Abstract
Purpose
To evaluate the safety and efficacy of performing histotripsy through overlying gas-filled bowel in an ex vivo swine model.
Methods
An ex vivo model was created to simulate histotripsy treatment of solid organs through gas-filled bowel. Spherical 2.5 cm histotripsy treatments were performed in agar phantoms for each of five treatment groups: 1) control with no overlying bowel (n = 6), 2) bowel 0 cm above phantom (n = 6), 3) bowel 1 cm above phantom (n = 6), 4) bowel 2 cm above phantom (n = 6), and 5) bowel 0 cm above the phantom with increased treatment amplitude (n = 6). Bowel was inspected for gross and microscopic damage, and treatment zones were measured. A ray-tracing simulation estimated the percentage of therapeutic beam path blockage by bowel in each scenario.
Results
All histotripsy treatments through partial blockage were successful (24/24). No visible or microscopic damage was observed to intervening bowel. Partial blockage resulted in a small increase in treatment volume compared to controls (p = 0.002 and p = 0.036 for groups with bowel 0 cm above the phantom, p > 0.3 for bowel 1 cm and 2 cm above the phantom). Gas-filled bowel was estimated to have blocked 49.6%, 35.0%, and 27.3% of the therapeutic beam at 0, 1, and 2 cm, respectively.
Conclusion
Histotripsy has the potential to be applied through partial gas blockage of the therapeutic beam path, as shown by this ex vivo small bowel model. Further work in an in vivo survival model appears indicated.
Introduction
Histotripsy is an emerging non-thermal, non-ionizing, and noninvasive focused ultrasound technique recently approved for use to treat liver tumors. High amplitude microsecond ultrasound pulses create inertial cavitation and mechanical destruction at a focal point [Citation1]. The resultant rapid expansion and collapse of gas bubbles causes high mechanical stress and strain on targeted tissues, resulting in disruption of cell membranes, organelles, and eventually tissue liquefaction [Citation2,Citation3]. To date abdominal histotripsy has been studied in pre-clinical models for treatment of liver, spleen, pancreas, kidney, and prostate, and early human feasibility trials have demonstrated safety for prostate and liver treatments. Current clinical trials are underway in the liver and kidney [Citation4–10]. Of note, the United States Food and Drug Administration granted approval for liver histotripsy in October 2023 [Citation11].
The safety of treating through gas-filled bowel with histotripsy needs to be demonstrated prior to widespread application to organs in the abdomen and pelvis. Rare bowel perforations due to thermal injury have been described with high intensity focused ultrasound (HIFU) which raises a general concern about energy delivery through the bowel with focused ultrasound [Citation12–15]. To date, liver histotripsy treatments have been safely performed with bowel in the most peripheral portions of the therapeutic beam path and proximal to the focal point [Citation4,Citation16]. However, treatments with bowel centered within the beam or near the focal point have been avoided due to an uncertain safety profile. The differences in mechanism of action of histotripsy vs. HIFU and the binary threshold effect inherent in tissue cavitation may more readily allow safe histotripsy treatments through bowel, but this effect has not been systematically studied [Citation3]. Therefore the purpose of this ex vivo proof-of-principle study is to determine if gas-filled bowel within the therapeutic beam path proximal to the focal point: 1) results in damage to the bowel, and 2) alters the treatment effect in the target organ.
Materials and methods
Experimental design
An ex vivo system was created to study the effects of histotripsy treatment through gas-filled small bowel with a clinical-grade therapy system (). Porcine small bowel was harvested, inflated with room air, and suspended above an agar phantom in a degassed water bath. Histotripsy treatments were created in phantoms and measured, and the bowel was inspected for signs of damage.
Figure 1. Experimental design five treatment groups were used to investigate the effects of histotripsy treatment through gas blockage. From left: Control treatments without blockage, bowel positioned 0 cm above the agar phantom at both normal and double amplitude, and bowel positioned 1 and 2 cm above the phantom. The therapeutic beam path is denoted by the dotted lines. A 2.5 cm diameter spherical treatment (red circle) was prescribed in each phantom. Following histotripsy treatment, the bowel was inspected for gross and microscopic signs of damage.
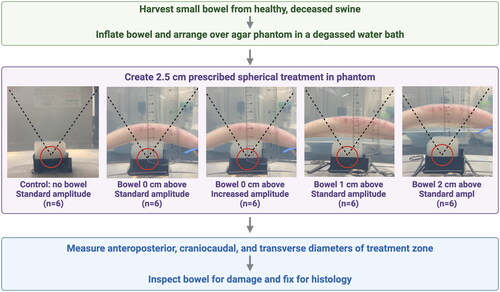
Bowel preparation
Small bowel (ileum) was obtained from recently deceased healthy swine and stored in 0.9% saline at 4 °C until treatment. All animals were sacrificed according to approved protocols for other purposes, and no additional institutional approval was required to obtain tissue following sacrifice. The mean delay time between harvesting and treatment was 5.1 ± 2.0 days. For histological evaluation, bowel was harvested, treated, and fixed on the same day. Prior to treatment, the ileum was cut into 20 cm sections, filled with approximately 60 ml of room air, and both ends secured with an elastic cord to maintain the air within the bowel loop.
Treatment Phantoms
Agar phantoms were used as generic soft tissue models due to the visibility of histotripsy treatment zones [Citation17]. Phantoms were prepared by dissolving agar in heated water to create a 1.5% solution [Citation17]. The heated liquid mixture was degassed in a vacuum chamber before pouring into 4.6 × 4.6 × 4.6 cm molds to set for at least 1 h at 4 °C. Phantoms were used within 24 h of preparation.
Experimental setup
Histotripsy was performed with a custom clinical therapy transducer (Vortx Rx, Histosonics, Inc.; 700 kHz, 20 cm diameter, 18-element transducer with a 14 cm focal length and a coaxially aligned 3-MHz curvilinear array diagnostic transducer). To mimic the treatment of a solid organ with intervening gas-filled bowel, phantoms were placed in a degassed water bath centered at the focal point of the therapeutic ultrasound beam. Gas-filled bowel was arranged in the craniocaudal direction and secured via an elastic cord to an adjustable platform submerged in the water bath (). The bowel was positioned either 0, 1, or 2 cm directly overlying the phantom, partially blocking the central portion of the therapeutic ultrasound beam path (). Treatments were prescribed for the center of the phantom, approximately 1 cm from the anterior face of the phantom. These scenarios were chosen to mimic the clinical conditions that could arise when targeting the pancreas [Citation6,Citation7, Citation18–20].
Figure 2. Experimental setup. A) the treatment head (pink arrow) of a clinical-grade histotripsy system was positioned in a degassed water bath above an agar phantom (white arrows) with gas-filled small bowel (yellow arrows) partially blocking the therapeutic beam path. B) Bowel was positioned 0 cm above the phantom (white arrow), shown in the sagittal plane. C) An image from the co-axially mounted diagnostic ultrasound transducer shows the bowel (yellow arrow) positioned above the phantom (white arrow) in the axial plane, which has been obscured by the intraluminal gas.
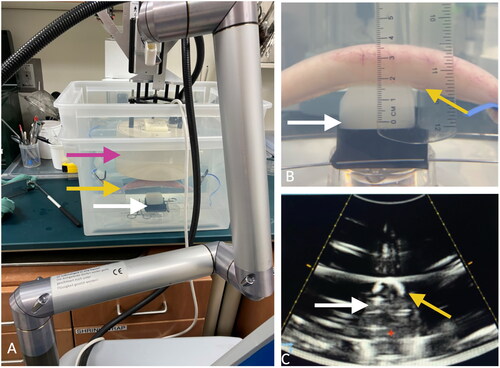
Treatment
Six treatments were performed for each of five groups: 1) control with no overlying bowel, 2) bowel 0 cm above the phantom (directly in contact with the phantom), 3) bowel 1 cm above the phantom, 4) bowel 2 cm above the phantom, and 5) bowel 0 cm above the phantom with treatment amplitude more than double required to form a treatment. The latter setup simulated a worst-case clinical scenario where excessive amplitudes were inadvertently utilized. For groups 2–5 (i.e., phantom with overlying bowel) the bubble cloud within the treatment phantom could not be visualized by ultrasound due to blockage of the diagnostic beam by gas-filled bowel. Therefore, the applied treatment amplitude was determined by an audible cavitation signature [Citation7,Citation21]. The driving amplitude was increased until the audible cavitation signature was observed and is reported as a percentage of the total possible amplitude allowed by the software. A 2.5 cm treatment sphere (volume 8.2 cm3) was prescribed in each phantom and treatment was completed in 13 min, 23 s. During treatment, bowel was visually monitored for any visible changes or indications of damage, including discoloration or development of air leaks or frank perforation. Continuous histotripsy treatment was verified by the presence of an audible cavitation signature and confirmed by the presence of visible pockets of cavitation in the phantom ().
Figure 3. Treated Phantoms. Phantoms (4.6 cm × 4.6 cm × 4.6 cm) were sliced twice following treatment to facilitate measurements in both the axial and sagittal planes. A) The AP and TRANS diameters were visible following a slice in the axial plane, illustrated by the drawing on the right. B) The AP and CC diameters in the same phantom were visible in the sagittal plane.
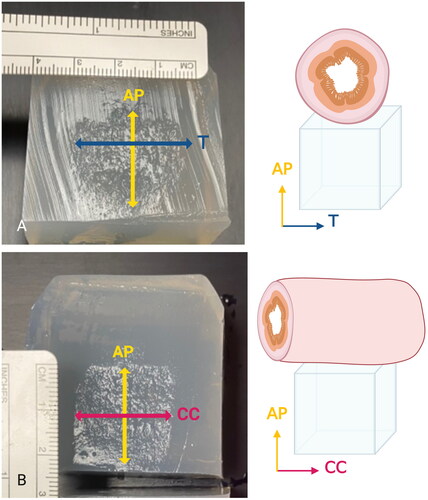
Measurement
Phantoms were sliced twice at orthogonal angles through the center of the treatment zone (). When sliced, the treated area of the agar phantom can be distinguished from untreated areas due to agar breakdown induced by cavitation (). The anteroposterior (AP), craniocaudal (CC), and transverse (TRANS) diameters of the treatment zone were manually measured, in addition to the depth of the treatment zone from the anterior face of the phantom. Volume of the treatment area was calculated by the formula: Volume=(π·D1·D2·D3)∕6.
Pathologic analysis
Following treatment the bowel was maintained in the water bath and inspected for any signs of gas leaking from the wall, both at rest and with manual external pressure applied. The small bowel was marked with ink at the center point, grossly inspected for damage, including pneumatosis, photographed, and placed in formalin. Following fixation for two weeks, the entire bowel circumference from 2 locations of each treated bowel loop were harvested and placed in a cassette: 1) Treatment group – Bowel adjacent to the center point which was in the therapeutic beam path; 2) Control group – Bowel distant from the center point outside of the therapeutic beam path. Tissue was processed with a Sakura Tissue-Tek VIP (Sakura Fintek, Torrance, CA), embedded with a Leica EB 1160 (Leica Biosystem, Richmond, IL), and sectioned to 5 microns using a Leica RM2125RT (Leica Biosystems, Richmond, IL). Hemotoxylin and eosin staining was performed for each slide. Histologic analysis was performed by a pathologist with ten years of experience (XZ).
Computer Simulation to estimate blockage
A ray tracing approach was used to estimate the percentage of therapeutic ultrasound blocked by gas-filled bowel (). The experimental setup was simulated in Matlab (R2021b, Mathworks, Natick, MA) using the known transducer geometry. The bowel was represented by a 2 cm diameter cylinder placed centrally, parallel to the face of the transducer in the CC direction. The distance between the transducer surface and target was 14 cm, where everything outside the bowel and transducer was assumed to be filled with degassed water. Three locations of the bowel above the phantom were simulated, where the distance between bowel and target was 1.8 cm plus 0, 1, or 2 cm, respectively. The percentage of the transducer surface that was blocked by bowel was determined using a ray-tracing approach. For each point on the transducer, a straight line to the target was calculated and the distance to the centerline of the cylinder representing the bowel was calculated. If the distance was smaller than the bowel radius, the corresponding point was assumed to be blocked. For the purposes of this simulation, bowel was presumed to cause 100% blockage of any ultrasound beam intersecting with it.
Figure 4. Computer simulation a ray-tracing approach was used to estimate the amount of the therapeutic transducer face (blue) blocked by gas-filled bowel (green) that extends in the CC direction. Yellow rays represent unobstructed therapeutic ultrasound that reaches the target while red rays represent blocked ultrasound intersecting with gas-filled bowel.
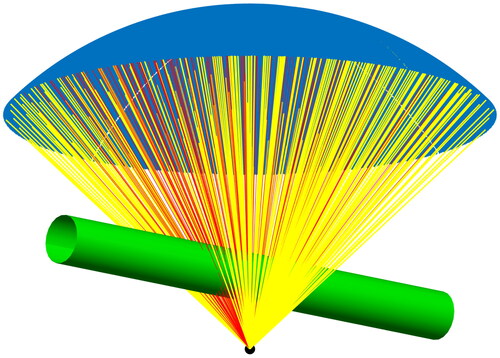
Statistical analysis
Comparisons of the three treatment zone diameters and the volume between the five treatment groups were calculated, where the p-values are based on the nonparametric Kruskal-Wallis test (with four degrees of freedom). The mean diameters and volumes for each of the five treatment groups were compared to each other and p-values were calculated using Tukey’s pairwise tests of means. The overall diameters of the treatments were determined by combining the AP, CC, and TRANS diameters and compared to the prescribed diameter (2.5 cm) using repeated measures (3 measures per unit) ANOVA.
Results
Bowel injuries (gross and histologic)
No gross or histologic injuries to the small bowel were observed following treatment. No air leaks or signs of pneumatosis were observed, either with the bowel at rest in the water bath or with externally applied pressure (0/24). Following fixation, the serosa was tan, pink, and glistening in all cases with no observable injury. There were no visible differences or signs of mechanical damage to treated vs. untreated sections of the bowel (). The small bowel in the treatment and control groups showed normal diameter and normal intestinal wall thickness (2–5 mm in thickness). Bowel wall in both treated and untreated groups showed four well-preserved layers, including mucosa, lamina propria, submucosa, and muscularis propria. The muscularis propria showed normal arrangement of inner circular and outer longitudinal layers with normal thickness for samples treated same day and those treated >1 day from harvesting. The mucosa layer in the treated tissue showed preserved villous architecture with normal villus height and normal villous-to-crypt ratio in the bowel treated same day. In bowel loops treated >1 day from harvesting there was varying loss of mucosal architecture which was equivalent between test and control samples.
Figure 5. Gross and microscopic pathology. A) bivalved small bowel sectioned longitudinally. There were no signs of visible damage to the small bowel mucosa following treatment. B) Treated tissue taken from the center point of the gross sample shows intact mucosa, lamina propria, submucosa, and muscularis propria (20× magnification, H&E). C) untreated control tissue harvested from bowel outside of the ultrasound beam demonstrates no signs of damage (20× magnification, H&E).
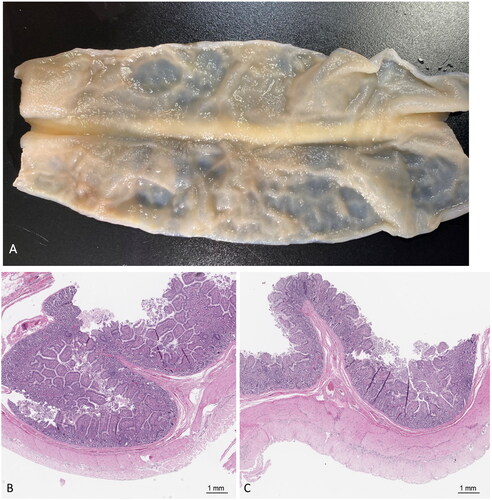
Treatment sizes in phantoms
Histotripsy treatment zones were successfully created in the phantoms in all treatment groups (). Treatments with blockage were 1.8 cm (SD = 0.7) deep to the top of the phantom. Mean diameters and volumes of the treatment zones are summarized in . There was a small but statistically significant difference between the prescribed and measured treatment diameters in all cases. The mean diameter for treatments with intervening bowel was 2.4 cm (0.2) (n = 24 treatments, p < 0.05 vs. prescribed diameter (2.5 cm)) while the mean diameter for the unobstructed, control treatments was 2.2 cm (0.2) (n = 6 treatments, p < 0.05 vs. prescribed diameter (2.5 cm)). With intervening bowel, mean diameters were 2.3 (0.3) × 2.4 (0.2) × 2.6 (0.2), AP, CC, and TRANS respectively.
Table 1. Summary of histotripsy treatment volume.
The two groups with bowel positioned 0 cm above the phantom had the largest mean diameters, which were not statistically different from the prescribed 2.5 cm diameter. The 0 cm standard amplitude group had an overall diameter of 2.4 cm (0.2) (n = 6 treatments, p > 0.05 vs. prescribed diameter (2.5 cm)), while the 0 cm group with increased amplitude had a mean diameter of 2.5 cm (0.2) (n = 6 treatments, p > 0.05 vs. prescribed diameter (2.5 cm)). Bowel positioned 1 cm and 2 cm from the phantom resulted in mean diameters of 2.3 cm (0.3) and 2.3 cm (0.2), respectively (n = 6 each, p < 0.05 vs. prescribed diameter (2.5 cm)).
Overall, there was a significant difference in volume and AP, CC, and TRANS diameters across treatment groups (p < 0.05). The mean TRANS diameters (perpendicular to the CC gas blockage) were significantly larger in both groups with bowel at 0 cm, (n = 6, p < 0.05 vs. control, for both groups).
Treatment amplitude
Mean amplitudes as a percentage of system maximum are summarized in . Higher amplitudes were required to produce histotripsy in the soft tissue phantom through gas blockage than without blockage. The mean amplitude for the treatment groups where bowel was positioned 0, 1, and 2 cm above the phantom were 15.7% (0.5), 15.3% (0.5), and 15.0% (0.0) respectively (p > 0.1 for 0 cm vs. 1 cm, 0 cm vs. 2 cm, and 1 cm vs. 2 cm). The control group required a mean amplitude of 7% of maximum (0.0) (p < 0.001 compared to all other treatment groups). A mean amplitude of 32.7% (1.4) was used for the group with increased amplitude and bowel positioned 0 cm above the phantom, (p < 0.001 compared to all other treatment groups).
Table 2. Summary of treatment amplitudes.
Degree of blockage
The ray-tracing simulation revealed that bowel blocked less than half of the transducer face in all treatment groups (). Assuming gas-filled bowel obstructs 100% of portions of the ultrasound beam that intersect with it, the treatment groups with bowel positioned 0 cm above the phantom had the highest degree of blockage (49.6%). The treatment groups with bowel positioned 1 cm and 2 cm above the phantom had a lesser degree of blockage, 35.0%, and 27.3% respectively.
Discussion
The results of this ex vivo study suggest that histotripsy has the potential to be performed safely and effectively in the presence of gas-filled small bowel partially blocking the therapeutic beam path. Importantly, there were no signs of damage at either the gross or microscopic levels and no loss of the integrity of the bowel wall, even when the amplitude was increased to levels double what was necessary to create histotripsy. As seen in prior studies, treatment zones were close to the prescribed diameters with highly precise margins [Citation22]. Though statistically significant, the slight increases in treatment diameters are unlikely to be clinically relevant.
Bowel injuries have been reported after other external beam therapies currently in clinical use – specifically HIFU and therapeutic radiation. Preclinical studies of pancreatic HIFU resulted in mild or moderate bowel injury in swine when the acoustic window was at least partially blocked by gas [Citation18–20]. In rare cases, HIFU has resulted in delayed bowel perforation, postulated to have resulted from direct contact with the thermal ablation zone or due to the inclusion of bowel in the therapeutic acoustic beam path [Citation12–15]. Bowel within a radiation field often results in either acute or chronic radiation enteritis due to mucosal or submucosal and vascular damage [Citation23–26]. Due to the mechanical nature of histotripsy, cavitation injuries would be expected to be immediately visible following treatment [Citation3]. In this study the treatment zone came within 1.8 ± 0.7 cm of the bowel loop which could provide some guidance on potentially safe clinical depths relative to bowel. Direct treatment of bowel needs to be further assessed before drawing any clinical inference for that scenario. One caveat is that thermal injuries caused by the transformation of acoustic energy into heat are not easily detected in this model, and a chronic live animal study will eventually be required for confirmation.
The mechanism of energy delivery and unique properties of cavitation give histotripsy potential advantages over other ablation modalities when treating near vulnerable structures. Cavitation results from low-duty cycle, high amplitude ultrasound pulses to create microscopic bubbles that rapidly expand and contract. This destroys tissues mechanically and only where a tissue-specific cavitation threshold is reached [Citation27]. HIFU, in contrast, creates tissue heating and coagulation necrosis through the application of near-continuous, low amplitude ultrasound pulses to tissue [Citation28]. Both heat and radiation are threshold-deterministic effects where tissue damage can still occur within the beam path at sub-therapeutic levels [Citation19]. Cell death by histotripsy, however, depends on the mechanical properties of the treated tissue, where stiffer, collagenous tissues, like large blood vessels and bile ducts, are more resistant than parenchymal organs and cellular tumors [Citation3–5]. Despite bowel wall containing collagen, directly targeting the bowel with histotripsy has been shown to cause perforation in a prior ex vivo study and should be avoided [Citation3].
The ability to successfully treat with histotripsy through acoustic blockage is a complex topic with many variables. In the ideal case, there is no intervening blockage between the transducer and target, and the tissue has a uniform speed of sound. However, this condition is unrealistic in the abdomen and pelvis due to bowel, bone, fat, and non-uniform solid organs. In the presence of blockage, the ability to create a histotripsy treatment at the target is dependent on the proportion of transducer surface area with an unobstructed path to the target. The degree of blockage in this study ranged from 27.3 − 49.6%, which allowed for sufficient energy to converge at the focal point to produce histotripsy. The slight widening of the treatment zone in the presence of blockage was likely due to the increased amplitude required to achieve histotripsy at the focal point. Unobstructed elements directing sound at the periphery of the treatment zone likely created robust cavitation which minimally enlarged the transverse diameter. This is unlikely to be clinically significant but should be a factor in choosing the size of a treatment zone in the event of partial central blockage.
Bowel blockage can be highly variable depending on the amount and proportion of gas and fluid in the bowel at the time of treatment, but this may be able to be manipulated. In a study comparing the feasibility of pancreatic histotripsy in fasted swine versus animals fed simethicone to improve the acoustic window, successful bubble clouds were only observed in the group receiving simethicone [Citation6]. As bowel fills with liquid and becomes more ‘transparent’ to sound, lesser amounts of unobstructed transducer surface area may be able to create safe treatments. If the overall blockage becomes too high, the risk of off-target heating increases which can result in bowel injuries. However, this risk should decrease when transducers with send-receive capability, aberration correction, and automated cavitation detection become available [Citation29–32]. Additionally, these hardware developments will help in scenarios where the bubble cloud is difficult to visualize because of gas or bone blockage. Finally, integrated guidance with cross-sectional imaging, such as cone-beam CT, may improve targeting and allow real-time evaluation of bubble clouds with a modality not subject to blockage by gas [Citation33].
This study had several limitations. Due to the use of an ex vivo model, chronic effects in live tissue and effects on the intervening body wall could not be evaluated. Additionally, the harvested ex vivo tissue lacked blood flow and peristalsis which could serve to decrease heating and the time the bowel was exposed to the ultrasound beam. Treatments were only prescribed deep to the gas blockage with a limited range of blockages, and the effects of treating with gas deep or adjacent to the target or with the treatment zone overlapping the bowel were not included in this preliminary study. The targeting accuracy of treating through gas, including potential translational effects from aberration were also not studied. Lastly, the effects of treating through different amounts of gas, gas/fluid mixtures, and gas/fluid/solid intraluminal contents was not studied.
In summary, this preliminary study suggests that histotripsy performed with gas-filled bowel within the therapeutic beam path is efficacious and has the potential to be safe. Further validation in an in vivo survival model is needed prior to applying the technique in a clinical setting.
Acknowledgments
The content is solely the responsibility of the authors and does not necessarily represent the official views of the NIH. Equipment was provided by Histosonics, Inc.
were created with BioRender.com under license numbers FG26JVR5JQ, TH26JVVWJA, and EE26UQY8JN.
The data that support the findings of this study are available from the corresponding author, TJZ, upon reasonable request.
Conflicts of interest
MAK: none
JKW: none
SP: none
AZK: none
ALK: none
XZ: none
LM: none
PFL: Consultant, research support and shareholder HistoSonics, Inc. Consultant NeuWave Medical/Ethicon/Johnson & Johnson. Shareholder Elucent Medical. Shareholder McGinley Orthopedics. Shareholder RevOps Medical. Research support Siemens Medical.
MGW: consultant: HistoSonics, research support: Siemens Healthineers, Canon
EV: Consultant, research support, and shareholder HistoSonics, Inc.
FTL: Patents, royalties Medtronic, Inc. Consultant, research support Ethicon, Inc. Stockholder, board of directors, research support HistoSonics, Inc. Board of Medical Advisors, Canon, Inc.
TJZ: Research support, shareholder Histosonics; Consultant, research support Ethicon; Consultant, Elephas; Speaker’s bureau, Canon.
Additional information
Funding
References
- Xu Z, Ludomirsky A, Eun LY, et al. Controlled ultrasound tissue erosion. IEEE Trans Ultrason Ferroelectr Freq Control. 2004;51(6):1–10. doi: 10.1109/tuffc.2004.1308731.
- Roberts WW, Hall TL, Ives K, et al. Pulsed cavitational ultrasound: a noninvasive technology for controlled tissue ablation (histotripsy) in the rabbit kidney. J Urol. 2006;175(2):734–738. doi: 10.1016/S0022-5347(05)00141-2.
- Vlaisavljevich E, Kim Y, Owens G, et al. Effects of tissue mechanical properties on susceptibility to histotripsy-induced tissue damage. Phys Med Biol. 2014;59(2):253–270. doi: 10.1088/0031-9155/59/2/253.
- Smolock AR, Cristescu MM, Vlaisavljevich E, et al. Robotically assisted sonic therapy as a noninvasive nonthermal ablation modality: proof of concept in a porcine liver model. Radiology. 2018;287(2):485–493. May doi: 10.1148/radiol.2018171544.
- Longo KC, Knott EA, Watson RF, et al. Robotically assisted sonic therapy (RAST) for noninvasive hepatic ablation in a porcine model: mitigation of body wall damage with a modified pulse sequence. Cardiovasc Intervent Radiol. 2019;42(7):1016–1023. Jul doi: 10.1007/s00270-019-02215-8.
- Gannon J, Imran KM, Hendricks-Wenger A, et al. Ultrasound-guided noninvasive pancreas ablation using histotripsy: feasibility study in an in vivo porcine model. Int J Hyperthermia. 2023;40(1):2247187.
- Imran KM, Gannon J, Morrison HA, et al. Successful in situ targeting of pancreatic tumors in a novel orthotopic porcine model using histotripsy. Ultrasound Med Biol. 2023;49(11):2361–2370. doi: 10.1016/j.ultrasmedbio.2023.07.013.
- Couillard AB, Zlevor AM, Ziemlewicz TJ, et al. A comparison of histotripsy and percutaneous cryoablation in a chronic healthy swine kidney model. J Vasc Interv Radiol. 2023;34(11):1986–1996. Nov doi: 10.1016/j.jvir.2023.07.014.
- Vidal-Jove J, Serres X, Vlaisavljevich E, et al. First-in-man histotripsy of hepatic tumors: the THERESA trial, a feasibility study. Int J Hyperthermia. 2022;39(1):1115–1123. doi: 10.1080/02656736.2022.2112309.
- Schuster TG, Wei JT, Hendlin K, et al. Histotripsy treatment of benign prostatic enlargement using the Vortx Rx system: initial human safety and efficacy outcomes. Urology. 2018;114:184–187. Apr doi: 10.1016/j.urology.2017.12.033.
- Xu Z, Khokhlova TD, Cho CS, et al. Histotripsy: a method for mechanical tissue ablation with ultrasound. Annu Rev Biomed Eng. 2024;26(1). doi: 10.1146/annurev-bioeng-073123-022334.
- Chen J, Chen W, Zhang L, et al. Safety of ultrasound-guided ultrasound ablation for uterine fibroids and adenomyosis: a review of 9988 cases. Ultrason Sonochem. 2015;27:671–676. Nov doi: 10.1016/j.ultsonch.2015.05.031.
- Hwang DW, Song HS, Kim HS, et al. Delayed intestinal perforation and vertebral osteomyelitis after high-intensity focused ultrasound treatment for uterine leiomyoma. Obstet Gynecol Sci. 2017;60(5):490–493. doi: 10.5468/ogs.2017.60.5.490.
- Ko JKY, Seto MTY, Cheung VYT. Thermal bowel injury after ultrasound-guided high-intensity focused ultrasound treatment of uterine adenomyosis. Ultrasound Obstet Gynecol. 2018; Aug52(2):282–283. doi: 10.1002/uog.18965.
- Liu Y, Zhang WW, He M, et al. Adverse effect analysis of high-intensity focused ultrasound in the treatment of benign uterine diseases. Int J Hyperthermia. 2018;35(1):56–61. Dec 31 doi: 10.1080/02656736.2018.1473894.
- Knott EA, Swietlik JF, Longo KC, et al. Robotically-assisted sonic therapy for renal ablation in a live porcine model: initial preclinical results. J Vasc Interv Radiol. 2019;30(8):1293–1302. Aug doi: 10.1016/j.jvir.2019.01.023.
- Maxwell AD, Wang TY, Yuan L, et al. A tissue phantom for visualization and measurement of ultrasound-induced cavitation damage. Ultrasound Med Biol. 2010;36(12):2132–2143. Dec doi: 10.1016/j.ultrasmedbio.2010.08.023.
- Hwang JH, Wang Y-N, Warren C, et al. Preclinical in vivo evaluation of an extracorporeal HIFU device for ablation of pancreatic tumors. Ultrasound Med Biol. 2009;35(6):967–975. Jun doi: 10.1016/j.ultrasmedbio.2008.12.006.
- Liu C-X, Gao X-S, Xiong L-L, et al. A preclinical in vivo investigation of high-intensity focused ultrasound combined with radiotherapy. Ultrasound Med Biol. 2011;37(1):69–77. Jan doi: 10.1016/j.ultrasmedbio.2010.10.001.
- Xie B, Li YY, Jia L, et al. Experimental ablation of the pancreas with high intensity focused ultrasound (HIFU) in a porcine model. Int J Med Sci. 2010;8(1):9–15. Dec 17 doi: 10.7150/ijms.8.9.
- Landry TG, Mallay MG, Brown JA. A simple and cost-effective benchtop setup for assessing ultrasound-induced inertial cavitation probability. IEEE Sens Lett. 2023;7(6):1–4. doi: 10.1109/LSENS.2023.3282076.
- Longo KC, Zlevor AM, Laeseke PF, et al. Histotripsy ablations in a porcine liver model: feasibility of respiratory motion compensation by alteration of the ablation zone prescription shape. Cardiovasc Intervent Radiol. 2020;43(11):1695–1701. Nov doi: 10.1007/s00270-020-02582-7.
- Loge L, Florescu C, Alves A, et al. Radiation enteritis: diagnostic and therapeutic issues. J Visc Surg. 2020;157(6):475–485. Dec doi: 10.1016/j.jviscsurg.2020.08.012.
- Kavanagh BD, Pan CC, Dawson LA, et al. Radiation dose–volume effects in the stomach and small bowel. Int J Radiat Oncol Biol Phys. 2010;76(3 Suppl):S101–S7. Mar doi: 10.1016/j.ijrobp.2009.05.071.
- Murphy JD, Christman-Skieller C, Kim J, et al. A dosimetric model of duodenal toxicity after stereotactic body radiotherapy for pancreatic cancer. Int J Radiat Oncol Biol Phys. 2010;78(5):1420–1426. Dec doi: 10.1016/j.ijrobp.2009.09.075.
- Hauer-Jensen M, Denham JW, Andreyev HJN. Radiation enteropathy—pathogenesis, treatment and prevention. Nat Rev Gastroenterol Hepatol. 2014; Aug11(8):470–479. doi: 10.1038/nrgastro.2014.46.
- Vlaisavljevich E, Lin K-W, Warnez MT, et al. Effects of tissue stiffness, ultrasound frequency, and pressure on histotripsy-induced cavitation bubble behavior. Phys Med Biol. 2015;60(6):2271–2292. doi: 10.1088/0031-9155/60/6/2271.
- Ter Haar >G, Coussios C. High intensity focused ultrasound: physical principles and devices. Int J Hyperthermia. 2007;23(2):89–104. doi: 10.1080/02656730601186138.
- Macoskey JJ, Sukovich JR, Hall TL, et al. Real-time acoustic-based feedback for histotripsy therapy. J Acoust Soc Am. 2017;141(5_Supplement):3551–3551. 1doi: 10.1121/1.4987518.
- Macoskey JJ, Hall TL, Sukovich JR, et al. Soft-tissue aberration correction for histotripsy. IEEE Trans Ultrason Ferroelectr Freq Control. 2018;65(11):2073–2085. Nov doi: 10.1109/TUFFC.2018.2872727.
- Yeats E, Gupta D, Xu Z, et al. Effects of phase aberration on transabdominal focusing for a large aperture, low f-number histotripsy transducer. Phys Med Biol. 2022;67(15):155004. doi: 10.1088/1361-6560/ac7d90.
- Sukovich JR, Macoskey JJ, Lundt JE, et al. Real-time transcranial histotripsy treatment localization and mapping using acoustic cavitation emission feedback. IEEE Trans Ultrason Ferroelectr Freq Control. 2020;67(6):1178–1191. Jun doi: 10.1109/TUFFC.2020.2967586.
- Wagner MG, Periyasamy S, Kutlu AZ, et al. An X-ray C-arm guided automatic targeting system for histotripsy. IEEE Trans Biomed Eng. 2022;70(2):592–602. doi: 10.1109/TBME.2022.3198600.