the Alba 4D, and with her informed consent all hyperthermia RTTs were clinically trained on the Alba 4D during her treatment sessions, which explains the relatively high number of Alba 4D sessions. Patient 3 turned out to be too thin for the AMC-4, e.g., the minimum distance that could be achieved between the top waveguide and the patient was too large, therefore, we continued treatment on the Alba 4D after consultation with her treating radiation oncologist.
Table 1. Overview of treatments per patient with the AMC-4 (grey) and Alba 4D (blue) locoregional hyperthermia systems.
Patients were treated with radiation therapy and hyperthermia for cervical carcinoma (n = 6), vaginal carcinoma (n = 2) and leiomyosarcoma of the uterus (n = 1), and two patients received two-weekly FOLFIRINOX chemotherapy the day before hyperthermia treatment for pancreatic carcinoma (Patient 1 and 11). The number of sessions per patient varied from 3 to 13 treatments (median 5).
Treatment week was not significant in the models; therefore, the final models did not include a random slope for treatment week. None of the treatment characteristics was significantly different between the two devices (; and ). We found no relationship between applied power and achieved temperature (). When we analyzed the results per patient, we found that in total five patients had on average a slightly higher T90 with the Alba 4D and six patients with the AMC-4. When analyzing the achieved temperature over time, we found no significant evidence that indicated a learning curve of the Alba 4D (R2 was 0.030 and 0.083 for T50 and T90, respectively).
Figure 2. The applied power per patient for each hyperthermia treatment session with the Alba 4D (blue) and the AMC-4 (grey) locoregional hyperthermia systems.
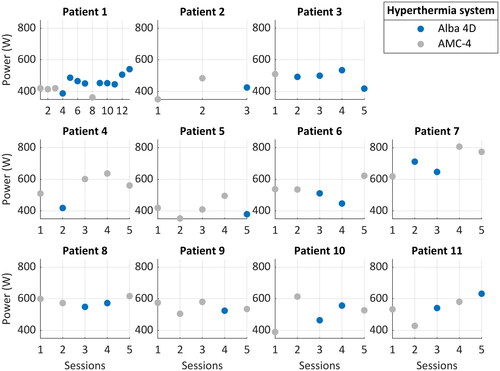
Figure 3. The achieved intraluminal T90 for each patient during the treatment sessions. Treatments with the Alba 4D are depicted in blue and treatments with the AMC-4 locoregional hyperthermia system in grey.
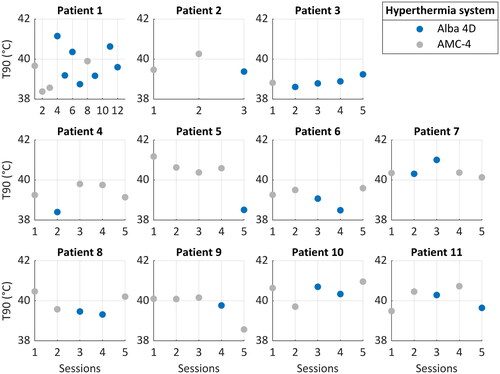
Figure 4. The applied power versus the achieved intraluminal T90. Each patient has a unique symbol; the color indicates the locoregional hyperthermia system: Alba 4D in blue, AMC-4 in grey. For both devices the mean applied power and achieved T90 are displayed with dashed lines.
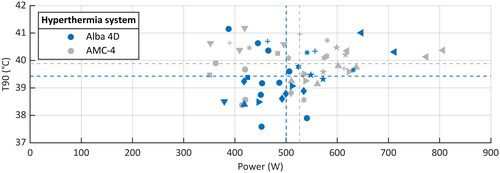
Table 2. Treatment characteristics presented as mean ± SD or median (range).
Per treatment, a median of one treatment complaint occurred per treatment session, with no significant difference between the devices (p = 0.34). Most treatment-related complaints were caused by fringing fields or by the supine treatment position in the devices, often caused by preexisting lower back pain. Fringing fields, i.e., local high field strengths caused by constraining the field lines at the edge of the water bolus, occur at the edges of the coupling water boluses and can cause superficial hotspots [Citation21]. Complaints caused by fringing fields can be solved by placing washcloths or towels on the affected area, or by adding additional water boluses, while complaints from the supine treatment position could be solved with adding pillows, knee support or pain medication. Most complaints could be solved with phase-amplitude steering (n = 31) or with medication (n = 2). Fourteen treatment sessions were stopped prematurely; all 13 sessions in Patient 1 on her request (27 ± 7 min) and the final session in Patient 5 (4 min). A premature stop could not be attributed to the use of either device.
The experience of both patients and staff was positive. Some patients had a preference for the AMC-4 system, others for the Alba 4D. The set-up time of the Alba 4D was slower than the AMC-4. The stated advantages and disadvantages are shown in .
Table 3. Advantages and disadvantages mentioned by patients and staff members.
Discussion
In this article, we report on the first 11 patients that were treated with the Alba 4D locoregional hyperthermia system, which is a new commercially available locoregional hyperthermia system. The Alba 4D used in this study was a hybrid Alba 4D/AMC-4 device, therefore, the main conclusions only concern the influence of the physical structure, waveguides and water boluses on the clinical treatment. We found no difference in clinical performance between the in-house designed AMC-4 and the hybrid Alba 4D. Therefore, it is safe to assume that both devices have similar power deposition and phase focusing in patients, and thus will likely yield similar favorable clinical results.
None of the treatment characteristics was significantly different for the two devices. Treatments with the Alba 4D tended to be performed with slightly less power and tended to result in slightly lower achieved temperatures than treatments with the AMC-4. It is likely that the radiotherapy technologists (RTTs) were more cautious giving treatments with the new hybrid Alba 4D system, and therefore also applied less power.
Although we aimed to randomize treatments between the two devices, in five patients, we changed from the planned device to the other device on the day of treatment. Only in Patient 3, this was a well-considered decision. The other changes in device were caused by unforeseen events, like technical issues with the device or due to a broken thermocouple probe used at the Alba 4D. This may have some influence on the results; however, we do not expect this to affect the main conclusion.
A major difference between the two devices is the bolus design. The AMC-4 has four water boluses, one closed and three open. The left, right and top water boluses are open at the top side, allowing the water to move with breathing. The Alba 4D utilizes two water boluses, both closed. The U-shaped top bolus couples the left, right and top antenna into the patient. Different widths were available for the top bolus, choosing a larger bolus was generally better for coupling the electromagnetic energy into the patient and for patient comfort. There was no indication that more or less fringing fields occurred at the edge of the water bolus of the Alba 4D compared to the AMC-4. The larger bolus size of the Alba 4D did result in longer filling times of the water boluses of the Alba 4D (∼5 min) compared to the AMC-4 (∼2 min), slightly increasing the overall set-up time of the Alba 4D. In the final commercial version of the Alba 4D the filling time of the top bolus was reduced (to ∼3 min). We attribute the longer set-up time of the Alba 4D that was experienced by patients and staff members mostly to the longer filling of the water boluses of the Alba 4D, however the learning curve for the Alba 4D might also contribute to the longer set-up time. Another major difference is that the AMC-4 can be extended to a double ring of eight waveguides (AMC-8) [Citation13]. Phantom experiments and simulations showed that the SAR distribution of the AMC-8 was more favorable than the AMC-4 [13]. Clinically this difference was shown to lead to fewer hotspots and thus potentially to higher tumor temperatures compared to the AMC-4 [Citation22]. Treatment with the AMC-8 is clinically indicated in, for example, very large and/or elongated tumors in the pelvis or abdomen and peritoneal carcinomatosis [Citation23].
In this study, a hybrid version of the Alba 4D system was tested. The AMC-4 software, thermocouple thermometry, RF generator and amplifiers were integrated in the hybrid Alba 4D, resulting in similar system efficiency. Therefore, power and treatment results could be reliably compared. After finishing this study, the final commercial version of the Alba 4D was installed, incorporating a new RF generator, amplifiers, thermocouple thermometry and software. Modest deviations in output amplitude of RF generators and amplifiers will not impact the clinical performance since treatment delivery is primarily steered based on the temperatures registered during treatment. Since new software could potentially influence treatment results, we performed extensive acceptance testing including phantom tests to determine the E-field and temperature distributions. No differences in power deposition or temperature distribution were observed when comparing the hybrid Alba with the AMC-4 software to the final commercial Alba 4D system. The final commercial Alba 4D showed similar E-field and temperature distributions as the hybrid Alba 4D for a central and shifted focus. This indicates that the new RF generator, amplifiers and software of the final commercial Alba 4D do not significantly alter power deposition patterns compared to the hybrid system as clinically evaluated in this study. Measurements of the phase and power in the treatment mode showed acceptable small deviations (max 2° and max 10% (200 W per antenna), respectively). The measured accuracy of the Alba 4D thermometry was within 0.2 °C, when the thermometry device was on for >8 h. A manuscript focusing on the results of the extensive clinical acceptance tests of the final commercial Alba 4D is in preparation.
Feedback based on experiences of patients and staff members has been provided to the manufacturer of the Alba 4D. Improvements, such as adding additional fans directed to the feet and quicker filling of the water boluses, have been implemented in the final commercial version of the Alba 4D. This final commercial version was installed at Amsterdam UMC in February 2021, replacing the Hybrid version. Keeping both devices in operation to repeat a comparative evaluation of clinical performance was unfortunately not feasible due to space and staff limitations under the COVID-19 conditions at that time. However, it would be counterintuitive to assume that the technical improvements in the Alba 4D would have resulted in a worse clinical performance.
Disclosure statement
No potential conflict of interest was reported by the author(s). The authors alone are responsible for the content and writing of the article.
Additional information
Funding
References
- Colombo R, Da Pozzo LF, Salonia A, et al. Multicentric study comparing intravesical chemotherapy alone and with local microwave hyperthermia for prophylaxis of recurrence of superficial transitional cell carcinoma. J Clin Oncol. 2003;21(23):4270–4276.
- Colombo R, Salonia A, Leib Z, et al. Long-term outcomes of a randomized controlled trial comparing thermochemotherapy with mitomycin-C alone as adjuvant treatment for non-muscle-invasive bladder cancer (NMIBC). BJU Int. 2011;107(6):912–918.
- Franckena M, Fatehi D, de Bruijne M, et al. Hyperthermia dose-effect relationship in 420 patients with cervical cancer treated with combined radiotherapy and hyperthermia. Eur J Cancer. 2009;45(11):1969–1978.
- Issels RD, Lindner LH, Verweij J, et al. Effect of neoadjuvant chemotherapy Plus regional hyperthermia on long-term outcomes Among patients With localized High-Risk soft tissue sarcoma: the EORTC 62961-ESHO 95 randomized clinical trial. JAMA Oncol. 2018;4(4):483–492.
- Maluta S, Schaffer M, Pioli F, et al. Regional hyperthermia combined with chemoradiotherapy in primary or recurrent locally advanced pancreatic cancer: an open-label comparative cohort trial. Strahlenther Onkol. 2011;187(10):619–625.
- Schroeder C, Gani C, Lamprecht U, et al. Pathological complete response and sphincter-sparing surgery after neoadjuvant radiochemotherapy with regional hyperthermia for locally advanced rectal cancer compared with radiochemotherapy alone. Int J Hyperthermia. 2012;28(8):707–714.
- van der Zee J, González D, van Rhoon GC, et al. Comparison of radiotherapy alone with radiotherapy plus hyperthermia in locally advanced pelvic tumours: a prospective, randomised, multicentre trial. Lancet. 2000;355(9210):1119–1125.
- Wust P, Rau B, Gellerman J, et al. Radiochemotherapy and hyperthermia in the treatment of rectal cancer. Recent Results Cancer Res. 1998;146:175–191.
- Kok HP, Cressman ENK, Ceelen W, et al. Heating technology for malignant tumors: a review. Int J Hyperthermia. 2020;37(1):711–741.
- Bruggmoser G, Bauchowitz S, Canters R, et al. Quality assurance for clinical studies in regional deep hyperthermia. Strahlenther Onkol. 2011;187(10):605–610.
- Schneider CJ, van Dijk JDP, de Leeuw AAC, et al. Quality assurance in various radiative hyperthermia systems applying a phantom with LED matrix. Int J Hyperthermia. 1994;10(5):733–747.
- van Dijk JDP, Schneider C, van Os R, et al. Results of deep body hyperthermia with large waveguide radiators. In: Bicher HI, McLaren JR , Pigliucci GM, editors. Consensus on hyperthermia for the 1990s. (Advances in Experimental Medicine and Biology). Vol. 267. Boston (MA): Springer; 1990. p. 315–319.
- Crezee J, Van Haaren PMA, Westendorp H, et al. Improving locoregional hyperthermia delivery using the 3-D controlled AMC-8 phased array hyperthermia system: a preclinical study. Int J Hyperthermia. 2009;25(7):581–592.
- Zweije R, Kok HP, Bakker A, et al. Technical and clinical evaluation of the Alba-4D 70MHz loco-regional hyperthermia system. European Microwave Conference; 2018 Sep 25–27; Madrid, Spain. New York, USA: Institute of Electrical and Electronics Engineers; 2018. p. 328–331.
- Bruggmoser G, Bauchowitz S, Canters R, et al. Guideline for the clinical application, documentation and analysis of clinical studies for regional deep hyperthermia: quality management in regional deep hyperthermia. Strahlenther Onkol. 2012;188(2):198–211.
- de Leeuw AA, Crezee J, Lagendijk JJ. Temperature and SAR measurements in deep-body hyperthermia with thermocouple thermometry. Int J Hyperthermia. 1993;9(5):685–697.
- Kok HP, Kotte ANTJ, Crezee J. Planning, optimisation and evaluation of hyperthermia treatments. Int J Hyperthermia. 2017;33(6):593–607.
- Kok HP, van der Zee J, Guirado FN, et al. Treatment planning facilitates clinical decision making for hyperthermia treatments. Int J Hyperthermia. 2021;38(1):532–551.
- Crezee J, Zweije R, Kok HP. Loco-regional hyperthermia delivery: patient-specific procedures for treatment optimisation. European Conference on Antennas and Propagation (EuCAP); 2020 Mar 15–20; Copenhagen, Denmark. Piscataway (NJ): IEEE; 2020.
- Kok HP, Korshuize-van Straten L, Bakker A, et al. Online adaptive hyperthermia treatment planning during locoregional heating to suppress treatment-limiting hot spots. Int J Radiat Oncol Biol Phys. 2017;99(4):1039–1047.
- Wiersma J, van Dijk JD, Sijbrands J, et al. The measurement of fringing fields in a radio-frequency hyperthermia array with emphasis on bolus size. Int J Hyperthermia. 1998;14(6):535–551.
- Crezee J, et al. Hyperthermia of deep seated pelvic tumors with a phased array of eight versus four 70 MHz waveguides. 47th European Microwave Conference Nurenberg; 2017 Oct 10–12; Nuremberg, Germany. New York, USA: Institute of Electrical and Electronics Engineers; 2017. p. 876–879.
- Kok HP, Beck M, Löke DR, et al. Locoregional peritoneal hyperthermia to enhance the effectiveness of chemotherapy in patients with peritoneal carcinomatosis: a simulation study comparing different locoregional heating systems. Int J Hyperthermia. 2020;37(1):76–88.