Abstract
Background
Cryoablation (Cryo) is a minimally invasive treatment for tumors. Cryo can activate the body’s immune response, although it is typically weak. The immune response induced by Cryo in hepatocellular carcinoma (HCC) is poorly understood. PD-1 and CTLA-4 monoclonal antibodies are immune checkpoint inhibitors used in immunotherapy for tumors. The combined use of these antibodies with Cryo may enhance the immune effect.
Methods
A Balb/c mouse model of HCC was established and treated with Cryo, immune checkpoint blockade (ICB), or Cryo + ICB (combination therapy). The growth trend of right untreated tumors and survival time of mice were determined. The expression of apoptosis-related proteins was detected by Western blot (WB) assay. The percentages of immune cells and immunosuppressive cells were analyzed by flow cytometry. The numbers of infiltrating T lymphocytes were checked by immunohistochemistry, and the levels of T-cell-associated cytokines were detected by Quantitative real-time Polymerase Chain Reaction (qRT-PCR) assays and Enzyme-Linked Immunosorbent Assays (ELISA) assays.
Results
Cryo + ICB inhibited the growth of right untreated tumors, promoted tumor cell apoptosis, and prolonged the survival time of mice. Local T-cell infiltration in right tumor tissues increased after the combination therapy, while the number of immunosuppressive cells was significantly reduced. In addition, the combination therapy may induce the production of multiple Th1-type cytokines but reduce the production of Th2-type cytokines.
Conclusions
Cryo can activate CD8+ and CD4+ T-cell immune responses. Cryo + ICB can relieve the immunosuppressive tumor microenvironment and shift the Th1/Th2 balance toward Th1 dominance, further enhancing the Cryo-induced T-cell immune response and resulting in a stronger antitumor immune response.
1. Background
As reported in Global Cancer Statistics 2020 [Citation1], hepatocellular carcinoma (HCC) is the third most frequent cause of cancer death in the world. Although surgery is currently the preferred treatment option for HCC, most patients are diagnosed at the intermediate or advanced stages, where surgical intervention is often not feasible due to the extent of tumor involvement, presence of metastasis, or underlying liver dysfunction [Citation2]. Compared to traditional surgery, cryoablation (Cryo) under ultrasound, Computed Tomography (CT) or Magnetic Resonance Imaging (MRI) guidance has the advantages of decreased invasiveness, fewer complications, precise guidance and rapid recovery, it has been rapidly applied in many hospitals [Citation3, Citation4]. Cryo treatment for malignant tumors induces tissue damage through a process known as freeze–thaw injury. Freezing the tumor tissue causes the formation of ice crystals, resulting in cellular dehydration and mechanical disruption. During the subsequent thawing phase, the ice crystals melt, leading to a rapid temperature change and thermal stress. This freeze–thaw cycle causes cellular destruction, disrupts tumor vasculature, and activates immune responses, ultimately leading to tumor cell death and tissue damage [Citation5]. Previous studies have confirmed that Cryo is a safe and feasible treatment for HCC [Citation6].
Currently, it is believed that Cryo can not only inactivate tumors in situ but also induce the release of tumor-associated antigens and cytokines from necrotic tissues, which can cause dendritic cell activation, activate effector T cells and induce antitumor immune responses [Citation7, Citation8]. In recent years, Zhang et al. demonstrated that Cryo can induce immune responses in lung cancer and renal cell carcinoma through mouse experiments and clinical trials. However, the immune response is weak and insufficient to prevent tumor recurrence [Citation9, Citation10]. Therefore, more combined treatment modalities are needed to improve the therapeutic effect of Cryo. The optimal treatment strategy for HCC should take into account both local tumor and systemic immunity characteristics. On the one hand, local tumors should be precisely targeted to reduce the tumor load, and on the other hand, existing metastases should be destroyed, and potential metastasis should be inhibited.
Cytotoxic T lymphocyte-associated protein-4 (CTLA-4), programmed death receptor-1 and programmed death ligand 1 (PD-L1) are immune checkpoint proteins involved in regulating the immune response against tumors. CTLA-4 inhibits T-cell activation by competing with CD28 for binding to CD80/CD86 on antigen-presenting cells (APCs), while PD-1/PD-L1 transmits inhibitory signals to T cells, leading to their exhaustion and reduced antitumor activity. Blocking CTLA-4 with monoclonal antibodies (mAbs) enhances T-cell activation, decreases regulatory T cells (Tregs)-mediated immune suppression, and promotes an antitumor immune response. Similarly, blocking PD-1/PD-L1 with mAbs reverses T-cell exhaustion, overcomes tumor immune evasion, and enhances the antitumor immune response [Citation11–13]. The combination of PD-1 and CTLA-4 mAbs has synergistic effects, as they act on different checkpoints and stages of the immune response. This combination broadens the immune response, overcomes resistance to PD-1/PD-L1 blockade, and improves therapeutic outcomes. The FDA-approved combination of nivolumab (PD-1 mAb) and ipilimumab (CTLA-4 mAb) has shown promising results in various cancers, making the combined use of PD-1 and CTLA-4 mAbs a promising approach in cancer immunotherapy [Citation14–16].
Ghassan et al. investigated the effectiveness and safety of combining tremelimumab (CTLA-4 mAb) and durvalumab (PD-1 mAb) compared to durvalumab or sorafenib alone in patients with unresectable liver cancer. The combination therapy significantly improved overall survival compared to sorafenib, and durvalumab alone was just as effective as sorafenib. Although there were no significant differences in median progression-free survival among all three groups, more adverse events were observed with sorafenib [Citation17]. HIMALAYA trial showed the effectiveness of dual immune checkpoint blockade (ICB) for liver cancer, forming the basis of the STRIDE regimen (Single Tremelimumab Regular Interval Durvalumab) [Citation17]. These findings suggest that dual ICB therapy may be a promising treatment option for unresectable liver cancer. At present, there are few animal experiments and clinical studies on Cryo combined with immunotherapy as HCC treatment.
Strategies combining Cryo with various immunotherapy modalities have the potential to become novel options for the treatment of tumors, including HCC. However, the mechanism underlying the potential synergistic antitumor effects of Cryo combined with immunotherapy in HCC is still unclear, and further research is needed to demonstrate this synergy. Therefore, we administered Cryo combined with dual ICB to HCC mice and assessed survival, the immune responses of distant tumors and markers of apoptosis. The aim of this study was to investigate the immune effects of combining Cryo with dual ICB in mice HCC model and to explore the potential mechanisms underlying the enhanced antitumor efficacy.
2. Materials and methods
2.1. Cell culture
Mouse HCC cells H22 were obtained from the Chinese Academy of Sciences. The cells used in this experiment were cultured in RPMI 1640 medium supplemented with 10% fetal bovine serum and 1% penicillin–streptomycin and incubated at 37 °C in a 5% CO2 incubator. External services were not involved in our study.
2.2. Animal models and treatment
SPF-grade 4- to 6-week-old male Balb/c mice were purchased from Sinosai Biotechnology Co., Ltd. (Suzhou, Jiangsu, China). The study design is shown in . A total of 1 × 106 cells were injected into the bilateral dorsum of the mice (). On the 12th day after tumor cell implantation, treatment was performed when the tumor volume reached approximately 400 mm3. Forty tumor-bearing mice were randomly divided into four groups using a random number table: Cryo combined with PD-1 and CTLA-4 mAbs (Cryo + P + C) treatment group, the Cryo treatment group, the PD-1 and CTLA-4 mAbs (P + C) treatment group, and the untreated (Control) group. Only the left tumor received complete Cryo. Seven mice from each group were used for right unablated tumor volume measurement and survival observation. On Day 13 after Cryo, three mice were sacrificed for a series of immunological tests of the right unablated tumors.
Figure 1. Flow chart of the study design and scheme of the untreated tumor assessment. A. Forty-five mice received tumor implantation on their bilateral flanks. Two of these mice had failed tumor implantation and tumor volumes of another three mice were >400 mm3 on Day 12 after tumor implantation, a total of five mice were excluded from our study. B. Finally, 40 mice with successful tumor implantation on their bilateral flanks were included in our study for right unablated tumor volume measurements, survival observations and immunological testing. Cryo: cryoablation.
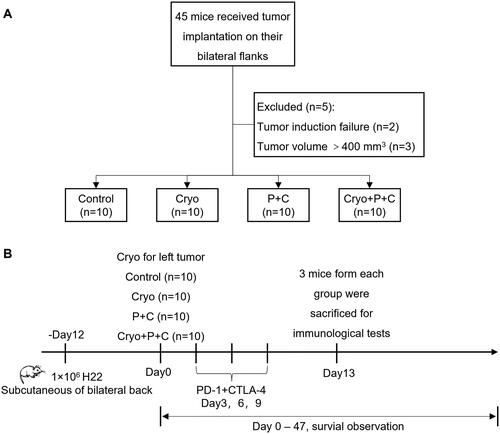
The mice were anesthetized by intraperitoneal injection of 1% sodium pentobarbital, and then they were secured on the operating table. The surgical area was routinely prepared with skin disinfectant and dried. A small incision of approximately 1.5 mm was made on the tumor surface, followed by the insertion of a 2.0 mm diameter cryoprobe (Endocare, USA) into the center part of the tumor. Argon gas was administered, and the temperature at the cryoprobe tip reached a minimum of −40 °C, lasting for at least 30 s, and two freeze–thaw cycles were conducted to ensure the complete ablation of the targeted tumors [Citation18, Citation19]. During the Cryo process, an ice ball was formed that completely covered the subcutaneous tumor. The cryoprobe was withdrawn, and the skin was sutured.
PD-1 and CTLA-4 mAbs (2 mg/kg, 10 mg/kg) were administered intraperitoneally to the P + C and Cryo + P + C groups on the third day after Cryo, and 100 µl of Phosphate Buffered Saline (PBS) was administered intraperitoneally to the Control and Cryo groups. The day Cryo was administered was Day 0, and PD-1 and CTLA-4 mAbs were also administered intraperitoneally on Day 6 and Day 9 after Cryo. The Catalogue numbers of all reagents are listed in Table E1. The Institutional Animal Care and Use Committee approved all animal studies (approval serial number: K2022070).
Table 1. The list of primers.
2.3. Tumor evaluation
The long diameter (A) and short diameter (B) of the tumor on the right side were recorded with Vernier calipers every 2 days until the mice died or the long diameter of the tumor exceeded 2 cm. The following formula was used: Tumor volume (mm3) = (A × B2)/2. One-way analysis of variance (ANOVA) was performed on the last measured tumor volume, and significant differences were noted.
2.4. Western blot (WB)
On the 13th day after Cryo, the mice were sacrificed by cervical dislocation and the right untreated tumor was completely isolated. Approximately 50 mg of tumor tissue was then collected for subsequent WB analysis. The tumor tissues were cut with ophthalmic scissors and placed in a 2 ml homogenizer. Next, 1.5 ml RIPA protein lysis solution was added. The sample was fully homogenized at 4 °C, the supernatant was collected. The concentration of each sample was measured and adjusted accordingly. Subsequently, sequential protein denaturation, electrophoresis, transfer, and blocking were performed. The sample was incubated with primary and secondary antibodies. Finally, Enhanced Chemiluminescence (ECL) chromogenic solution was added under a gel imager.
2.5. Flow cytometry
The right untreated tumor was extracted, immersed in digestion solution and incubated at 37 °C for 1 h. The cell suspension was then filtered through a 40 µm filter to remove debris and cell clumps. The cells were washed with PBS and resuspended. The cell concentration was adjusted to 1 × 107/ml in l00 µl for examination. The corresponding surface antibodies (1:200) were added and incubated on ice in the dark for 20 min for surface antibody staining. After surface antibody staining was completed, 60 µl of cytokine fixation solution was added and fixed on ice in the dark for 20 min. The cells were washed twice with cytokine permeabilization buffer, and cytokine staining antibodies (1:200) were prepared with cytokine permeabilization buffer. The cells were incubated on ice in the dark for 25 min for cytokine staining. Anti-mouse FITC CD45 Antibody, PerCP/Cyanine5.5 CD4 Antibody, PE/Cyanine7 CD8a Antibody, PE FOXP3 Antibody, FITC Ly-6G/Ly-6C (Gr-1) Antibody, APC CD11b Antibody (Biolegend, USA) were used. Detection was performed on a Beckman Coulter (USA) flow cytometer, and the percentages of immune cells and immunosuppressive cells in right tumor tissues were analyzed by CytExpert software.
2.6. qRT-PCR
Total RNA was extracted from approximately 50 mg of right untreated tumor tissue using TRIzol. The sample volume needed to obtain 5 µg of RNA was calculated based on the concentration of RNA, 1 µl of reverse transcription primer was added, and RNase-free water was added up to 12 µl. The sample was mixed thoroughly, denatured thermally at 65 °C for 10 min, and then immediately removed and placed on ice. cDNA was synthesized using a cDNA synthesis kit. The primer sequences are listed in .
2.7. Immunohistochemistry staining
The right untreated tumor tissue was removed on Day 13 after Cryo, fixed in 4% formalin and processed into paraffin sections. The sections were dewaxed by the conventional method, placed in antigen repair buffer and heated in a microwave oven at medium–low heat for a suitable time. Goat serum was added and incubated at room temperature. Then, 50 µl of primary antibody (CD4, CD8, CD31) diluted to the appropriate ratio was added and incubated for 2 h. Then, 50 µl of horseradish peroxide-labeled sheep anti-mouse IgG antibody was added and incubated for 1 h. Two drops of diaminobenzidine reagent were added. Finally, all sections were immersed in hematoxylin staining solution for 10 min.
2.8. ELISA
Sufficient blood samples were collected on Day 13 after completing the combination therapy by removing the eyeballs of mice. Serum specimens were obtained through high-speed centrifugation. All reagents and mouse serum were first brought to room temperature. Then, serum concentrations of Interferon-gamma (IFN-γ) and Interleukin-4 (IL-4) (Abam, USA) were determined according to the reagent instructions. All measurements were conducted in triplicate to ensure accuracy and reduce repetition.
2.9. Statistical analysis
GraphPad Prism 8 was used to graph and statistically analyze the experimental results. All data from this experiment were obtained from at least three independent experiments, and the data are presented as the mean ± SD. The log-rank test was used to compare survival results of Kaplan–Meyer curves, and one-way ANOVA was used to compare more than two groups of samples. A p value of <.05 was used as the threshold for statistical significance.
3. Results
3.1. Distribution of PD-1 and CTLA-4 gene expression in human HCC tumor tissues and corresponding normal paracancerous tissues
Due to the complex immune microenvironment of HCC, the efficacy of individual immune drug therapy is limited. Immune combination therapy can act on multiple targets simultaneously to enhance the antitumor effect. PD-l and CTLA-4 are classical immune checkpoints that act as inhibitors in physiological situations to regulate the duration and intensity of the immune response. However, they are involved in assisting tumor immune escape in the tumor microenvironment. We obtained RNAseq data from tumor tissues of 371 primary HCC patients and 113 paired paraneoplastic normal tissues from the TCGA database (). The results showed that the expression of the PDCD1 gene and CTLA-4 gene was significantly higher in tumor tissues of HCC patients. These results provide a theoretical basis for the treatment of HCC patients with dual ICB.
3.2. Cryo with dual ICB combination therapy produced synergistic antitumor effects
To determine whether Cryo + ICB could produce synergistic antitumor effects, we grew H22 HCC cells bilaterally in the backs of mice, and after tumor formation (), the mice were randomly divided into different treatment groups. The left tumor in the Control group showed rapid growth, while the P + C group significantly reduced the growth rate of the left tumor. Additionally, Cryo completely eliminated the left tumor. The growth curves of right tumors showed that the tumor volume in the Cryo + P + C group was significantly smaller than those in the other groups on Day 20 after Cryo (). Mice in the Cryo + P + C group had the lightest tumor weight on Day 20 after Cryo, indicating that the combined treatment had the most significant effect on reducing tumor load (). These results showed that Cryo and P + C treatment alone had a moderate inhibitory effect on tumor growth, but importantly, this effect was more pronounced when Cryo was combined with P + C.
Figure 3. Cryo with PD-1/CTLA-4 monoclonal antibody combination therapy produces synergistic antitumor effects. A. Cryo of the left tumor and preservation of the right tumor. B. H&E staining of the tumor tissue. Bars = 50 µm. C. Growth of the tumors on the untreated side during the observation period. D. Tumor specimens of the tumors on the untreated side in each group on Day 20 after Cryo. Five representative tumor specimens were taken from the untreated side of each group. E. Weight of the tumors on the untreated side in each group on Day 20 after Cryo. F. Survival time curves of each group of tumor-bearing mice. G. Body weights of each group of tumor-bearing mice. N = 7.
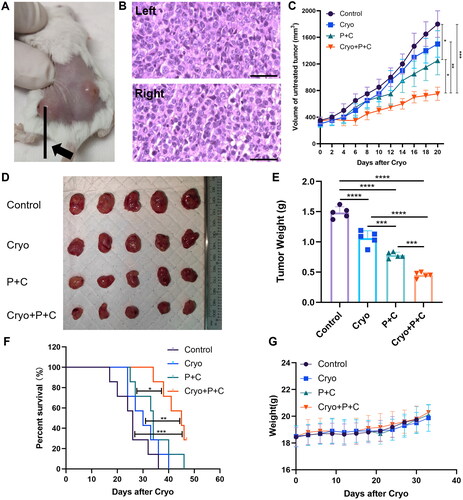
Cryo treatment was administered on Day 0. The survival period of the four groups of mice was recorded (). By the end of the observation period, all the mice in the Control, Cryo and P + C groups had died, and two mice in the Cryo + P + C group survived the whole observation period. The median survival times of mice in the Control, Cryo, P + C and Cryo + P + C groups were 26, 30, 34 and 45 days, respectively. The survival time of mice in the Cryo + P + C group was significantly longer than that in the Control, Cryo and P + C groups. The body weights of the mice in the four groups were not significantly different throughout the observation period (), suggesting that Cryo combined with P + C had no significant toxic side effects.
We also found that the combination treatment significantly increased the expression of Bax and cleaved Caspase 3 (C-caspase-3) and decreased the expression of Bcl-2 in distant tumor tissues of H22 HCC-bearing mice by WB assay (), suggesting that the combination treatment promotes apoptosis of tumor cells via regulation of the Caspase-3/Bax/Bcl-2 apoptotic signaling pathway. The results of these animal experiments confirmed that Cryo and P + C combination treatment significantly reduced the growth rate of distant tumors and increased the survival rate of HCC mice, showing significant synergistic antitumor effects.
Figure 4. Analysis of apoptosis-related protein expression in right tumor tissues and immune-related cytokine levels in serum. A. The protein expression of Bax, Bcl-2 and C-caspase-3 in the right tumor tissues of each treatment group. B. C-caspase-3, Bax and Bcl-2 protein expression levels were calculated with reference to the grayscale values of Glyceraldehyde-3-Phosphate Dehydrogenase (GAPDH) in protein strips. C–D. The expression levels of serum IFN-γ and IL-4 in each treatment group. N = 3.
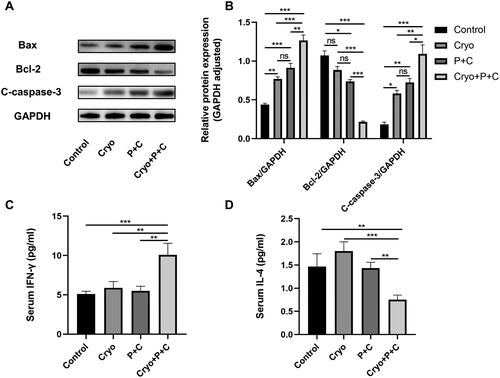
3.3. Cryo combined with dual ICB promoted the secretion of IFN-γ and decreased the secretion of IL-4
Assessment of the Th1-type cytokine IFN-γ by ELISA of mouse peripheral blood showed that the highest IFN-γ concentrations were found in the Cryo + P + C groups (). A comparison of IFN-γ concentrations among the Control, Cryo, and P + C groups showed no significant differences. Notably, Cryo alone increased the level of IL-4, whereas P + C combined with Cryo decreased the levels of IL-4 (). The results suggest that Cryo combined with P + C significantly promoted the secretion of IFN-γ and decreased the secretion of IL-4.
3.4. Cryo and dual ICB combination therapy synergistically improved the immune microenvironment in distant tumors
To investigate the effect of Cryo combined with dual ICB on immune cells and immunosuppressive cells, we extracted the right tumors of mice in the Control, Cryo, P + C and Cryo + P + C groups on Day 13 after the Cryo treatment and assessed them with immunoassays. The results showed that the percentages of CD8+ and CD4+ T cells in the Cryo + P + C group were significantly increased compared to those in the Con, Cryo, P + C groups (). Myeloid-derived suppressor cells (MDSCs) and Tregs play a critical immunosuppressive role in the tumor immune microenvironment, and we found that the percentages of Tregs and MDSCs were higher in the Cryo alone treatment group, while the Treg and MDSC percentages were significantly lower in the Cryo + P + C group (). Our experimental results suggest that Cryo combined with P + C can enhance T-cell immune responses in distant tumor tissues while decreasing the aggregation of immunosuppressive cells.
Figure 5. Cryo and PD-1/CTLA-4 monoclonal antibody treatment (Cryo + P + C) synergistically improves the immune microenvironment of right tumors. A. Representative flow cytograms showing CD4+ cells, CD8+ cells, Tregs, and MDSCs in single-cell suspensions. B–E. Percentages of CD4+ cells, CD8+ cells, Tregs, and MDSCs in single-cell suspensions. N = 3.
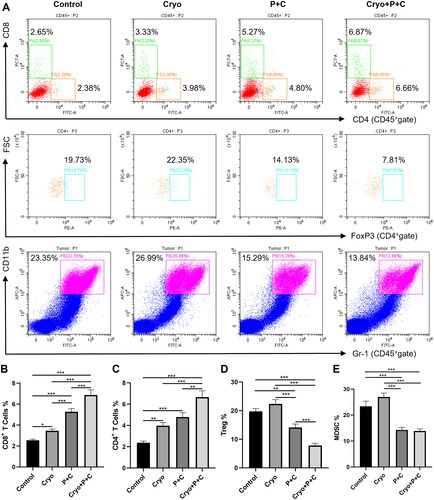
3.5. Cryo and dual ICB combination therapy enhanced T-lymphocyte infiltration into distant tumor tissue
Compared with those in the Control, Cryo, P + C groups, the numbers of CD4+ and CD8+ T cells in the Cryo + P + C group were significantly increased (). CD31 is a marker of microvascular endothelial cells. The tumor microvascular density of the Cryo + P + C group was significantly lower than that of the Control, Cryo, and P + C groups (). These results indicate that Cryo and P + C combination therapy can enhance T lymphocyte infiltration into distant tumor tissue. In addition, the combination therapy can inhibit angiogenesis in distant tumor tissue.
Figure 6. Cryo And PD-1/CTLA-4 monoclonal antibody combination therapy (Cryo + P + C) promotes intratumoral infiltration of T lymphocytes. A. Representative micrographs of CD4+ cells, CD8+ cells, and CD31+ cells in the control, Cryo, P + C and Cryo + P + C groups at 200× magnification. The cellular nuclei exhibited a blue coloration when observed under the microscope, whereas the outcome of the positive test was characterized by a brown hue. Bars = 50 µm. B–D. From each slide, 10 fields were selected randomly for analysis. N = 3.
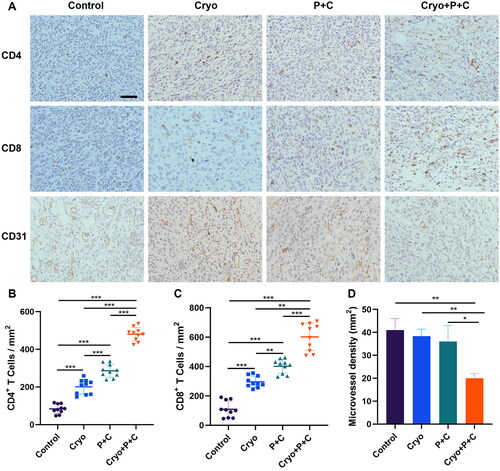
3.6. Cryo combined with dual ICB increased Th1-type cytokines but reduced Th2-type cytokines
To assess the levels of cytokines associated with Th1 and Th2 cells, we examined the relative mRNA expression levels of various cytokines by qRT-PCR. The expression levels of the Th1-type cytokines IFN-γ and IL-12A were higher in the Cryo + P + C group than in the Cryo group, P + C group, or untreated group (). In addition, the Th1 cell transcription factor Eomes showed a similar expression pattern (). It is worth noting that Cryo alone increased the expression level of the Th2 cytokine IL-4 and the Th2 transcription factor GATA3, but Cryo + P + C reduced the levels of these two cytokines (). Another Th2-type cytokine, IL-10, did not exhibit significantly different relative mRNA expression levels between the groups (). Furthermore, we discovered that the outcomes of the analysis of the relative levels of IFN-γ and IL-4 mRNA expression in tumor tissues matched those of the serum ELISA. The above results show that Cryo combined with P + C can promote Th1 cytokine expression and reduce Th2 cytokine expression.
Figure 7. A–F. Determination of the relative mRNA expression levels of IFN-γ, IL-12A, Eomes, IL-4, GATA3, and IL-10 in right tumor tissues of mice in the control, Cryo, P + C and Cryo + P + C groups. The data obtained from qRT-PCR were evaluated using the 2−ΔΔCT method, which involves normalization of the control group values to a relative expression of 1. N = 3.
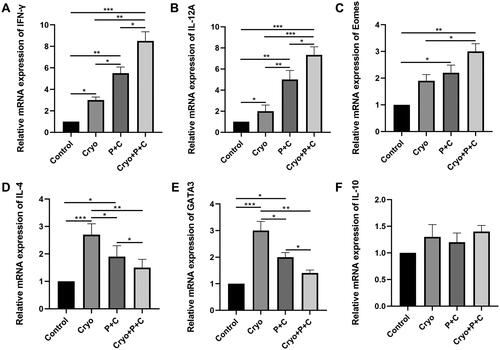
4. Discussion
Surgery is the recommended method of treating HCC, but a huge proportion of patients are not surgical candidates at the time of diagnosis, and surgery can seriously compromise the body’s immune defense mechanism [Citation20, Citation21]. The ‘abscopal effect’ caused by Cryo, which is considered to be the foundation of the synergistic antitumor benefits seen with immunotherapy, can cause the regression of primary tumors and distant metastases [Citation22, Citation23]. In this study, we discovered that Cryo induced a T-cell immune response in mice with HCC, but the median survival of mice treated with Cryo alone was only 4 days longer than that of untreated mice. PD-1 and CTLA-4 mAbs injection after Cryo can delay the growth of distant tumors, promote apoptosis of tumor cells and prolong the survival time of mice. At the same time, local T-cell infiltration was increased in distant tumors after the combination therapy.
MDSCs and Tregs are two major immunosuppressive cell types in tumor patients [Citation24, Citation25], and we found that these two cell types were significantly decreased in distant tumor tissue in the Cryo + P + C group. This result suggests that the combination therapy can reduce the percentage of immunosuppressive cells in distant tumor tissues while reducing tumor load. In addition, we validated the antiangiogenic effects of Cryo combined with P + C. Caspase-3/Bax/Bcl-2 is an important apoptosis signaling pathways, and caspase activation is a key factor initiating apoptosis [Citation26]. We found that the combination treatment significantly increased the expression of Bax and C-caspase-3 and decreased the expression of Bcl-2 in distant tumor tissues of HCC mice by WB, suggesting that the combination treatment promotes apoptosis of tumor cells via regulation of the Caspase-3/Bax/Bcl-2 apoptotic signaling pathway.
Several preliminary studies of the combination of Cryo with immunotherapy have been conducted. Liu et al. retrospectively evaluated the efficacy of Cryo combined with immunotherapy in patients with metastatic pancreatic cancer and found that the median survival time of patients was significantly longer than that of those receiving Cryo alone or chemotherapy after 4 years of follow-up [Citation27]. Cryo combined with CTLA4 mAb enhanced the immune functions of the body, improved the effect of prostate cancer treatment, and effectively prevented the metastasis and recurrence of prostate cancer in a mouse model [Citation18]. Mandt et al. conducted experiments in orthotopic liver HCC xenograft model mice and treated the tumors with incomplete Cryo alone or with intratumoral the immunostimulant CpG and/or PD-1 inhibitor [Citation16]. Their study demonstrated Cryo combined with immunostimulant therapy has potential in promoting antitumoral immunity and slowing tumor growth in HCC. Notably, Shi et al. found that incomplete radiofrequency ablation (RFA) of metastatic colorectal cancer limited the efficacy of PD-1 mAb immunotherapy. The authors demonstrated that incomplete ablation induced local inflammation and led to the accumulation of immunosuppressive myeloid cells in the residual tumor, which suppressed T-cell function [Citation28]. These results suggest that Cryo or incomplete Cryo in combination with various immunotherapies for the treatment of tumors needs to be further validated by more studies in the future.
In the treatment of HCC, clinical doctors prefer to use RFA and microwave ablation (MWA) as the preferred treatment methods rather than Cryo. RFA and MWA can generate high temperatures in a shorter period of time, causing thermal necrosis of tumor tissue. In contrast, Cryo requires a longer time to reach a sufficiently low temperature. RFA and MWA are easier to operate and control compared to Cryo. They require relatively simple equipment and techniques, allowing treatment to be completed in a shorter period of time [Citation6]. Although RFA and MWA are the preferred treatment methods for clinical doctors, the specific treatment choice still depends on the condition of the HCC patients, the location and size of the tumor, and the experience and professional opinion of the doctor. In terms of immune modulation effects, the main difference between Cryo and high-temperature-based ablation techniques lies in the nature of tissue injury they induce. Cryo causes cell death by freezing and subsequent thawing, leading to the release of pro-inflammatory mediators and damage-associated molecular patterns (DAMPs). This can trigger an immune response characterized by an increase in pro-inflammatory cytokines and chemokines. In contrast, high-temperature-based ablation techniques like RFA/MWA primarily cause thermal coagulative necrosis, which may induce a milder immune response compared to Cryo [Citation13].
The relative mRNA expression levels of IFN-γ and IL-12A were increased in the right tumor tissues of mice in the Cryo + P + C group, and the expression pattern of the Th1-type transcription factor Eomes was similar. Eomes is an important transcription factor regulating the differentiation and function of type I effector T cells such as CD4+ Th1 and CD8+ cytotoxic T cells, and plays an important regulatory role in CD8+ T-cell-mediated antitumor immune response [Citation29]. GATA3, on the other hand, mainly mediates the differentiation of Th2-type T cells [Citation30]. Cryo can stimulate the body’s immune response, but it also induces the development of immunosuppression, increases the number of Tregs and MDSCs and upregulates the expression of Th2-type cytokines [Citation4, Citation8]. In our study, Cryo alone increased the levels of IL-4 and the Th2-type transcription factor GATA3, while Cryo combined with P + C decreased the levels of IL-4 and GATA3. In addition, we found that the peripheral blood IFN-γ concentration in mice in the Cryo + P + C group was also significantly increased. IFN-γ is a pleiotropic cytokine mainly secreted by activated T lymphocytes and Natural killer (NK) cells with powerful antitumor functions [Citation31, Citation32]. IFN-γ was significantly elevated in the Cryo + P + C group, indicating that the mice receiving the combined therapy had a powerful antitumor immunity. Shi et al.’s research revealed that the combination treatment of RFA and PD-1 mAb resulted in a significant upregulation of IFN-γ mRNA expression levels in the unablated side tumor compared to the individual treatment groups. Moreover, the combination therapy group demonstrated a notable increase in the population of CD8+ T cells capable of secreting IFN-γ compared to individual treatment groups [Citation33]. IL-4 is a representative Th2-type cytokine [Citation34]. The level of IL-4 expression in mouse serum was consistent with the results of qRT-PCR analysis of tumor tissues. This evidence suggests that Cryo combined with dual ICB improves the tumor immunosuppressive microenvironment and increases the expression of multiple Th1-type cytokines. The induced shift in the Th1/Th2 balance toward Th1 dominance may be an important reason why the combination therapy produced a stronger T-cell immune response.
In addition, as observed in this study, there is a significant increase in pro-inflammatory mediators after Cryo of HCC, which may induce systemic inflammatory response syndrome, leading to a phenomenon known as cryoshock. Cryoshock can have serious consequences, including disseminated intravascular coagulopathy, multi-system organ failure, or even death [Citation35]. In order to mitigate these risks and improve the safety of Cryo in the clinical setting, several strategies can be adopted. These include careful patient selection, optimization of Cryo parameters, real-time monitoring of tissue temperature, and close postoperative monitoring for signs of cryoshock or other complications. Additionally, advancements in Cryo techniques and technology contribute to minimizing the occurrence of cryoshock and improving patient prognosis.
The present study still has some shortcomings, and thus, the results need to be verified by further studies. Firstly, the dosing regimen used in our study is based on previous preclinical research experience. However, we should also consider the toxicity and immune-related adverse events associated with dual ICB therapy, as they are important considerations in clinical use. In future studies, we should design experiments to explore the appropriate dosage and administration schedule for dual ICB, in order to minimize adverse events while maintaining therapeutic efficacy. Secondly, The antitumor effects of T cells need to be further investigated, for example, by performing T-cell depletion experiments. Finally, the immune systems of different species can vary greatly, so the immune effects of Cryo combined with dual ICB need further validation in other animals and humans.
5. Conclusions
In summary, this study confirmed that Cryo treatment can promote T-cell immunity in HCC, and the combined application of dual ICB can improve the immunosuppressive tumor microenvironment and increase the expression of multiple Th1-type cytokines, further enhancing Cryo-induced T-cell immune responses. Both Cryo and PD-1/CTLA-4 mAbs are well-established therapeutic modalities already used in the clinic, so their combination is expected to be an effective strategy for immunotherapy in HCC.
Supplemental Material
Download ()Disclosure statement
No potential conflict of interest was reported by the author(s).
Additional information
Funding
References
- Sung H, Ferlay J, Siegel RL, et al. Global cancer statistics 2020: GLOBOCAN estimates of incidence and mortality worldwide for 36 cancers in 185 countries. CA Cancer J Clin. 2021;71(3):1–12. doi: 10.3322/caac.21660.
- Anwanwan D, Singh SK, Singh S, et al. Challenges in liver cancer and possible treatment approaches. Biochim Biophys Acta Rev Cancer. 2020;1873(1):188314. doi: 10.1016/j.bbcan.2019.188314.
- Yakkala C, Chiang CL, Kandalaft L, et al. Cryoablation and immunotherapy: an enthralling synergy to confront the tumors. Front Immunol. 2019;10:2283. doi: 10.3389/fimmu.2019.02283.
- Chen J, Qian W, Mu F, et al. The future of cryoablation: an abscopal effect. Cryobiology. 2020;97:1–4. doi: 10.1016/j.cryobiol.2020.02.010.
- Chen Z, Meng L, Zhang J, et al. Progress in the cryoablation and cryoimmunotherapy for tumor. Front Immunol. 2023;14:1094009. doi: 10.3389/fimmu.2023.1094009.
- Wang K, Wang C, Jiang H, et al. Combination of ablation and immunotherapy for hepatocellular carcinoma: where we are and where to go. Front Immunol. 2021;12:792781. doi: 10.3389/fimmu.2021.792781.
- Yakkala C, Dagher J, Sempoux C, et al. Rate of freeze impacts the survival and immune responses post cryoablation of melanoma. Front Immunol. 2021;12:695150. doi: 10.3389/fimmu.2021.695150.
- Wu Y, Cao F, Zhou D, et al. Cryoablation reshapes the immune microenvironment in the distal tumor and enhances the anti-tumor immunity. Front Immunol. 2022;13:930461. doi: 10.3389/fimmu.2022.930461.
- Zhang M, Yin T, Lu Y, et al. The application of cytidyl guanosyl oligodeoxynucleotide can affect the antitumor immune response induced by a combined protocol of cryoablation and dendritic cells in Lewis lung cancer model. Med Sci Monit. 2016;22:1309–1317. doi: 10.12659/msm.898194.
- Kato T, Iwasaki T, Uemura M, et al. Characterization of the cryoablation-induced immune response in kidney cancer patients. Oncoimmunology. 2017;6(7):e1326441. doi: 10.1080/2162402X.2017.1326441.
- Jiang Y, Chen M, Nie H, et al. PD-1 and PD-L1 in cancer immunotherapy: clinical implications and future considerations. Hum Vaccin Immunother. 2019;15(5):1111–1122. doi: 10.1080/21645515.2019.1571892.
- Hosseini A, Gharibi T, Marofi F, et al. CTLA-4: from mechanism to autoimmune therapy. Int Immunopharmacol. 2020;80:106221. doi: 10.1016/j.intimp.2020.106221.
- Jiang M, Fiering S, Shao Q. Combining energy-based focal ablation and immune checkpoint inhibitors: preclinical research and clinical trials. Front Oncol. 2023;13:1153066. doi: 10.3389/fonc.2023.1153066.
- Wagner MJ, Othus M, Patel SP, et al. Multicenter phase II trial (SWOG S1609, cohort 51) of ipilimumab and nivolumab in metastatic or unresectable angiosarcoma: a substudy of dual anti-CTLA-4 and anti-PD-1 blockade in rare tumors (DART). J Immunother Cancer. 2021;9(8):e002990. doi: 10.1136/jitc-2021-002990.
- Willsmore ZN, Coumbe BGT, Crescioli S, et al. Combined anti-PD-1 and anti-CTLA-4 checkpoint blockade: treatment of melanoma and immune mechanisms of action. Eur J Immunol. 2021;51(3):544–556. doi: 10.1002/eji.202048747.
- Mandt T, Bangar A, Sauceda C, et al. Stimulating antitumoral immunity by percutaneous cryoablation and combination immunoadjuvant therapy in a murine model of hepatocellular carcinoma. J Vasc Interv Radiol. 2023;34(9):1516–1527.e6.
- Abou-Alfa GK, Lau G, Kudo M, et al. Tremelimumab plus durvalumab in unresectable hepatocellular carcinoma. NEJM Evid. 2022;1(8):EVIDoa2100070. doi: 10.1056/EVIDoa2100070.
- Benzon B, Glavaris SA, Simons BW, et al. Combining immune check-point blockade and cryoablation in an immunocompetent hormone sensitive murine model of prostate cancer. Prostate Cancer Prostatic Dis. 2018;21(1):126–136. doi: 10.1038/s41391-018-0035-z.
- Zhu C, Lin S, Liang J, et al. PD-1 blockade enhances the anti-tumor immune response induced by cryoablation in a murine model of renal cell carcinoma. Cryobiology. 2019;87:86–90. doi: 10.1016/j.cryobiol.2019.01.015.
- Yang JD, Hainaut P, Gores GJ, et al. A global view of hepatocellular carcinoma: trends, risk, prevention and management. Nat Rev Gastroenterol Hepatol. 2019;16(10):589–604. doi: 10.1038/s41575-019-0186-y.
- Hack SP, Spahn J, Chen M, et al. IMbrave 050: a Phase III trial of atezolizumab plus bevacizumab in high-risk hepatocellular carcinoma after curative resection or ablation. Future Oncol. 2020;16(15):975–989. doi: 10.2217/fon-2020-0162.
- Olagunju A, Forsman T, Ward RC. An update on the use of cryoablation and immunotherapy for breast cancer. Front Immunol. 2022;13:1026475. doi: 10.3389/fimmu.2022.1026475.
- Tian Y, Qi X, Jiang X, et al. Cryoablation and immune synergistic effect for lung cancer: a review. Front Immunol. 2022;13:950921. doi: 10.3389/fimmu.2022.950921.
- Rahma OE, Hodi FS. The intersection between tumor angiogenesis and immune suppression. Clin Cancer Res. 2019;25(18):5449–5457. doi: 10.1158/1078-0432.CCR-18-1543.
- Li N, Kang Y, Wang L, et al. ALKBH5 regulates anti-PD-1 therapy response by modulating lactate and suppressive immune cell accumulation in tumor microenvironment. Proc Natl Acad Sci USA. 2020;117(33):20159–20170. doi: 10.1073/pnas.1918986117.
- Jin S-J, Yang Y, Ma L, et al. In vivo and in vitro induction of the apoptotic effects of oxysophoridine on colorectal cancer cells via the Bcl-2/Bax/caspase-3 signaling pathway. Oncol Lett. 2017;14(6):8000–8006. doi: 10.3892/ol.2017.7227.
- Niu L, Chen J, He L, et al. Combination treatment with comprehensive cryoablation and immunotherapy in metastatic pancreatic cancer. Pancreas. 2013;42(7):1143–1149. doi: 10.1097/MPA.0b013e3182965dde.
- Shi L, Wang J, Ding N, et al. Inflammation induced by incomplete radiofrequency ablation accelerates tumor progression and hinders PD-1 immunotherapy. Nat Commun. 2019;10(1):5421. doi: 10.1038/s41467-019-13204-3.
- Zhu Y, Ju S, Chen E, et al. T-bet and eomesodermin are required for T cell-mediated antitumor immune responses. J Immunol. 2010;185(6):3174–3183. doi: 10.4049/jimmunol.1000749.
- Yu F, Sharma S, Edwards J, et al. Dynamic expression of transcription factors T-bet and GATA-3 by regulatory T cells maintains immunotolerance. Nat Immunol. 2015;16(2):197–206. doi: 10.1038/ni.3053.
- Zheng M, Sun R, Wei H, et al. NK cells help induce anti-hepatitis B virus CD8+ T cell immunity in mice. J Immunol. 2016;196(10):4122–4131. doi: 10.4049/jimmunol.1500846.
- Ahmetlic F, Fauser J, Riedel T, et al. Therapy of lymphoma by immune checkpoint inhibitors: the role of T cells, NK cells and cytokine-induced tumor senescence. J Immunother Cancer. 2021;9(1):e001660. doi: 10.1136/jitc-2020-001660.
- Shi L, Chen L, Wu C, et al. PD-1 blockade boosts radiofrequency ablation-elicited adaptive immune responses against tumor. Clin Cancer Res. 2016;22(5):1173–1184. doi: 10.1158/1078-0432.CCR-15-1352.
- Kan X, Zhou G, Zhang F, et al. Enhanced efficacy of direct immunochemotherapy for hepatic cancer with image-guided intratumoral radiofrequency hyperthermia. J Immunother Cancer. 2022;10(11):e005619. doi: 10.1136/jitc-2022-005619.
- Ní Eochagáin A. Cryoshock following cryoablation for hepatocellular carcinoma. J Clin Anesth. 2022;77:110641.