Abstract
Background
Microwave ablation (MWA) is a widely adopted treatment technique for hepatocellular carcinoma (HCC). However, MWA alone is of limited use and has a high recurrence rate. Transforming growth factor-β1 (TGF-β1) is recognized as a potential therapeutic target for HCC patients. Therefore, this study was designed to investigate whether the TGF-β1 inhibitor could increase the efficacy of MWA therapy for HCC treatment.
Methods
In vitro, HCC cells challenged with TGF-β1 inhibitor (SB-525334), or normal saline were then heated by microwave. Methyl tetrazolium assays were performed to detect cell survival rate and half-maximal drug inhibitory concentration (IC50). Cell viability and apoptosis were detected by cell counting kit-8 assays, flow cytometry and western blotting. In vivo, the mice injected with HepG2 cells received oral gavage of SB-525334 (20 mg/kg) or normal saline and MWA at a power of 15 W. Tumor volume was recorded. Expression of Ki67 and apoptosis-related proteins were detected by immunohistochemistry and western blotting. TUNEL assays were used to detect cell death ratio. Histopathological changes were examined by hematoxylin and eosin staining. The mechanisms associated with the function of MWA combined with TGF-β1 inhibitor in HCC development were explored by western blotting.
Results
Combination of MWA and SB-525334 decreased the survival rate and promoted the apoptosis of HCC cells compared with MWA alone. SB-525334 enhanced the suppressive effect of MWA on tumor growth and amplified cell apoptosis. Mechanistically, MWA collaborated with SB-525334 inhibitor inactivated the TGF-β1/Smad2/Smad3 pathway.
Conclusion
TGF-β1 inhibitor enhances the therapeutic effect of MWA on HCC.
Introduction
Hepatocellular carcinoma (HCC), a hematology-rich malignant tumor that originates from liver cells, is the fifth most common cancer and second leading cause of cancer-related death worldwide [Citation1,Citation2]. Viral hepatitis B/C and cirrhosis, metabolic liver disease (particularly diabetes mellitus and nonalcoholic fatty liver disease), alcohol addiction and exposure to dietary toxins such as aflatoxins and aristolochic acid are critical risk factors for HCC [Citation3]. Currently, radiofrequency, microwave, laser and cryoablation are available as curative treatment options for early-stage HCC [Citation4]. Microwave ablation (MWA), a thermal ablation technique, is known for its theoretical strengths such as rapid heating, large ablation zone, high temperature, and low susceptibility to heat-sink effect and thus has become a promising technique in the thermal ablation field [Citation5]. For HCC tumors <3 cm, MWA has achieved favorable effects, and its ablation rate reaches 97.1%; however, the complete ablation rate for tumors (3–5 cm and 5–8 cm) is decreased to 82.6% and 64.3%, respectively [Citation6,Citation7]. Additionally, MWA with sufficient energy for HCC in risky locations (such as adjacent to the diaphragm, gallbladder, large vessels, hilum and gastrointestinal tract) may cause damage to important structures, while insufficient MWA may leave residual tumor [Citation8–11]. Moreover, following MWA treatment, the high recurrence and metastasis rats adversely affect the long-term prognosis [Citation12]. Liver transplantation is also a well-established indication for patients with early-stage HCC, but the shortage of available organ donors imposes the application of restrictive criteria to secure the optimal use of the available livers [Citation13]. Unfortunately, HCC is asymptomatic in the early stages, and more than 60% of patients with HCC are diagnosed at an advanced stage [Citation14]. Chemotherapy and radiotherapy are effective in controlling tumor growth at an advanced stage, but their efficacy is greatly limited by their low specificity [Citation15,Citation16]. Thus, findings novel therapeutic strategies for the efficient treatment of HCC remains an important medical issue.
The immune system plays a critical role in controlling cancer progression. Dysfunctional tumor-immune system interactions lead to immune evasion through impaired antigen recognition or by generating an immunosuppressive tumor microenvironment [Citation17]. Various immunomodulatory factors, such as tumor antigens, danger signals and cytokines, are released after MWA and play a critical role in stimulating the antitumor immune response [Citation5]. The immune response produced by ablation alone is often too modest to completely expunge established tumors [Citation18]. MWA combined with anti-programmed death-1 (PD-1)/anti-cytotoxic T-lymphocyte-associated protein 4 (CTLA-4) can protect mice from recurrence with improved survival [Citation19]. MWA combined with apatinib (vascular endothelial growth factor receptor-2, VEGFR-2) and camrelizumab (anti-PD-1 antibody) therapy in patients with advanced HCC results in durable antitumor response and significantly improves progression-free survival and overall survival [Citation20]. These preclinical and clinical studies have suggested that MWA can induce therapeutically effective systemic anti-tumor immune response if combined with appropriate immunomodulators.
The transforming growth factor-β (TGF-β) pathway is a multifunctional signaling cascade involved in the regulation of critical cellular processes, such as cell differentiation, apoptosis, proliferation, epithelial-to-mesenchymal transition (EMT) and extracellular matrix formation [Citation21]. Previous studies have revealed that TGF-β is a master immune checkpoint and acts at multiple steps to suppress the generation of anti-tumor immune responses [Citation22,Citation23]. TGF-β consists of three different mammalian isoforms, including TGF-β1, TGF-β2 and TGF-β3. TGF-β1 is the most abundantly expressed isoform in immune cells among three TGF-β isoforms [Citation24]. TGF-β1 is found to be upregulated in patients with HCC [Citation25,Citation26], and TGF-β1 can contribute to HCC progression and prognosis via regulating Tregs and γδ T cells to modulate tumor immunity in patients [Citation27]. Additionally, TGF-β1 is found to promote the proliferative, migrative and invasive capabilities of HCC cells in vitro and in vivo [Citation28–30], and its inhibition can inhibit HCC cell growth, migration and invasion in vitro and in vivo [Citation31]. Furthermore, TGF-β ligands bind to their receptors and then phosphorylate TGF-βR1 which activates the Smad2/Smad3-mediated pathway [Citation32]. Based on these findings, TGF-β1 could be a potential therapeutic target for HCC patients. However, whether TGF-β1 inhibitor improves the antitumor effect of MWA remains unknown. Therefore, this study was designed to detect the roles of the combination of MWA and TGF-β1 inhibitor in HCC. We hypothesized that MWA combined with TGF-β1 inhibitor can prevent HCC progression. This study would provide novel insights for the application of MWA combined with TGF-β1 inhibitor for HCC treatment.
Methods
Cell culture and treatment
Two human HCC cell lines, HepG2 and Huh7, were purchased from Procell (Wuhan, China) and incubated in Dulbecco’s Modified Eagle Medium (DMEM; Solarbio, Beijing, China) containing 10% fetal bovine serum (FBS) and 1% penicillin/streptomycin at 37 °C with 5% CO2.
When cells reached 90%–95% confluency, they were digested and prepared in suspension with a density of 1 × 104 cells. After HepG2 and Huh7 cells were adhered to the plate for 20 h, fresh culture medium with 13.9 nM TGF-β1 inhibitor (, SB-525334; MedChemExpress, Shanghai, China) or normal saline was added to HepG2 cells, and fresh culture medium with 15.6 nM TGF-β1 inhibitor or normal saline was added to Huh7 cells.
Figure 1. MWA combined with TGF-β1 inhibitor decreases survival rate of HCC cells. (A) Chemical structure of SB-525334. (B) Schematic illustration of MWA combined with TGF-β1 inhibitor (SB-525334). (C) Time temperature curve under 15 W MWA. (D) The IC50 value of SB-525334 was measured in HepG2 and Huh7 cells via MTT assays. (E) MTT assays to detect the role of MWA combined with SB-525334 in cell survival at different temperatures. Data were analyzed by one way analysis of variance followed by Tukey’s post hoc analysis and Student’s t test and expressed as mean ± SD of three independent experiments. *p < .05, **p < .01, ***p < .001 vs. NS (37 °C) group.
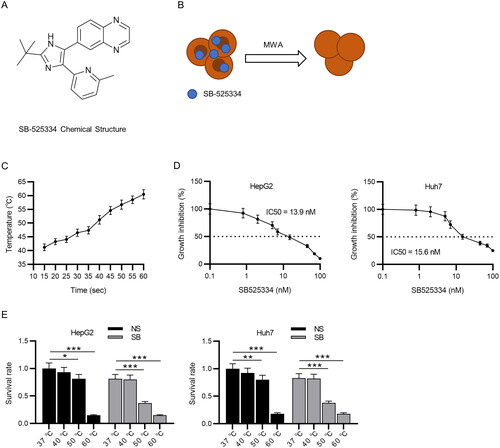
Methyl tetrazolium (MTT) assays
The HepG2 and Huh7 cells was prepared at 7 × 104 cells/mL, and then 500 μL suspension was added to each well of a 48-well plate and incubated for 20 h at 37 °C. Then, fresh culture medium with SB-525334 or normal saline was added and incubated for 4 h. Subsequently, the cells were heated by microwave to 37 °C, 40 °C, 50 °C and 60 °C, and after 4 h, the cells were incubated with 5 mg/mL MTT solution (Procell) in a 37 °C incubator for 4 h. The crystal violet was dissolved with DMSO solution. A microplate reader was used to measure the optical density at 490 nm. The cell survival rates were calculated by reference to the 37 °C + NS group. The half-maximal drug inhibitory concentration (IC50) was calculated from the dose-responsive viability curves. Each experiment was repeated three times.
Temperature rising curve
Following addition of 500 μl DMEM into each well of 48-well plates, a microwave needle fixed with a temperature measuring probe was inserted into the well. The microwave therapy device was adjusted to 15 W under physiotherapy mode, and the starting temperature of each well was 37 °C. The microwave time was set at 15, 20, 25, 30, 40, 50 and 60 s. The microwave treatment was repeated three times for each treatment time. The temperature of the DMEM was recorded and the time-temperature curve was plotted.
Cell counting kit-8 (CCK-8) assays
HepG2 and Huh7 cells were seeded into 96-well plates (2 × 104 cells/well) and cultured at 37 °C with 5% CO2 overnight for attachment. Next, HepG2 cells and Huh7 cells were pretreated with 13.9 nM SB525334 and 15.6 nM SB525334, respectively, for 4 h and heated by microwave (15 W) for 40 s. Subsequently, serum-free DMEM (100 μl) containing 10 μl CCK-8 solution (Yeasen) was added to each well. After 2 h of incubation at 37 °C, the absorbance at 450 nm was measured using a microplate reader (Molecular Devices, Shanghai, China).
Flow cytometry
HepG2 and Huh7 cells were treated in the absence or presence of SB525334 for 4 h and then received microwave ablation with a power of 15 W and a duration time of 40 s. Subsequently, the cells were collected, washed twice with cold phosphate buffer saline (PBS) and stained with Annexin V-FITC and propidium iodide (PI) (Beyotime, Shanghai, China) for 30 min at 4 °C in the binding buffer. Cells were analyzed by fluorescence-activated cell sorting (FACS) with flow cytometer.
Western blotting
Total protein was extracted by ice-cold RIPA lysis buffer (MedChemExpress, Shanghai, China) containing protease inhibitor cocktails and phosphatase inhibitor cocktails. Then the samples were subjected to 12,000 g centrifugation for 10 min at 4 °C. The protein concentration was determined using a BCA assay kit (Yeasen, Shanghai, China). The extracted protein was added to a 5× loading buffer and boiled. Then, 20 μg of protein was separated by sodium dodecyl sulfate polyacrylamide gel electrophoresis and then transferred onto polyvinylidene fluoride membranes. The membranes were subsequently blocked using 5% skimmed milk at room temperature for 1 h, followed by incubation overnight at 4 °C with primary antibodies against cleaved caspase-3 (ab214430, 1:5000; Abcam), phosphorylated Smad3 (ab52903, 1:2000; Abcam), cleaved caspase-9 (#9507, 1:1000; Cell Signaling Biotechnology), Smad2 (ab40855, 1:2000; Abcam), cleaved PARP (Ab32064, 1:3000; Abcam), GAPDH (ab9485, 1:2500; Abcam), TGF-β1 (ab215715, 1:1000; Abcam), phosphorylated Smad2 (ab188334, 1:1000; Abcam), and Smad3 (ab40854, 1:2000; Abcam). Subsequently, the membranes were washed with PBS and then incubated with secondary antibodies at room temperature for 2 h. The immunoblots were visualized using enhanced chemiluminescence (Yeasen). To obtain the optical densities of signals, the digital images were analyzed by ImageJ.
Xenograft animal model
BALB/c nude mice (male, 5–6 weeks of age) were obtained from Charles River Laboratories (Beijing, China) and housed in an environmentally controlled breeding room (22 ± 2 °C, 55 ± 5% humidity, 12-h light/dark cycle). All mice were housed individually in stainless steel cages with free access to food and water. All procedures were performed under aseptic conditions and isoflurane anesthesia. All animal studies were approved by the Animal Care and Use Committee of Hubei Provincial Center for Disease Control and Prevention (202410052).
The HepG2 cells (1 × 106 cells) were suspended in 0.2 ml PBS and then injected into the center of the left hepatic lobe. When the maximum diameter of tumors reached 7–9 mm after one week, mice were randomly divided into four groups: control + normal saline (NS) group, control + SB525334 (TGF-β1 inhibitor) group, MWA + NS group and MWA + SB525334 group. Each group had five mice. The mice then received oral gavage of SB525334 (20 mg/kg) or the same volume of normal saline every other day. The SB525334 concentration was chosen based on previous findings [Citation33]. MWA was conducted on a microwave ablation apparatus, with an antenna having an active tip length of 5 mm (Canyou Medical, Nanjing, China). The antenna was placed in the non-central site of liver tumors and set to an output power of 15 W for 40 s. The mice were sacrificed at 3 weeks after MWA, and the ablated liver lobe was isolated for histopathological examination to assess macrophage recruitment. Tumor size was measured every other day with a caliper and calculated with the following equation: Tumor volume = (length × width2)/2.
Immunohistochemistry staining
The transplanted tumor tissues were fixed in 4% formaldehyde, embedded in paraffin and sectioned in 4 μm thickness, followed by being dewaxed to water and incubated in 3% H2O2 for 10 min. After blocking with 10% goat serum for 20 min at room temperature, the sections were incubated with an anti-Ki67 antibody (ab16667, 1:200; Abcam) in PBS buffer overnight at 4 °C. Next, the sections were incubated with a corresponding secondary antibody at 37 °C for 20 min. Afterwards, HRP-conjugated streptavidin and 3,3′-diaminobenzidine solution (Beyotime) were added to the slides and developed for 5 min. After being rinsed with tap water for 10 min, the sections were counterstained with hematoxylin (Beyotime) for 2 min at room temperature and differentiated with hydrochloric acid alcohol. A fluorescence microscope (Olympus, Tokyo, Japan) was used to observe the samples.
Hematoxylin-eosin staining
The tumor tissues were fixed in 10% formaldehyde, dehydrated in graded ethanol, embedded in paraffin and sliced at 5 μm. Then, the tissue samples were dewaxed in xylene, rehydrated in graded ethanol and staining with hematoxylin (Beyotime) for 5 min and eosin (Beyotime) for 2 min. After dehydration with graded ethanol and vitrification with dimethylbenzene, the tissue samples were observed under a microscope (Olympus).
Terminal deoxynucleotide transferase dUTP nick end labeling (TUNEL)
TUNEL assay was performed to assess apoptosis in tumor tissues using an EduTP TUNEL cell detection kit (Ribobio, Guangzhou, China). Briefly, the paraffin-embedded sections (5 μm) were cut and mounted on glass slides, and then incubated with the TUNEL reaction mixture for 1 h at 37 °C. Nuclei were observed by DAPI (Sigma–Aldrich) staining. Sections were observed under a light microscope (Olympus).
Statistics analysis
All experiments were performed three times. All results were analyzed by GraphPad Prism and reported as the mean ± standard deviation (SD). One way analysis of variance followed by Tukey’s post hoc analysis and Student’s t test were used for statistical analysis. p < .05 was considered as statistically significant.
Results
MWA combined with TGF-β1 inhibitor decreases cell survival
Schematic diagram depicting how MWA combined with TGF-β1 inhibitor (SB-525334) was shown in . The temperature and time were linearly related when the power was 15 W. The time required to reach the temperature of 40, 50 and 60 °C were 10–15 s, 35–40 s and 55–60 s, respectively (). The IC50 value was measured using the MTT assays. The results revealed that in HepG2 and Huh7 cells, the IC50 value of SB-525334 was 13.9 and 15.6 nM, respectively (). The MTT results also revealed that the viability of HepG2 and Huh7 cells was significantly impaired at 50 °C and 60 °C. The addition of SB-525334 at 37 °C and 40 °C had no significant effect on the survival of HepG2 and Huh7 cells. When the temperature was 50 °C, the survival rate of HCC cells was remarkably declined after treatment with SB-525334 compared with that in the counterpart (50 °C + NS group). Moreover, the cell survival in the 60 °C group was dramatically lower than the other temperature groups (). Thus, HepG2 cells treated with SB-525334 (13.9 nM) and Huh7 cells treated with SB525334 (15.6 nM) at 50 °C were used in the subsequent study.
MWA combined with TGF-β1 inhibitor promotes HCC cell apoptosis
HepG2 and Huh7 cells were pretreated with SB-525334 4 h prior to the MWA. As CCK-8 assays revealed, the viability rate of HepG2 and Huh7 cells was significantly reduced when exposed to SB-525334 or MWA alone. MWA combined with SB-525334 treatment further strengthened the suppressive effect of MWA on cell viability (). The results of flow cytometry demonstrated that SB-525334 or MWA alone markedly increased the apoptotic rate of HepG2 and Huh7 cells, whereas MWA combined with SB-525334 treatment further strengthened the apoptosis enhancing effect of MWA alone (). Moreover, the protein levels of cleaved caspase-3, capase-9 and PARP were remarkably upregulated in HepG2 and Huh7 cells after SB-525334 or MWA treatment, which were further increased by treatment of MWA combined with SB-525334 (). Taken together, MWA treatment combined with TGF-β1 inhibitor exerts more apoptosis enhancing effect than MWA or TGF-β1 inhibitor alone.
Figure 2. MWA combined with TGF-β1 inhibitor promotes the apoptosis of HCC cells. (A) CCK-8 assays to evaluate cell viability in the control (37 °C) + NS, the control (37 °C) + SB, the MWA (50 °C) + NS and the MWA (50 °C) + SB groups. (B–C) The apoptosis of HepG2 and Huh7 cells was assessed via flow cytometry. (D–E) Western blotting to measure the protein levels of cleaved caspase-3, caspase-9 and PARP in HepG2 and Huh7 cells. Data were analyzed by one-way analysis of variance followed by Tukey’s post hoc analysis and expressed as mean ± SD of three independent experiments. *p < .05, **p < .01, ***p < .01.
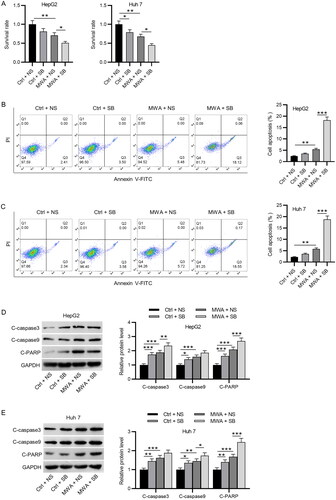
MWA combined with TGF-β1 inhibitor effectively inactivates the TGF-β1/Smad2/Smad3 pathway in HCC cells
Then, we investigated the mechanisms by which MWA combined with TGF-β1 promoted cell apoptosis. Both SB-525334 and MWA treatment significantly downregulated the protein levels of TGF-β1, phosphorylated Smad2 and phosphorylated Smad3 in HepG2 and Huh7 cells, whereas MWA combined with SB-525334 strengthened the inhibitory effect of MWA alone on the levels of pathway-related proteins, as western blotting revealed ().
Figure 3. TGF-β1 inhibitor effectively inactivates the TGF-β1/Smad2/Smad3 pathway in HCC cells. (A–B) Western blotting to assess the protein levels of TGF-β1, phosphorylated Smad2, and phosphorylated Smad3 in HepG2 and Huh7 cells. Data were analyzed by one way analysis of variance followed by Tukey’s post hoc analysis and expressed as mean ± SD of three independent experiments. *p < .05, **p < .01, ***p < .001.
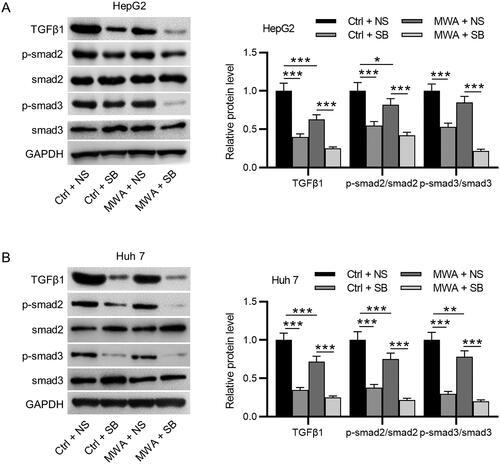
MWA combined with TGF-β1 inhibitor inhibits tumor growth in vivo
Next, we investigated the function of MWA combined with TGF-β1 inhibitor in the xenograft model of HCC. The mice injected with HepG2 cells were subjected to oral gavage of SB-525334 (20 mg/kg) or normal saline and MWA at a power of 15 W. The tumor images were presented in . Tumor size was significantly suppressed by SB-525334 or MWA alone, while MWA combined with SB-525334 further enhanced the suppressive effect of MWA alone on tumor growth (). Immunohistochemistry staining was performed to evaluate Ki67 expression, showing that SB-525334 or MWA alone partially decreased the number of cells positive for Ki67, whereas the inhibitory impact of MWA alone was further strengthened by SB525334 (). The histopathological changes in the tumor tissues in the HepG2 xenograft mouse model was evaluated by hematoxylin and eosin staining. Obvious tumor infiltration and angiogenesis were observed in tumor tissues of the control group. However, SB-525334 or MWA alone treatment significantly decreased tumor infiltration area and blood vessel density and increased the percentage of tumor cells with nucleus deformation and cells with condensed chromatin in tumor tissues, whereas MWA combined with SB-525334 had the more suppressive effect on the histopathological changes than MWA alone (). Finally, the apoptotic cells in the tumor sections of mice were visualized by TUNEL staining. SB-525334 or MWA alone led to a significant increase in the number of TUNEL-positive cells, whereas the apoptosis promoting effect of MWA alone was enhanced by MWA combined with SB-525334 (). These findings indicate that TGF-β1 inhibitor enhances the tumor suppressive effect of MWA.
Figure 4. MWA combined with TGF-β1 inhibitor inhibits tumor growth and histopathological changes in vivo. (A) The gross manifestation of tumors on day 22 after MWA. (B) Changes in tumor volume were recorded at the indicated time points. (C) Immunohistochemistry staining was used to detect the expression of Ki67. (D) Histopathological changes of tumor tissues were examined by hematoxylin and eosin staining, and quantification of tumor infiltration area, blood vessel density, cells with nuclear collapse and cells with condensed chromatin was conducted. Black and red arrows represent blood vessels and tumor infiltration, respectively. Blue arrow represents nuclear deformation and chromatin condensation. (E) TUNEL staining was performed to assess tumor apoptosis. Data were analyzed by one way analysis of variance followed by Tukey’s post hoc analysis and expressed as mean ± SD of three independent experiments. N = 5 mice each group. *p < .05, **p < .01, ***p < .001.
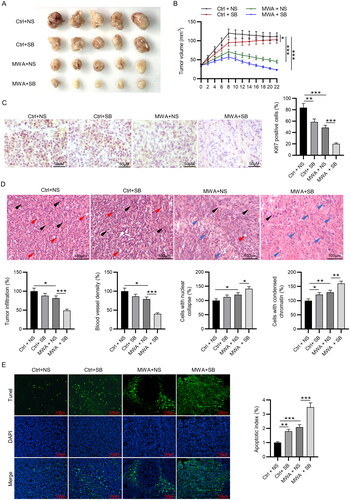
MWA combined with TGF-β1 inhibitor promotes cell apoptosis by inactivating the TGF-β1/Smad2/Smad3 in vivo
When treated with MWA or SB-525334 alone, the protein levels of cleaved caspase-3, caspase-9 and PARP were significantly upregulated in tumor tissues. Notably, MWA combined with SB-525334 further increased the protein levels of cleaved caspase-3, caspase-9 and PARP compared with those in the MWA alone group (). Finally, as western blotting demonstrated, MWA or SB-525334 alone significantly decreased the protein levels of TGF-β1, phosphorylated Smad2 and phosphorylated Smad3, which were further downregulated by MWA combined with SB-525334 treatment ().
Figure 5. MWA combined with TGF-β1 inhibitor promotes the apoptosis of tumor cells by inactivating the TGF-β1/Smad2/Smad3 in vivo. (A–B) Western blotting to measure the protein levels of cleaved caspase-3, cleaved caspase-9 and cleaved PARP. (C–D) Western blotting to measure the protein levels of TGF-β1, phosphorylated Smad2 and phosphorylated Smad3. Data were analyzed by one-way analysis of variance followed by Tukey’s post hoc analysis and expressed as mean ± SD of three independent experiments. N = 3 mice each group. *p < .05, **p <.01, ***p < .001.
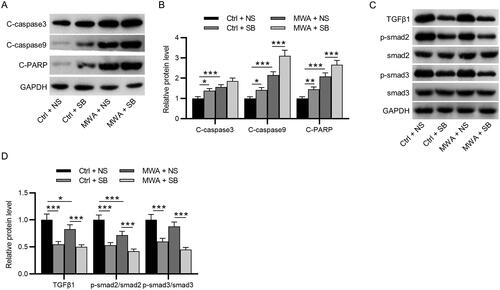
Discussion
MWA is suggested to be a safe and efficient therapeutic option for tumors less than 3 cm. However, insufficient MWA develops relapse in the tumor transitional zone, while extensive ablation often leads to high risk of damage to important organs [Citation10,Citation11]. Therefore, methods that can improve the therapeutic efficacy of MWA for HCC treatment is necessary. The tumor immune microenvironment is a novel concept that is closely related to the clinical prognosis of patients with HCC [Citation34]. The proportions of immunosuppressive cells are significantly increased in the tumor immune microenvironment, thereby contributing to tumor immune suppression [Citation35,Citation36]. Accumulating evidence has shown that the TGF-β signaling is involved in the regulation of immunosuppressive microenvironment [Citation37,Citation38]. MWA used with cell immunotherapies (anti-PD-1/anti-CTLA-4) has collaborative effects on HCC model in mice [Citation19]. Thus, the combination of MWA and immunotherapy has been a subject of recent clinical and basic research. In the current study, we investigated the efficacy of combination of MWA and TGF-β1 inhibitor in HCC. MWA combined with TGF-β1 inhibitor can significantly strengthen the anti-tumor effect of MWA alone as indicated by following findings: a) MWA combined with SB-525334 significantly increased the apoptotic rate of HCC cells and upregulated the protein levels of apoptotic markers compared to MWA alone; b) MWA combined with SB-525334 further reduced the Ki67 expression and increased the number of TUNEL-positive cells compared to MWA alone; c) MWA combined with SB-525334 significantly decreased the tumor infiltration area and blood vessel density and increased the percentages of cells with nuclear deformation and cells with condensed chromatin compared to MWA alone; d) MWA combined with SB-525334 enhanced the suppressive effect of MWA alone on tumor growth; e) and MWA combined with SB-525334 inactivated the TGF-β1/Smad2/Smad3 pathway in vivo and in vitro.
MWA has been found to increase CD3 + T cells, CD4 + T cells and IL-2 in peripheral blood of HCC patients one month after treatment. Additionally, IL-4 and IL-10 levels are decreased after MWA, indicating that MWA relieves immunosuppression in HCC patients [Citation39]. Besides, MWA increases Th17 cells in HCC patients, and high frequency of circulating Th17 cells is associated with tumor recurrence [Citation40]. Previously studies have shown that the combination of MWA and immunotherapies (anti-PD-1 antibody, anti-CTLA-4 antibody, and VEGFR-2 inhibitor) has the potential to treat HCC [Citation19,Citation20,Citation41]. Thus, novel strategies on immunomodulation after MWA should be developed. TGF-β1 participates in regulating cell differentiation and immune homeostasis. As reported, high TGF-β1 expression can inhibit antitumor immunity, inducing poor prognosis [Citation42,Citation43]. TGF-β1 silencing leads to a significant reduction in HCC cell malignancies [Citation44]. MWA collaborated with decorin decreases formation of hard nodules in a model of mammary gland hyperplasia by inhibiting the TGF-β1/Smad and MAPK pathways [Citation45]. Additionally, combination of MWA and TGF-β1 and HSP90 inhibitors can enhance the therapeutic effect on osteosarcoma [Citation46]. In the current study, we found that MWA or SB-525334 alone could effectively impair HCC cell viability and enhance cell apoptosis in vitro, while MWA combined with SB-525334 enhanced the apoptosis promoting effect of MWA alone. For in vivo analysis, MWA combined with SB-525334 promoted the inhibitory effect of MWA alone on tumor infiltration and angiogenesis and tumor growth and enhanced the promoting effect of MWA alone on the apoptosis of tumor cells. Mechanistically, the active TGF-β binds to the serine/threonine protein kinase receptors TGF-βR1 and TGF-βRII, and the activated receptor complex then phosphorylates specific Smads, a family of signal-transduction proteins. These activated Smad complexes interact with cell-specific transcription factors in the nucleus. The Smad2/Smad3-depdent TGF-β pathway is involved in the regulation of immunosuppression in HCC [Citation37,Citation47]. Here, we found that MWA combined with SB-525334 decreased Smad2 and Smad3 phosphorylation in HCC cells and xenograft models.
In conclusion, this study suggests that microwave ablation combined with TGF-β1 inhibitor promotes the apoptosis of tumor cells and prevents tumor growth by inactivating the TGF-β1/Smad2/Smad3 pathway. To be honest, there are limitations to this study. First, more function experiments are required to detect the roles of MWA combined with TGF‑β1 inhibitor in cell migration, invasion and EMT process. Second, other animal species and even clinical practice are required to uncover the clinical influence of application of MWA combined with TGF‑β1 inhibitor for HCC treatment. Despite these limitations, we believe that this study would provide evidence for the potential beneficial effects of TGF-β1 on improving the therapeutic efficacy of MWA for HCC treatment.
Ethical approval
Animal experiments were approved by Hubei Provincial Center for Disease Control and Prevention (202410052).
Acknowledgements
The authors appreciate the help of The First Affiliated Hospital of Yangtze University.
Disclosure statement
No potential conflict of interest was reported by the author(s).
Data availability statement
The datasets used or analyzed during the current study are available from the corresponding author on reasonable request.
Additional information
Funding
References
- Forner A, Reig M, Bruix J. Hepatocellular carcinoma. Lancet. 2018;391(10127):1–11.
- Ferlay J, Soerjomataram I, Dikshit R, et al. Cancer incidence and mortality worldwide: sources, methods and major patterns in GLOBOCAN 2012. Int J Cancer. 2015;136(5):E359–86.
- Yang JD, Hainaut P, Gores GJ, et al. A global view of hepatocellular carcinoma: trends, risk, prevention and management. Nat Rev Gastroenterol Hepatol. 2019;16(10):589–604. doi: 10.1038/s41575-019-0186-y.
- EASL clinical practice guidelines: management of hepatocellular carcinoma. J Hepatol. 2018;69(1):182–236.
- Chu KF, Dupuy DE. Thermal ablation of tumours: biological mechanisms and advances in therapy. Nat Rev Cancer. 2014;14(3):199–208. doi: 10.1038/nrc3672.
- Liang P, Yu J, Yu X-L, et al. Percutaneous cooled-tip microwave ablation under ultrasound guidance for primary liver cancer: a multicentre analysis of 1363 treatment-naive lesions in 1007 patients in China. Gut. 2012;61(7):1100–1101. doi: 10.1136/gutjnl-2011-300975.
- Zhang NN, et al. High-powered microwave ablation of larger hepatocellular carcinoma: evaluation of recurrence rate and factors related to recurrence. Clin Radiol. 2015;70(11):1237–1243.
- Yu J, Liang P, Yu X-L, et al. Local tumour progression after ultrasound-guided microwave ablation of liver malignancies: risk factors analysis of 2529 tumours. Eur Radiol. 2015;25(4):1119–1126. doi: 10.1007/s00330-014-3483-4.
- Teratani T, Yoshida H, Shiina S, et al. Radiofrequency ablation for hepatocellular carcinoma in so-called high-risk locations. Hepatology. 2006;43(5):1101–1108. doi: 10.1002/hep.21164.
- Huang S, et al. Percutaneous microwave ablation for hepatocellular carcinoma adjacent to large vessels: a long-term follow-up. Eur J Radiol. 2014;83(3):552–558.
- Ren H, et al. Treatment of liver tumours adjacent to hepatic hilum with percutaneous microwave ablation combined with ethanol injection: a pilot study. Int J Hyperthermia. 2011;27(3):249–254.
- El Dika I, Makki I, Abou-Alfa GK. Hepatocellular carcinoma, novel therapies on the horizon. Chin Clin Oncol. 2021;10(1):12–12. doi: 10.21037/cco-20-113.
- Sapisochin G, Bruix J. Liver transplantation for hepatocellular carcinoma: outcomes and novel surgical approaches. Nat Rev Gastroenterol Hepatol. 2017;14(4):203–217. doi: 10.1038/nrgastro.2016.193.
- Singal AG, Nehra M, Adams-Huet B, et al. Detection of hepatocellular carcinoma at advanced stages among patients in the HALT-C trial: where did surveillance fail? Am J Gastroenterol. 2013;108(3):425–432. doi: 10.1038/ajg.2012.449.
- Kalogeridi MA, et al. Role of radiotherapy in the management of hepatocellular carcinoma: a systematic review. World J Hepatol. 2015;7(1):101–112.
- Rinninella E, Cerrito L, Spinelli I, et al. Chemotherapy for hepatocellular carcinoma: current evidence and future perspectives. J Clin Transl Hepatol. 2017;5(3):235–248.
- Rabinovich GA, Gabrilovich D, Sotomayor EM. Immunosuppressive strategies that are mediated by tumor cells. Annu Rev Immunol. 2007;25:267–296.
- Machlenkin A, Goldberger O, Tirosh B, et al. Combined dendritic cell cryotherapy of tumor induces systemic antimetastatic immunity. Clin Cancer Res. 2005;11(13):4955–4961. doi: 10.1158/1078-0432.CCR-04-2422.
- Duan X, Wang M, Han X, et al. Combined use of microwave ablation and cell immunotherapy induces nonspecific immunity of hepatocellular carcinoma model mice. Cell Cycle. 2020;19(24):3595–3607. doi: 10.1080/15384101.2020.1853942.
- Li X, Zhang Q, Lu Q, et al. Microwave ablation combined with apatinib and camrelizumab in patients with advanced hepatocellular carcinoma: a single-arm, preliminary study. Front Immunol. 2022;13:1023983. doi: 10.3389/fimmu.2022.1023983.
- Dituri F, Mancarella S, Cigliano A, et al. TGF-β as multifaceted orchestrator in HCC progression: signaling, EMT, immune microenvironment, and novel therapeutic perspectives. Semin Liver Dis. 2019;39(1):53–69. doi: 10.1055/s-0038-1676121.
- Larson C, Oronsky B, Carter CA, et al. TGF-beta: a master immune regulator. Expert Opin Ther Targets. 2020;24(5):427–438.
- van den Bulk J, de Miranda N, Ten Dijke P. Therapeutic targeting of TGF-β in cancer: hacking a master switch of immune suppression. Clin Sci (Lond). 2021;135(1):35–52. doi: 10.1042/CS20201236.
- de Streel G, Lucas S. Targeting immunosuppression by TGF-β1 for cancer immunotherapy. Biochem Pharmacol. 2021;192:114697.
- Wang B, Liu T, Wu JC, et al. STAT3 aggravates TGF-β1-induced hepatic epithelial-to-mesenchymal transition and migration. Biomed Pharmacother. 2018;98:214–221.
- Sun H, Huang Q, Huang M, et al. Human CD96 correlates to natural killer cell exhaustion and predicts the prognosis of human hepatocellular carcinoma. Hepatology. 2019;70(1):168–183. doi: 10.1002/hep.30347.
- Jin X, Zhang S, Wang N, et al. High expression of TGF-β1 contributes to hepatocellular carcinoma prognosis via regulating tumor immunity. Front Oncol. 2022;12:861601. doi: 10.3389/fonc.2022.861601.
- Luo Y, Huang K, Zheng J, et al. TGF-β1 promotes cell migration in hepatocellular carcinoma by suppressing reelin expression. Gene. 2019;688:19–25. doi: 10.1016/j.gene.2018.11.033.
- Huang J, Qiu M, Wan L, et al. TGF-β1 promotes hepatocellular carcinoma invasion and metastasis via ERK pathway-mediated FGFR4 expression. Cell Physiol Biochem. 2018;45(4):1690–1699. doi: 10.1159/000487737.
- Sun SL, Wang XY. TGF-β1 promotes proliferation and invasion of hepatocellular carcinoma cell line HepG2 by activating GLI-1 signaling. Eur Rev Med Pharmacol Sci. 2018;22(22):7688–7695.
- Tu J, Zhao Z, Xu M, et al. NEAT1 upregulates TGF-β1 to induce hepatocellular carcinoma progression by sponging hsa-mir-139-5p. J Cell Physiol. 2018;233(11):8578–8587.
- Wegner K, Bachmann A, Schad JU, et al. Dynamics and feedback loops in the transforming growth factor β signaling pathway. Biophys Chem. 2012;162:22–34.
- Heo J-Y, Do J-Y, Lho Y, et al. TGF-β1 receptor inhibitor SB525334 attenuates the epithelial to mesenchymal transition of peritoneal mesothelial cells via the TGF-β1 signaling pathway. Biomedicines. 2021;9(7):839. doi: 10.3390/biomedicines9070839.
- Roth GS, Decaens T. Liver immunotolerance and hepatocellular carcinoma: patho-physiological mechanisms and therapeutic perspectives. Eur J Cancer. 2017;87:101–112.
- Mao X, Xu J, Wang W, et al. Crosstalk between cancer-associated fibroblasts and immune cells in the tumor microenvironment: new findings and future perspectives. Mol Cancer. 2021;20(1):131. doi: 10.1186/s12943-021-01428-1.
- Zhang Y, Lazarus J, Steele NG, et al. Regulatory T-cell depletion alters the tumor microenvironment and accelerates pancreatic carcinogenesis. Cancer Discov. 2020;10(3):422–439. doi: 10.1158/2159-8290.CD-19-0958.
- Batlle E, Massagué J. Transforming growth factor-β signaling in immunity and cancer. Immunity. 2019;50(4):924–940. doi: 10.1016/j.immuni.2019.03.024.
- Stuelten CH, Zhang YE. Transforming growth factor-β: an agent of change in the tumor microenvironment. Front Cell Dev Biol. 2021;9:764727. doi: 10.3389/fcell.2021.764727.
- Zhang H, Hou X, Cai H, et al. Effects of microwave ablation on T-cell subsets and cytokines of patients with hepatocellular carcinoma. Minim Invasive Ther Allied Technol. 2017;26(4):207–211.
- Zhou Y, Xu X, Ding J, et al. Dynamic changes of T-cell subsets and their relation with tumor recurrence after microwave ablation in patients with hepatocellular carcinoma. J Cancer Res Ther. 2018;14(1):40–45. doi: 10.4103/jcrt.JCRT_775_17.
- Huang S, Li T, Chen Y, et al. Microwave ablation combined with anti-PD-1 therapy enhances systemic antitumor immunity in a multitumor murine model of Hepa1-6. Int J Hyperthermia. 2022;39(1):278–286.
- Budhu S, Schaer DA, Li Y, et al. Blockade of surface-bound TGF-β on regulatory T cells abrogates suppression of effector T cell function in the tumor microenvironment. Sci Signal. 2017;10(494):10. doi: 10.1126/scisignal.aak9702.
- Wang Y, Liu T, Tang W, et al. Hepatocellular carcinoma cells induce regulatory T cells and lead to poor prognosis via production of transforming growth factor-β1. Cell Physiol Biochem. 2016;38(1):306–318. doi: 10.1159/000438631.
- Fezza M, Moussa M, Aoun R, et al. DKK1 promotes hepatocellular carcinoma inflammation, migration and invasion: implication of TGF-β1. PLoS One. 2019;14(9):e0223252. doi: 10.1371/journal.pone.0223252.
- Du Y, Liu X, Du K, et al. Decorin inhibits the formation of hard nodules after microwave ablation by inhibiting the TGF-β1/SMAD and MAPK signaling pathways: in a bama miniature pig model of mammary gland hyperplasia. Int J Hyperthermia. 2023;40(1):2188151.
- Chen L, Wang M, Lin Z, et al. Mild microwave ablation combined with HSP90 and TGF‑β1 inhibitors enhances the therapeutic effect on osteosarcoma. Mol Med Rep. 2020;22(2):906–914. doi: 10.3892/mmr.2020.11173.
- Chen J, Gingold JA, Su X. Immunomodulatory TGF-β signaling in hepatocellular carcinoma. Trends Mol Med. 2019;25(11):1010–1023.