Abstract
Objective
To compare the clinical efficacy of percutaneous vertebroplasty (PVP) alone and microwave ablation (MWA) combined with PVP for the treatment of painful spinal metastases from non-small cell lung cancer (NSCLC).
Methods
From October 2014 to October 2021, the data of 58 NSCLC patients with refractory painful spinal metastases (visual analog scale score ≥ 5) were retrospectively collected and analyzed. Patients in Group A (n = 30) and Group B (n = 28) received PVP alone and MWA combined with PVP, respectively. The primary endpoint was pain relief. The secondary endpoints were quality of life (QoL), local tumor progression (LTP), and complications.
Results
The technical success rate was 100% in both groups. Patients in both groups showed similar pain relief at 1–12 weeks, but patients in Group B still showed sustained pain relief at 24 weeks compared to those in Group A (p = 0.03). The assessment of QoL showed similar changes. LTP (33.00% vs. 7.14%, p = 0.02) and cement leakage rates (40.00% vs. 7.14%, p = 0.03) were lower in Group B. The multivariate analysis demonstrated spinal metastases with a maximum diameter ≤ 3.0 cm (p = 0.027) and MWA combined with PVP (p = 0.028) were two independent protective factors for LTP. For cement leakage, spinal metastases with vertebral body compression (p = 0.019) was an independent risk factor, while MWA combined with PVP (p = 0.042) was an independent protective factor.
Conclusion
MWA combined with PVP for painful spinal metastases from NSCLC performed more sustained pain relief (>6 months) and ultimately improved QoL with lower LTP and cement leakage rates, compared to PVP alone.
Introduction
More than 60% of cases suffer from bone metastasis during the development of non-small cell lung cancer (NSCLC), and 15% of cases even seek medical advice with bone metastasis as the first symptom [Citation1,Citation2]. Of all sites of bone metastasis, the spinal metastases are the most severe due to the adjacent vital anatomical structures of the spine and the weight-bearing function of the body. The progression of spinal metastases can lead to skeletal-related events such as pathological fractures, spinal cord or nerve root compression [Citation3]. Even worse, the severe pain with or without mechanical instability caused by these complications, can impair functional status and diminished quality of life (QoL) [Citation4].
Currently, the treatment of spinal metastases is evolving toward a multidisciplinary field, and the most appropriate treatment should be selected based on the characteristics of the metastatic tumor and the general condition of the patient [Citation4,Citation5]. Although extracorporeal radiotherapy (ER) has been used as a standard pain relief tool with pain relief rates as high as 50–80% [Citation6], it is limited by relatively insensitive tumor tissue such as NSCLC, and the potential risk for pathologic fracture (11.9%) [Citation7]. A systematic literature review [Citation8] reported that 50% of patients who initially responded to ER relapsed within one year, and re-irradiation was limited by cumulative spinal cord tolerance. Surgery can be selectively used for significant spinal deformity, unstable pathological fracture, or spinal cord compression; however, it is characterized by large trauma, long healing time, and complication rates up to 10–20% [Citation9,Citation10].
Percutaneous vertebroplasty (PVP), is a minimally invasive alternative to treating spinal metastases with the advantages of rapid analgesia, maintenance of spinal stability, and rapid postoperative recovery [Citation11,Citation12]. However, it has certain technical limitations: (1) relatively poor tumor control; (2) solid tumors have a certain impact on bone cement distribution; and (3) cement injection with high-pressure results in easy leakage or tumor metastasis [Citation13]. Interestingly, PVP does not interfere with the implementation of subsequent adjuvant therapy and has been demonstrated to achieve satisfactory pain management and local tumor control in combination with other minimally invasive therapies [Citation14,Citation15]. Microwave ablation (MWA) can make the dipole in the tissue rotate in the microwave field and continuously accelerate the collision to generate heat energy, which rapidly causes coagulative necrosis of tumor tissue and is less affected by the carbonization effect [Citation16]. Therefore, this retrospective case-control study aimed to evaluate the efficacy and safety of MWA combined with PVP and seek relevant influencing factors associated with local tumor progression (LTP) and cement leakage in patients with spinal metastases.
Methods
Study subjects
From our center’s database, clinical data of 129 NSCLC patients with refractory painful spinal metastases who underwent PVP combined with or without MWA from October 2014 to October 2021 were extracted and reviewed. In total, 58 patients were ultimately enrolled in this retrospective study, of which 30 patients received PVP alone (Group A) and 28 patients received MWA combined with PVP (Group B); none of the baseline clinicopathological characteristics were significantly different (). The inclusion criteria were as follows: (1) 18–75 years old; (2) primary lesion confirmed as NSCLC; (3) single spinal metastase confirmed by magnetic resonance (MR) or computed tomography; (4) visual analog scale (VAS) score ≥ 5; (5) the presence of persistent or recurrent pain after ER or standard analgesics; (6) local tumor diameter ≤ 4 cm; and (7) Karnofsky score ≥ 60. The exclusion criteria were as follows: (1) severe damage to the posterior vertebral cortex; (2) severe coagulation dysfunction, such as prothrombin time ≥ 25 s and PLT count < 30 × 109/L; (3) uncontrollable infection at the puncture path; (4) severe cardiopulmonary dysfunction; (5) expected lifetime ≤ 3 months; and (6) incomplete data. The workflow diagram of this study was illustrated in . The Ethics Committee of The First Affiliated Hospital of Zhengzhou University approved the retrospective study (Ethics approval number: 2013-KY-095), and the requirement for individual consent for this retrospective analysis was waived.
Figure 1. the workflow Schematic diagram of this study. NSCLC, non-small cell lung cancer; VAS, visual analog scale; MWA, microwave ablation; PVP, percutaneous vertebroplasty; QoL, quality of life; LTP, local tumor progression.
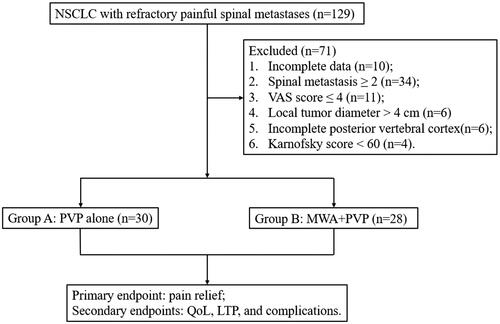
Table 1. Baseline clinicopathological characteristics of patients in both groups.
Preparation before the procedure
Routine blood tests, liver/kidney function tests, coagulation function tests, tumor markers, electrocardiograms, and enhanced CT scans were completed before all procedures. Multidisciplinary team of specialists involving neurosurgery, orthopedics, oncology, radiology, and radiation therapy evaluated all patients prior to the procedures. Since the introduction of the MWA device at our center in March 2018, MWA combined with PVP has begun to be recommended and used for the treatment of NSCLC patients with painful spinal metastases, whereas PVP alone was previously used primarily for the treatment of painful spinal metastases from NSCLC. Two interventional radiologists (DC Jiao and XW Han with 15 and 20 years of experience, respectively) performed all the procedures.
The procedure of groups A and B
The patient lay prone on a digital subtraction angiography (DSA) machine (Artis zeego with iGuide virtual navigation puncture system and C-arm CT (Siemens, Munich, Germany), and dexmedetomidine (0.5 mg/kg) and diloxin (10 mg) were intravenously infused by pump to obtain a satisfactory pain control state. All cases first underwent C-arm CT according to previous parameters [Citation17], and iGuide navigation (Syngo X Workplace) was used to plan the puncture path. All puncture paths were selected depending on the convenience of the puncture and the operator’s experience. After local sterilization, local anesthesia was performed with 2% lidocaine (10 ml), and a 13 G puncture needle (12 cm in length, Dragon Crown Medical Products Co., Ltd. China) was advanced under real-time fluoroscopy; the correct position was confirmed by the anterior and lateral positions. A contrast agent (320 mg I/100 ml, Hengrui Medical Technology Co., Ltd.) (5 ml) was used to visualize the peripheral vertebral veins. Bone cement (polymethyl methacrylate, PMMA, Heraeus Medical GmbH, Wertheim, Germany) was injected under the real-time fluoroscopy monitor to complete PVP in Group A.
For Group B, before PVP, the 16 G MWA applicator (active tip and total length 1.5 cm and 20 cm, respectively; Medical Instrument Co., Ltd. Nanjing, China) was inserted into the vertebral metastases by coaxial technology (Figure S1). After its location was confirmed by fluoroscopy or C-arm CT, the MWA applicator was connected to the MWA generator system (power range 0–100 W, voltage: 220 V, frequency 2.45 GHz, ECO-100E, Nanjing, China). The ablation power was selected as 25–45 W according to the manufacturer’s recommendation, the patient’s pain tolerance, and the operator’s experience. The cement was injected into the MWA zone after applicator withdrawal as in Group A. The 13 G bone puncture needle was drawn out when the cement filling was satisfactory, or cement leakage was confirmed by fluoroscopy or C-arm CT (). The patient was sent back to the observation room after local bandaging and intravenous anesthesia were stopped.
Figure 2. A 61-year-old Female patient with thoracic vertebra 10 (T10) metastases from NSCLC, and VAS score was 7 at pre-procedure (A-C). (A) the 13 G puncture needle was inserted into the T10 under the guidance of C-arm computed tomography (CT) and fluoroscopy, and intra-operative C-arm CT showed that metastases damaged the integrity of the T10 (arrow); (B) bone cement was slowly injected under real-time fluoroscopy; (C) intra-operative C-arm CT showed adequate filling of the bone cement in the T10, and the VAS score decreased to 2 at 1 week evaluation. Another 57-year-old male patient with lumbar vertebra 4 (L4) metastases from NSCLC, and VAS score was 8 at pre-procedure (D-F). (D) the 13G puncture needle was inserted into the L4 under the guidance of C-arm CT and fluoroscopy, intra-operative C-arm CT showed that metastases damaged the integrity of the L10 (arrow), and a MWA applicator was inserted into the L4 by coaxial technology; (E) the location of puncture needle and applicator were confirmed by real-time fluoroscopy; (F) intra-operative C-arm CT showed adequate filling of the bone cement in the L4, and the VAS score was reduced to 2 at 1 week evaluation.
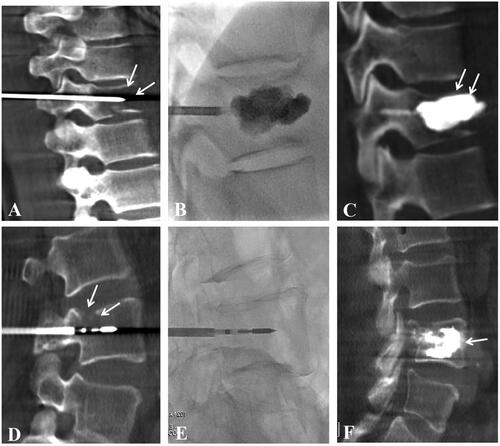
Definition and follow-up
Technical success was defined as PVP and MWA completion and bone cement filling at least 1/2 vertebral osteolytic area. The primary endpoint was pain relief, which was evaluated by the daily morphine consumption (DMC) according to the conversion standard of the NCCN guidelines for adult cancer pain management in 2019 [Citation18] and VAS (0–3 points, mild pain; 4–6 points, moderate pain; 7–10 points, severe pain).
The secondary endpoints included QoL, LTP, and complications. QoL was comprehensively assessed Oswestry Disability Index (ODI) and QoL scores about five aspects (Karnofsky score, appetite, sleep, spiritual, and fatigue). The Karnofsky score was on a scale of 10 (range: 10–100). The following 4 parameters were scored using a five-point scale (1 = worst, 2 = bad, 3 = mild, 4 = normal, and 5 = very good). LTP was defined as a follow-up CT/MR showing the progression of disease at the treatment site according to the modified Response Evaluation Criteria in Solid Tumors (mRECIST) [Citation19]. Complications were evaluated by the Cardiovascular and Interventional Radiological Society of Europe (CIRSE) classification [Citation20]. The dose area product (DAP) was collected in the DSA system, including fluoroscopy and C-arm CT scans. The effective dose = κDAP × DAP, κDAP = 0.17 mSv/Gy·cm2 according to a previous study [Citation17].
The clinical assessments were conducted at T0 (pretreatment), T1 (1 week), T4 (4 weeks), T12 (12 weeks), and T24 (24 weeks) for each patient in both groups. At each examination, DMC, VAS score, ODI, and QoL score were self-reported and recorded. Dynamic enhanced CT/MR was performed at 1 month after procedures and every 3 months thereafter. The last follow-up was performed in September 2022.
Statistical analysis
All statistical analyses were performed using the SPSS 24.0 software package (IBM Corp, New York, USA). Descriptive values are presented as the medians, numbers, means ± standard deviation, and ranges. Pearson’s chi-squared test was used to compare the categorical variables. Independent samples t tests were used to compare continuous variables, and paired t tests were used to compare continuous variables at different time points within groups. The Wilcoxon test was used to compare the VAS scores and QoL scores. Univariate logistic regression model analysis using the enter method was performed to identify influencing factors associated with LTP or cement leakage. Factors with differences < 0.1 were included in the multivariate logistic regression model, and independent predictors were further identified. The p < 0.05 indicated significant difference.
Results
General characteristics
The intraoperative and postoperative parameters are listed in . Both groups of patients successfully completed the procedures with the technical success rates of 100%. Although the total procedure time was longer in Group B than in Group A (p = 0.01), there was no significant difference in fluoroscopy dose (p = 0.14), which may be related to the fact that there was no significant difference in the number of intraoperative C-arm CT scans (p = 0.42).
Table 2. Intraoperative and postoperative parameters from different therapy strategies.
Pain relief
The DMC and VAS scores at T1, T4, T12, and T24 were significantly lower than those at T0 in both groups, and the differences were statistically significant (all p < 0.01, ). However, pain relief was comparable between the two groups at T1-T12 and showed no significant differences (). Interestingly, at T24, patients in Group B had lower DMC and VAS scores than those in Group A (p = 0.03 and p = 0.01), demonstrating superior mid-term pain relief. According to the stratified analysis of VAS grades (), the pain in both groups was significantly relieved at T1, as ‘mild pain’, and was maintained until T12. However, at T24, the pain worsened again in three patients in Group A and presented as ‘moderate pain’, while all patients in Group B remained in ‘mild pain’.
Figure 3. Changes of the indicators in both groups at different times. (A) trends in visual analog scale (VAS) scores over time in both groups; (B) trends in daily morphine consumption (DMC) over time in both groups; (C) stratification analysis of VAS grades between two groups at different times; (D) trends in Oswestry Disability Index (ODI) over time in both groups; (E) trends in Karnofsky score over time in both groups; (F) at 24 weeks (T24), comparison of sleep, appetite, fatigue, and spiritual scores between two groups.
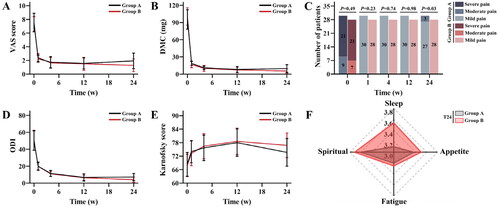
Table 3. Pain relief and ODI evaluation at both groups.
QoL
For ODI and QoL scores about five aspects, patients in both groups showed significant functional status improvement from T1-T12 after treatment compared to T0 in each group (), but there were no significant differences between the two groups at each time point (all p > 0.05). As shown in Table S1, patients in both groups had significantly higher QoL scores at different time points after procedures than before procedures. At T24, ODI showed a rebound in Group A, which was significantly different compared to that in Group B (p = 0.02). Moreover, the sleep and Karnofsky scores of patients in Group A were lower than those in Group B at T24, with statistically significant differences (p = 0.29 and 0.21, respectively) ().
LTP and complications
During the follow-up period, 10 patients (33.33%) in Group A experienced local progression after treatment, while only 2 patients (7.14%) in Group B experienced LTP, with a significant difference (p = 0.02, ). Multivariate logistic regression model analysis (Table S2) revealed that spinal metastases with maximum diameter ≤ 3.0 cm (OR: 0.066, p = 0.027) and combined treatment with MWA and PVP (OR: 0.127, p = 0.028) were two independent protective factors against LTP, restricting the occurrence of LTP. The total incidence of complications was significantly lower in Group B patients than in Group A (53.33% vs. 14.29%, p = 0.02), and the incidence of cement leakage was significantly lower in Group B than in Group A (40.00% vs. 7.14%, p = 0.03). Moreover, multivariate analysis () showed that spinal metastases with vertebral body compression (OR: 21.553, p = 0.019) was an independent risk factor that contributed to the occurrence of cement leakage, while combined treatment with MWA and PVP (OR: 0.131, p = 0.042) was an independent protective factor.
Table 4. Univariable and multivariable analyses of predictors of cement leakage.
Discussion
For spinal metastases from NSCLC, PVP alone is a safe and effective treatment for patients with painful spinal metastases, but its local tumor control effect is limited [Citation5,Citation21,Citation22]. Combination with other minimally invasive treatments for long-term local control of metastatic lesions is worth being investigated and applied. Thermal ablation, especially MWA, performs high heat generation efficiency, strong local damage, low cost, and repeatability, which can theoretically make up for the shortages of PVP alone. To the best of our knowledge, this is the first case-control study to assess the efficacy of MWA combined with PVP and PVP alone for the treatment of painful spinal metastases.
In a prospective study by Chew et al. [Citation23], 128 patients with spinal malignancies (41 myeloma and 87 spinal metastases) were treated with PVP alone, and VAS scores decreased from 7.57 ± 1.88 preoperatively to 4.77 ± 2.69 postoperatively (p < 0.001). Kaloostian et al. [Citation22] concluded that PVP for painful spinal disorders achieved 91% (range 73%-100%) pain relief and 62% (range 52%-70%) functional improvement postoperatively in a meta-analysis. In this study, DMC and VAS scores in both groups were significantly lower at T1-T12 compared to at T0 (all p < 0.05), with no statistical difference between the two groups (p > 0.05), which is consistent with previous studies [Citation21–23]. However, further follow-up revealed that the pain relief with PVP alone for spinal metastases did not appear to be sufficient. Three patients with spinal metastases treated with PVP alone experienced a recurrence of pain at T24, presenting as ‘moderate pain’, while all patients treated with MWA combined with PVP showed sustained pain relief at least until T24. Moreover, there was a significant difference in DMC and VAS scores of patients compared between the two groups at T24, which was similar to the findings of Lv et al. [Citation24]. This may be related to the following factors: (1) MWA disrupts pain nerve fibers in the periosteum and cortex, reducing pain transmission; (2) MWA inactivates tumor tissue, decreases osteoclast activity of tumor cells, and reduces the release of neurostimulator cytokines (such as interleukins and tumor necrosis factor-α); and (3) PVP after MWA enhances spinal stability and reduces the occurrence of pathological fractures, which in turn reduces the compression of the spinal cord or nerve roots caused by them. Deib et al. [Citation25] advocated that the bone-tumor interface should be targeted for maximum pain relief, where the inflammatory microenvironment stimulates the nerve endings the most.
The distinguishing feature of this study is QoL evaluation, and both groups showed no differences in sleep, appetite, fatigue, spiritual, or Karnofsky scores at T1-T12. This may be associated with pain relief and enhanced spinal stability, allowing the patients to recover their psychological and functional status. However, at T24, patients treated with PVP alone had lower sleep and Karnofsky score of QoL scores and ODI than those treated with MWA combined with PVP, with significant differences (p < 0.05). This may be due to the deterioration of pain at T24 in some patients treated with PVP alone, allowing functional status and sleep quality to be compromised again. Therefore, MWA combined with PVP performs excellent efficacy in improving the QoL of patients with spinal metastases.
The thermal effect of bone cement in a low heat transfer mode is inadequate, resulting in limited local tumor control in spinal metastases treated with PVP alone [Citation5]. Moreover, as the local recurrence of the tumor occurs, the invasion of tumor cells into the vertebral body or compression of nerves can again cause pain and spinal instability. MWA was less affected by high-impedance tissues such as bone and has deep tumor penetration, which can rapidly inactivate tumor tissues and occlude tumor blood vessels, thus contributing to local tumor control [Citation4,Citation25,Citation26]. Khan et al. [Citation27] reported 69 patients with spinal metastases treated with MWA combined with cementoplasty, and follow-up imaging at 20–24 weeks in 59 of 61 surviving patients showed no LTP. A case-control study of radiofrequency ablation combined with cementoplasty versus cementoplasty alone for spinal metastases [Citation24] showed LTP of 11.4% and 30.8% (p < 0.05) in both groups, respectively. In this study, the incidence of LTP at T24 was lower in Group B than that in Group A (7.14% vs. 33.33%, p = 0.02), which demonstrated the superiority of MWA combined with PVP for local tumor control. Further analysis of independent risk factors for LTP in spinal metastases confirmed that MWA with PVP reduced the incidence of LTP compared to PVP alone, which was consistent with previous studies [Citation4,Citation25–27]. In addition, a maximum diameter of spinal metastases ≤ 3.0 cm was another independent protective factor for LTP. This may be attributed to the fact that larger-sized tumors tend to cause increased surrounding microinvasive areas, which makes it difficult to ensure adequate ablation margins [Citation25,Citation27]. For the larger spinal metastases from NSCLC, the experience in our center is that MWA combined with PVP therapy is preferred than PVP alone and multiple overlapping ablations for the metastases and an additional 3 mm ablation margin for microtumor spread are performed. Moreover, C-arm CT facilitates real-time detection of intraoperative tissue changes, which is considered as the best tool for MWA combined with PVP [Citation28].
Due to the space-occupying effect of irregular metastases limiting cement distribution and high-pressure injections, PVP was highly susceptible to cement leakage, with a high incidence of 30–70% in the previous studies [Citation28–33]. During the MWA combined with PVP for spinal metastases, the remaining bone shell after MWA ablation can provide sufficient space for filling cement, and MWA also thrombosed the para- and intravertebral venous plexus, thus reducing the risk of cement leakage. In this study, compared to those in PVP alone, cement volume was higher in MWA combined with PVP (3.87 vs. 3.47 ml, p = 0.01); but cement leakage rate was lower in MWA combined with PVP (7.14% vs. 40.00%, p = 0.03). Multivariate logistic regression analysis similarly concluded that MWA combined with PVP was an independent protective factor against the occurrence of bone cement leakage and could reduce its incidence. Vertebral body compression is an independent risk factor for the development of cement leakage, which is consistent with the results of previous studies [Citation4,Citation5,Citation31]. This may be attributed to the microscopic intravertebral fractures or cortical continuity impetration following compressive changes in the vertebral body due to tumor destruction, which would lead to more frequent cement leakage. The strategy at our center is to use high-viscosity cement and to inject it in small amounts and multiple times under the dual guidance of real-time fluoroscopy and C-arm CT; unused bone cement should be placed in ice saline to prolong the solidification time.
In addition, nerve damage was the most serious complication, which did not occur in this study. Other centers use intraoperative temperature monitoring to reduce injury. Although this method is safer, it also increases the complexity of the procedures [Citation34]. The experience of our center is summarized as follows: (1) Local anesthesia is recommended, and the operator and patient can communicate with each other. If there is a burning sensation or numbness in the lower limbs, MWA should be stopped in time, and the temperature should be lowered. (2) The puncture cannula should be placed in the front 1/3 of the vertebral body. (3) The selection of power is very critical, and a low power/short time mode is recommended for spinal metastases to minimize nerve damage to the patient.
However, several limitations remained in this study: (1) The nature of this study was retrospective, and the sample size was small. Future prospective, multicenter trials could yield a higher level of evidence. (2) Considering that the pain of patients may be affected by the metastatic lesions or additional systemic therapy, there may be a potential bias in this study. (3) The inclusion factors in the univariate and multivariate logistic regression were few, and other potential independent risk factors were worthy of further deeper investigation.
Conclusions
In conclusion, MWA combined with PVP is safe and effective for the treatment of painful spinal metastases from NSCLC, which can provide more sustained pain relief (> 6 months) and ultimately improve the QoL of patients, compared to PVP alone. Moreover, the application of MWA prior to PVP may improve the local anti-neoplastic effect and reduce the cement leakage rate.
Ethics approval and consent to participate
This retrospective study was performed in accordance with the Declaration of Helsinki and was approved by the Clinical Ethics Committees of the First Affiliated Hospital of Zhengzhou University (Ethical review number: 2013-KY-095). The requirement for informed consent was waived by the Ethics Committee of The First Affiliated Hospital of Zhengzhou University because of the retrospective nature of the study.
Author contributions
Conception and design: Liu YM; Administrative support: Jiao DC and Han XW; Provision of study materials or patients: Jiao DC and Han XW; Collection and assembly of data: Liu YM and Zhang CZ; Data analysis and interpretation: Liu YM, Yuan HY, and Milan S; Manuscript writing: Liu YM; preparing: Liu YM and Milan S; Figure 4–5 preparing: Liu YM and Zhang CZ; All tables preparing: Liu YM; Final approval of manuscript: all authors.
Supplemental Material
Download PDF (255.5 KB)Disclosure statement
No potential conflict of interest was reported by the author(s).
Data availability statement
In addition to the raw data in the manuscript, the datasets used are available from the corresponding author on reasonable request.
Additional information
Funding
Reference
- Herbst RS, Morgensztern D, Boshoff C. The biology and management of non-small cell lung cancer. Nature. 2018;553(7689):446–454. doi: 10.1038/nature25183.
- Broderick SR. Adjuvant and neoadjuvant immunotherapy in non-small cell lung cancer. Thorac Surg Clin. 2020;30(2):215–220. doi: 10.1016/j.thorsurg.2020.01.001.
- Del Conte A, De Carlo E, Bertoli E, et al. Bone metastasis and immune checkpoint inhibitors in Non-Small cell lung cancer (NSCLC): microenvironment and possible clinical implications. Int J Mol Sci. 2022;23(12):6832. doi: 10.3390/ijms23126832.
- Tomasian A, Gangi A, Wallace AN, et al. Percutaneous thermal ablation of spinal metastases: recent advances and review. AJR Am J Roentgenol. 2018;210(1):142–152. doi: 10.2214/AJR.17.18205.
- Zhang HR, Xu MY, Yang XG, et al. Percutaneous vertebral augmentation procedures in the management of spinal metastases. Cancer Lett. 2020;475:136–142. doi: 10.1016/j.canlet.2020.01.038.
- Needham PR, Mithal NP, Hoskin PJ. Radiotherapy for bone pain. J R Soc Med. 1994;87(9):503–505. doi: 10.1177/014107689408700904.
- Ito K, Ogawa H, Shimizuguchi T, et al. Stereotactic body radiotherapy for spinal metastases: clinical experience in 134 cases from a single japanese institution. Technol Cancer Res Treat. 2018;17:1533033818806472. doi: 10.1177/1533033818806472.
- Kotecha R, Dea N, Detsky JS, et al. Management of recurrent or progressive spinal metastases: reirradiation techniques and surgical principles. Neurooncol Pract. 2020;7(Suppl 1):i45–i53. doi: 10.1093/nop/npaa045.
- Galgano M, Fridley J, Oyelese A, et al. Surgical management of spinal metastases. Expert Rev Anticancer Ther. 2018;18(5):463–472. doi: 10.1080/14737140.2018.1453359.
- de Ruiter GC, Nogarede CO, Wolfs JF, et al. Quality of life after different surgical procedures for the treatment of spinal metastases: results of a single-center prospective case series. Neurosurg Focus. 2017;42(1):E17. doi: 10.3171/2016.6.FOCUS16150.
- Bao L, Jia P, Li J, et al. Percutaneous vertebroplasty relieves pain in cervical spine metastases. Pain Res Manag. 2017;2017:3926318. doi: 10.1155/2017/3926318.
- Huang M, Zhu H, Liu T, et al. Comparison of external radiotherapy and percutaneous vertebroplasty for spinal metastasis. Asia Pac J Clin Oncol. 2016;12(2):e201–e208. doi: 10.1111/ajco.12162.
- Mohme M, Riethdorf S, Dreimann M, et al. Circulating tumour cell release after cement augmentation of vertebral metastases. Sci Rep. 2017;7(1):7196. doi: 10.1038/s41598-017-07649-z.
- Yang Z, Tan J, Zhao R, et al. Clinical investigations on the spinal osteoblastic metastasis treated by combination of percutaneous vertebroplasty and (125)I seeds implantation versus radiotherapy. Cancer Biother Radiopharm. 2013;28(1):58–64. doi: 10.1089/cbr.2012.1204.
- Masala S, Roselli M, Massari F, et al. Radiofrequency heat ablation and vertebroplasty in the treatment of neoplastic vertebral body fractures. Anticancer Res. 2004;24(5B):3129–3133.
- Cazzato RL, de Rubeis G, de Marini P, et al. Percutaneous microwave ablation of bone tumors: a systematic review. Eur Radiol. 2021;31(5):3530–3541. doi: 10.1007/s00330-020-07382-8.
- Jiao DC, Li TF, Han XW, et al. Clinical applications of the C-arm cone-beam CT-based 3D needle guidance system in performing percutaneous transthoracic needle biopsy of pulmonary lesions. Diagn Interv Radiol. 2014;20(6):470–474. doi: 10.5152/dir.2014.13463.
- Swarm RA, Paice JA, Anghelescu DL, BCPS., et al. Adult cancer pain, version 3.2019, NCCN clinical practice guidelines in oncology. J Natl Compr Canc Netw. 2019;17(8):977–1007. doi: 10.6004/jnccn.2019.0038.
- Seyal AR, Gonzalez-Guindalini FD, Arslanoglu A, et al. Reproducibility of mRECIST in assessing response to transarterial radioembolization therapy in hepatocellular carcinoma. Hepatology. 2015;62(4):1111–1121. doi: 10.1002/hep.27915.
- Filippiadis DK, Binkert C, Pellerin O, et al. Cirse quality assurance document and standards for classification of complications: the cirse classification system. Cardiovasc Intervent Radiol. 2017;40(8):1141–1146. doi: 10.1007/s00270-017-1703-4.
- Health Quality Ontario. Vertebral augmentation involving vertebroplasty or kyphoplasty for Cancer-Related vertebral compression fractures: a systematic review. Ont Health Technol Assess Ser. 2016;16(11):1–202.
- Kaloostian PE, Yurter A, Zadnik PL, et al. Current paradigms for metastatic spinal disease: an evidence-based review. Ann Surg Oncol. 2014;21(1):248–262. doi: 10.1245/s10434-013-3324-8.
- Chew C, Ritchie M, O'Dwyer PJ, et al. A prospective study of percutaneous vertebroplasty in patients with myeloma and spinal metastases. Clin Radiol. 2011;66(12):1193–1196. doi: 10.1016/j.crad.2011.08.004.
- Lv N, Geng R, Ling F, et al. Clinical efficacy and safety of bone cement combined with radiofrequency ablation in the treatment of spinal metastases. BMC Neurol. 2020;20(1):418. doi: 10.1186/s12883-020-01998-5.
- Deib G, Deldar B, Hui F, et al. Percutaneous microwave ablation and cementoplasty: clinical utility in the treatment of painful extraspinal osseous metastatic disease and myeloma. AJR Am J Roentgenol. 2019;212(6):1377–1384. doi: 10.2214/AJR.18.20386.
- Kastler A, Alnassan H, Aubry S, et al. Microwave thermal ablation of spinal metastatic bone tumors. J Vasc Interv Radiol. 2014;25(9):1470–1475. doi: 10.1016/j.jvir.2014.06.007.
- Khan MA, Deib G, Deldar B, et al. Efficacy and safety of percutaneous microwave ablation and cementoplasty in the treatment of painful spinal metastases and myeloma. AJNR Am J Neuroradiol. 2018;39(7):1376–1383. doi: 10.3174/ajnr.A5680.
- Pusceddu C, Sotgia B, Fele RM, et al. Treatment of bone metastases with microwave thermal ablation. J Vasc Interv Radiol. 2013;24(2):229–233. doi: 10.1016/j.jvir.2012.10.009.
- Xiao Q, Zhao Y, Qu Z, et al. Association between bone cement augmentation and new vertebral fractures in patients with osteoporotic vertebral compression fractures: a systematic review and Meta-Analysis. World Neurosurg. 2021;153:98–108.e3. doi: 10.1016/j.wneu.2021.06.023.
- Tang B, Cui L, Chen X, et al. Risk factors for cement leakage in percutaneous vertebroplasty for osteoporotic vertebral compression fractures: an analysis of 1456 vertebrae augmented by Low-Viscosity bone cement. Spine (Phila Pa 1976). 2021;46(4):216–222. doi: 10.1097/BRS.0000000000003773.
- Wu L, Fan J, Yuan Q, et al. Computed tomography-guided microwave ablation combined with percutaneous vertebroplasty for treatment of painful high thoracic vertebral metastases. Int J Hyperthermia. 2021;38(1):1069–1076. doi: 10.1080/02656736.2021.1951364.
- Qiu YY, Zhang KX, Ye X, et al. Combination of microwave ablation and percutaneous osteoplasty for treatment of painful extraspinal bone metastasis. J Vasc Interv Radiol. 2019;30(12):1934–1940. doi: 10.1016/j.jvir.2019.08.008.
- Zhao W, Wang H, Hu JH, et al. Palliative pain relief and safety of percutaneous radiofrequency ablation combined with cement injection for bone metastasis. Jpn J Clin Oncol. 2018;48(8):753–759. doi: 10.1093/jjco/hyy090.
- Lecigne R, Cazzato RL, Dalili D, et al. Transosseous temperature monitoring of the anterior epidural space during thermal ablation in the thoracic spine. Cardiovasc Intervent Radiol. 2021;44(6):982–987. doi: 10.1007/s00270-021-02771-y.