Abstract
Background: Central nervous system (CNS) injury is the most prominent feature of heatstroke and the hippocampus is prone to damage. However, the mechanisms underlying the heatstroke-induced hippocampal injury remain unclear. Hyperbaric oxygen (HBO) therapy prevents CNS injury in heatstroke mice. However, the underlying mechanisms of HBO in heatstroke-induced hippocampal injury remain unclear. This study aimed to elucidate the protective effects of HBO against hippocampal injury and its potential role in microglial pyroptosis in heatstroke rats.Methods: A rat heatstroke model and a heat stress model with a mouse microglial cell line (BV2) were, respectively, used to illustrate the effect of HBO on heat-induced microglial pyroptosis in vivo and in vitro. We used a combination of molecular and histological methods to assess microglial pyroptosis and neuroinflammation both in vivo and in vitro.Results: The results revealed that HBO improved heatstroke-induced survival outcomes, hippocampal injury, and neurological dysfunction in rats. In addition, HBO mitigates microglial pyroptosis and reduces the expression of pro-inflammatory cytokines in the hippocampus of heatstroke rats. In vitro experiments showed that HBO attenuated BV2 cell injury under heat stress. Furthermore, HBO prevented heat-induced pyroptosis of BV2 cells, and the expression of pro-inflammatory cytokines IL-18 and IL-1β was reduced. Mechanistically, HBO alleviates heatstroke-induced neuroinflammation and hippocampal injury by preventing microglial pyroptosis. Conclusions: In conclusion, HBO attenuates heatstroke-induced neuroinflammation and hippocampal injury by inhibiting microglial pyroptosis.
Introduction
Heatstroke, a fatal hyperthermia-associated disease with a core body temperature over 40 °C, recently increased around the world due to global warming [Citation1,Citation2]. This disease can cause systemic inflammatory response syndromes (SIRS) and multiple organ dysfunction syndromes (MODSs), particularly central nervous system (CNS) damage, which is the main cause of death or serious sequelae [Citation3,Citation4]. The hippocampus is sensitive to temperature alterations and hippocampal injury leads to cognitive deficits during the early stages of heatstroke [Citation5,Citation6]. Previous research using magnetic resonance imaging (MRI) in patients with heatstroke suggested that the hippocampus is sensitive to hyperthermia [Citation7]. Cerebral ischemia and hypoxia are involved in heatstroke-induced CNS injury. Additionally, the CNS inflammatory response is also a crucial factor in the occurrence and development of heatstroke-induced CNS injury [Citation8,Citation9]. Inhibition of neuroinflammation rapidly improves the symptoms of heatstroke and prolongs survival time [Citation10,Citation11].
Pyroptosis, a pro-inflammatory form of programmed cell death, is mainly mediated by inflammasomes [Citation12]. Activation of pyroptosis led to cell swelling, rupture and aggravated the inflammatory cascade, including the release of pro-inflammatory cytokines IL-1β and IL-18 [Citation13]. Microglia are major innate immune cells of the CNS and play a prominent role in neuroinflammation and CNS injury. Microglia are also considered the main cells in the CNS where pyroptosis occurs [Citation14,Citation15]. Microglia pyroptosis has been repeatedly implicated in the pathogenesis of multiple CNS diseases including spinal cord injury [Citation16], traumatic brain injury [Citation17], and vascular cognitive impairment [Citation18]. Recent studies have found that microglial cells were involved in heatstroke-induced hippocampal neuronal apoptosis, which was mostly focused on the phenotypic conversion from an anti-inflammatory to a pro-inflammatory phenotype [Citation6,Citation10]. However, the role of microglial pyroptosis in heatstroke-induced hippocampal injury remains unclear.
Hyperbaric oxygen (HBO) refers to the administration of pure oxygen under a pressure greater than 100 kPa (1 atmosphere absolute), which can significantly increase the amount of oxygen dissolved in the plasma and is a noninvasive medical strategy [Citation19,Citation20]. HBO exerts neuroprotective effects against ischemia/hypoxia injury [Citation21], trauma [Citation22], and heatstroke [Citation23] by inhibiting oxidative injury, apoptosis, inflammation, and autophagy [Citation23,Citation24]. Microglia play a key role in triggering cellular responses to HBO [Citation25]. A recent study has found that HBO can attenuate neuroinflammation by regulating microglial polarization during intracerebral hemorrhage in rats [Citation26]. Considering the anti-inflammatory and anti-apoptotic roles of HBO in cerebral injury, we hypothesized that HBO protects the hippocampus from heatstroke injury by inhibiting microglial pyroptosis.
Materials and methods
Animals and materials
Male Sprague–Dawley rats weighing 200–240 g were purchased from Beijing Vital River Laboratory Animal Technology Co. Ltd. (No. 4400210001101). The experimental procedures were approved by the Animal Care and Use Committee of the Wenzhou Medical University (approval number: WYDW2022-0658). The rats were housed under a controlled 12 h/12 h light–dark cycle at 26 °C and (50 ± 5)% humidity. The rats had ad libitum access to food and water. The care and handling of laboratory animals were conducted according to standard guidelines and regulations for the use and care of experimental animals in China.
Cell culture
The mouse microglial cell line (BV2) was provided by the Laboratory of Meisen Biotechnology Company (Zhejiang, China). BV2 cells were cultured in dulbecco’s modified eagle medium (DMEM) (11965092, Gibco, California, USA) containing 10% fetal bovine serum (FBS) (10099141, Gibco, California, USA) and 1% penicillin/streptomycin (15140122, Gibco, California, USA) at a density of 2 × 105/mL in a humidified incubator (3111, Thermo Fisher Scientific, Massachusetts, USA) with 5% CO2 at 37 °C.
Heat protocol for animals and cells
For in vivo experiments, a rat heatstroke model was established according to a previous study [Citation23]. Briefly, the rats in the Heat group were administered an intraperitoneal injection (i.p.) of 30 mg/kg pentobarbital sodium. The anesthetized rats were placed in a pre-warmed intelligent artificial climate chamber (RXZ-280A, Ningbo Jiangnan Instrument Factory, China) that was maintained at 42 ± 0.5 °C and a relative humidity of (55.0 ± 5.0)% without food and water. The rectal temperature (Tc) was monitored every 30 min and subsequently monitored every 10 min after it reached 41.0 °C. The time at which the Tc reached 42 °C was arbitrarily taken as the time of heatstroke onset. All rats were moved to a box at (25.0 ± 0.5)°C and a humidity of (35.0 ± 5.0)% for 10 min after heatstroke. Rats in the Heat + HBO group were immediately placed in an animal HBO chamber. The untreated heatstroke rats and the control group rats were kept at an ambient temperature of 24 ± 0.5 °C with a humidity of (35 ± 5)%. For survival time and neurological function assessment, the rats were assigned to the Control, Heat and Heat + HBO groups (n = 10 in each group). The survival time of rats was assessed when the Tc reached 42 °C. No rats died within 3 h after the heatstroke, 10 surviving rats in each group underwent the behavioral study for 3 h after the heatstroke. For biochemical analysis, the rats in each group (n = 12 in each group) were euthanized 3 h after the heatstroke using 30 mg/kg pentobarbital sodium (i.p.). Hippocampi were collected rapidly after cardio-reperfusion with 300 mL pre-cooled saline (4° C). Among these 12 rats, six rats were quickly dissected and frozen in liquid nitrogen for western blot analysis, and the other six rats were intracardially perfused with 4% paraformaldehyde in phosphate-buffered saline (PBS) at 4 °C for histopathological analysis and immunohistochemical staining.
In in vitro experiments, we randomized the cell cultures into the Control, Heat and Heat + HBO groups (n = 3, each with three parallel wells), the BV2 cells were placed in an incubator (3111, Thermo Fisher Scientific, Massachusetts, USA) at 40 °C for 1 h to prepare the heat-stressed cellular model. After heating, cells were treated with or without HBO, and then immediately returned to a normal incubator at 37 °C with 5% CO2. The control group cells were consistently maintained at 37 °C with 5% CO2.
HBO exposure for animals and cells
In the in vivo experiments, Heat + HBO rats were exposed to HBO in a transparent hyperbaric rodent chamber (Type RDC 150-300-6, Yangquan Precision Instrument Factory, Shanxi, China). The chamber was filled with pure oxygen (100%), pressurized to 250 kPa at a rate of 25 kpa/min for 1 h and was terminated at a decompression rate of 20 kPa/min. Carbon dioxide (CO2) absorbent was placed inside the chamber to avoid CO2 retention. The temperature in the HBO chamber was maintained at 24 ± 0.5 °C for all experiments.
In in vitro experiments, Heat + HBO group BV2 cells were exposed to HBO for 1 h at 250 kPa in a sterilized hyperbaric incubator (Ox-121C, Tawang, Shanghai, China), which was flushed and compressed with oxygen-containing 2% CO2 to maintain the physiological pH of the culture. The temperature in the HBO chamber was maintained at 37 °C for all experiments. Compression and decompression were both performed within 5 min.
Neurologic deficit test
The Modified neurological severity score (mNSS) is a comprehensive measurement of performance on motor tests, which was used to evaluate neurological function before, 0 and 3 h post-heatstroke. The rats received motor test scores, sensory test scores, balance beam test scores, lack of normal reflex and abnormal movement scores. The mNSS was graded on a scale of 0–18 (normal, 0; maximal deficit, 18) [Citation27].
Hematoxylin and eosin (H&E) staining and immunohistochemistry (ICH)
Hippocampal sections (10 µm) were dewaxed and heated for 5 min in 10 mmol/L citrate buffer (pH 6.0). For immunohistochemical analysis, sections were incubated with 3% H2O2 for 15 min and blocked with 5% bovine serum albumin (BSA) (GC305010, Servicebio, Wuhan, China) for 1 h. Then, the sections were incubated with Iba-1 antibodies (22915-1-AP, 1:100, Ptg, Chicago, USA) overnight at 4 °C. The sections were incubated with horseradish peroxidase-conjugated secondary antibody for another 1 h at 37 °C after rinsing with 0.01 mol/L PBS (Beyotime Company, Jiangsu, China) as previously described [Citation28]. For histopathological analysis, sections were stained with diaminobenzidine (Beyotime Company, Jiangsu, China) and hematoxylin (Beyotime Company, Jiangsu, China). Scoring was blindly performed by two different people using the following scale: 0 = 0%, 1 = 1–20%, 2 = 21–40%, 3 = 41–60%, 4 = 61–80%, and 5 = 81–100% of the area were affected. Nuclear pyknosis, cytoplasmic condensation, and hyperchromatism are defined as neuronal injury [Citation23].
Western blotting
Protein samples were obtained from rat hippocampi or BV2 cells and treated with ice-cooled RIPA lysis buffer (Beyotime Company, Jiangsu, China). Protein concentration was assessed using an Enhanced BCA Protein Assay Kit (Beyotime Company, Jiangsu, China). Subsequently, each sample (20 µg) was electrophoresed on 10% SDS-polyacrylamide gel and transferred to polyvinylidene fluoride (PVDF) membranes (IPVH00010, Merck Millipore, Massachusetts, USA). The membranes were blocked for 1 h in 5% nonfat milk dissolved in Tris-buffered saline containing 0.1% Tween 20 and then cultured with the primary antibodies against IL-1β (A00101, 1:1000, Boster, California, USA), IL-18 (Pb0251, 1:1000, Boster, California, USA), NLRP3 (nucleotidebinding oligomerization domain-like receptor protein 3) (Ba3677, 1:1000, Boster, California, USA), ASC (apoptosis-associated speckle-like protein) (YT0365, 1:1000, Immunoway, Texas, USA), caspase-1 (22915-1-AP, 1:1000, Ptg, Chicago, USA), cleaved caspase-1 (YC0003, 1:1000 Immunoway, Texas, USA), and β-actin (66009-1-Ig, 1:2000, Ptg, Chicago, USA) as the internal reference overnight at 4 °C overnight. Then, incubated with corresponding secondary antibodies (A0453/A0423, 1:2000, Beyotime Company, Jiangsu, China). Protein bands were visualized by enhanced chemiluminescence reagent (K1129, APExBIO, Houston, USA) and analyzed using Image J software (Bio-Rad, USA).
Lactate dehydrogenase (LDH) assay
The release of LDH from BV2 cells was detected using an LDH kit (BC0685; Solarbio, Beijing, China) according to the manufacturer’s instructions. BV2 cells were seeded in 96-well plates until they reached the desired confluence. The cell supernatants were harvested and used for LDH determination. The optical density of LDH activity was measured at 450 nm using a microplate reader (MultiskanMK3, Thermo Fisher Scientific, Massachusetts, USA). The values were interpolated using a calibration curve, and the LDH release was calculated and expressed as U/L.
Cell Counting Kit-8 (CCK8) assay
The viability of BV2 cells was assessed using the CCK8 (E606335-0500, BBI Life Sciences, Shanghai, China) according to the manufacturer’s instructions. Cells were grown in 96-well plates till the appropriate time. Ten microliters of CCK-8 solution was added to each well and the cell culture plate was incubated for 3 h in the dark. Finally, absorbance was measured at 450 nm using a microplate reader.
Quantitative real-time PCR analysis
TRIzol reagent (15596-018, Invitrogen, California, USA) was used to extract Total RNA in BV2 cells according to the manufacturer’s instructions, and mRNAs were quantified by the SYBR Green dye method using SG Fast qPCR Master Mix (B639273, Sangon Biotech, Shanghai, China). β-actin mRNA was used as a housekeeping gene, and relative expression levels of mRNAs were calculated using the 2−ΔΔCq method [Citation29]. The target mRNA was amplified in the following thermocycling conditions: Predenaturation at 95 °C for 3 min; 45 cycles of 95 °C 7 s, 57 °C for 10 s, and 72 °C for 10 s. Total extension was performed at 72 °C for 10 min. The primer sequences used were as follows:
NLRP3-Forward: 5′-CCCAAGGCTGCTATCTGGAG-3′
NLRP3-Reverse: 5′-CCAGCAAACCCATCCACTCT-3′
ASC-Forward: 5′-ATGGGGCTTAGAAAGGCACC-3′
ASC-Reverse: 5′-AGAATGGCCCTGAAGCTCAC-3′
Caspase-1-Forward: 5′-ACAGAGGATTTCCCCGATGC-3′
Caspase-1-Reverse: 5′-GCAAGCCCCCGGTTTTTATG-3′
β-actin-Forward: 5′-CCTCATGCCATCCTGCGTCTG-3′
β-actin-Reverse: 5′-TTGCTCGAAGTCTAGGGCAACATAG-3′.
Immunofluorescence (IF) staining
IF staining of BV2 cells was performed to determine the expression of pyroptosis-related proteins (NLRP3, ASC, caspase-1, and cleaved caspase-1). Briefly, the BV2 cells were cultured on coverslips, fixed with 4% paraformaldehyde for 15 min, and then blocked with 5% BSA (GC305010, Servicebio, Wuhan, China) at room temperature for 1 h. Then, the sections were incubated with the primary antibodies against NLRP3 (Ba3677, 1:100, Boster, California, USA), ASC (YT0365, 1:100, Immunoway, Texas, USA), caspase-1 (22915-1-AP, 1:100, Ptg, Chicago, USA) and cleaved caspase-1 (YC0003, 1:100, Immunoway, Texas, USA) at 4 °C overnight. Subsequently, the sections were incubated with the corresponding secondary antibody (A0453/A0423, 1:400, Beyotime Company, Jiangsu, China) for 2 h. Nuclei were stained with 1 µg/mL DAPI (4′,6-diamidino-2-phenylinedole, Solarbio, Beijing, China) for 3 min, and the images were taken under fluorescence microscopy and analyzed with Image-Pro Plus 6.0 software.
Enzyme-linked immunosorbent assay (ELISA)
To determine the protein levels of inflammatory cytokines in the cell culture medium, we measured the levels of IL-1β and IL-18 in the culture medium using commercial ELISA kits (MM-0040M2, Meimian, Jiangsu, China) according to the manufacturer’s protocol. The absorbance was measured at 450 nm using a microplate reader.
Statistical analysis
All data were presented in the mean ± standard deviation (mean ± SD). Statistical analyses were performed using SPSS software (version 19.0; IBM Corp., Armonk, NY, USA) and GraphPad Prism 9.0 (GraphPad Software Inc., San Diego, CA, USA). Student t-test was employed to compare the means of the two samples. One-way analysis of variance (ANOVA) was used for comparisons among multiple groups, and the least significant difference (LSD) test was used to assess the homogeneity of variance. All experiments were conducted with a minimum of three replicates, and p < 0.05 was considered statistically significant.
Results
HBO attenuates mortality and hippocampal injury caused by heatstroke
We established a rat heatstroke model to investigate the effects of HBO on heatstroke-induced mortality and hippocampal injury. HBO significantly reduces heatstroke-induced lethality, with mortality rates decreasing from 80% to 40% (p < 0.01, ). The mean survival time of rats in the different groups was measured (). Our results demonstrated that heatstroke significantly reduced the mean survival time compared to the control group (p < 0.001), while HBO significantly improved the survival outcome of heatstroke rats (p < 0.01). Neurological function was evaluated using the mNSS test (). Rats exposed to the high-temperature environment exhibited significantly higher mNSS scores compared to the control group (p < 0.001). Over time, the mNSS scores decreased in all rats with heatstroke owing to spontaneous recovery. However, at 3 h post-heatstroke, the HBO-treated rats showed significantly lower scores than the heatstroke group (p < 0.01). Histological examination of the hippocampus was performed using H&E. Control rats exhibited no pathological changes, with clear cytoplasmic and nuclear structures. In contrast, heatstroke rats displayed edema, vacant spaces around neurons, and nuclear condensation. HBO decreased the heatstroke-induced neuronal damage (). These changes can be seen more directly from the pathologic scores analysis, which revealed a significantly higher score in the heatstroke group compared to the control group (p < 0.001), whereas the Heat + HBO group exhibited a significantly lower score than the heatstroke group (p < 0.01, ). These results suggest that HBO attenuates heatstroke-induced mortality and hippocampal injury in rats.
Figure 1. HBO alleviates heatstroke-induced mortality and hippocampal injury in vivo. (A, B) The mortality and mean survival time of rats in different groups, n = 10. (C) Neurological function in different groups was assessed by mNSS, n = 10. (D, E) The histopathological changes of the hippocampus were shown by H&E staining. Scale bars = 50 µm/400× visual field. Black arrows point to pyknotic nuclei. Data are shown as mean ± SEM. **p < 0.01 versus the Heat group, ***p < 0.001 versus the Control group, n = 6. H&E: hematoxylin and eosin; HBO: hyperbaric oxygen; mNSS: modified neurological severity score.
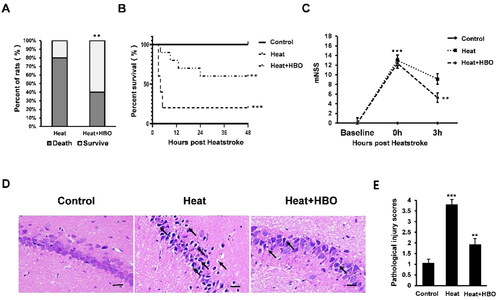
HBO mitigates microglial pyroptosis and the inflammation response in heatstroke-induced hippocampal injury
To determine the effect of HBO on the expression of pro-inflammatory cytokines in the hippocampus after heatstroke, the protein levels of IL-1β and IL-18 were measured using western blotting (). The heatstroke rats exhibited elevated protein levels of IL-1β and IL-18 (p < 0.01), whereas HBO significantly reduced hippocampal expression of IL-1β and IL-18 induced by heatstroke (p < 0.05). Pyroptosis-related proteins in the hippocampus were also detected by western blotting (). Heatstroke increased the levels of hippocampal caspase-1 and NLRP3 (p < 0.01), whereas HBO significantly decreased both caspase-1 and NLRP3 levels compared to those in the heatstroke group (p < 0.05). Considering the possible involvement of microglial pyroptosis in heatstroke-induced hippocampal inflammation, we also investigated microglial activation in the different groups using IHC with Iba-1 antibody (). As expected, IHC demonstrated that heatstroke stimulated, whereas HBO inhibited hippocampal Iba-1 expression, indicating similar expression patterns of Iba-1 as pyroptosis-related proteins. These findings suggest that HBO alleviates the inflammatory response in the hippocampus of heatstroke rats by inhibiting microglial pyroptosis.
Figure 2. HBO decreased the inflammatory cytokine release in the hippocampus of heatstroke rats. Western blot assays were performed to assess the IL-1β and IL-18 protein expression levels in the hippocampus. Data are shown as mean ± SEM. *p < 0.05 versus the Control group; **p < 0.01 versus the Heat group. n = 6. HBO: hyperbaric oxygen.
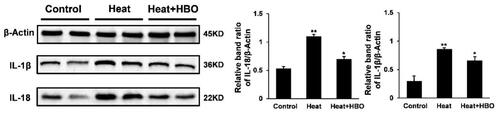
Figure 3. HBO inhibited the pyroptosis of hippocampal microglia in rats after heatstroke. (A) Western blot assays were performed to assess the caspase-1 and NLRP3 protein expression levels in the hippocampus of rats after heatstroke. Data are shown as mean ± SEM. *p < 0.05 versus the Control group; **p < 0.01 versus the Heat group, n = 6. (B) Immunohistochemical staining of Iba-1 of different groups. Black arrows point to Iba-1-positive microglia. Scale bars = 50 µm/400× visual field. HBO: hyperbaric oxygen.
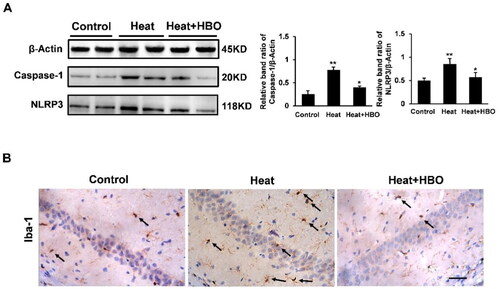
HBO alleviated the injury caused by heat stress in BV2 cells
To further verify the effect of HBO on microglia after heat stress, the BV2 cells were incubated at 40 °C with 5% CO2 for 1 h in an incubator and then treated with or without HBO. LDH release in BV2 cells was quantified at 3, 6, 12, and 24 h after heat stress using an LDH assay kit. Our results demonstrated that heat stress stimulated a gradual increase in LDH release from BV2 cells, which peaked at 12 h post heat stress and subsequently decreased at 24 h post heat stress (). It suggested that heat stress injured BV2 cells and increased the permeability of the BV2 cell membranes. In subsequent experiments, we found that HBO reduced the release of LDH from BV2 cells at 12 h post heat stress (p < 0.05) and alleviated heat-stressed BV2 cell membrane permeability (). The CCK-8 assay indicated that heat stress decreased the viability of BV2 cells compared to that of the control group (p < 0.01), and HBO reversed this result (p < 0.05, ). Collectively, these results indicate that HBO alleviated the injury caused by heat stress in BV2 cells.
Figure 4. HBO alleviated the injury caused by heat stress in BV2 cells. LDH release at different times (3, 6, 12, and 24 h) after heat stress (A) and the intervention of HBO (B) was assessed by the LDH Assay Kit (N = 3). (C) The viability of BV2 cells in different groups was assessed by the CCK-8 (N = 3). Data are shown as mean ± SEM. *p < 0.05, **p < 0.01, ***p < 0.001 versus the Control group; +p < 0.05 versus the Heat group, +++p < 0.001 versus the Control group; #p < 0.05 versus the Heat group, ##p < 0.01 versus the Control group, n = 3, each with three parallel wells. HBO: hyperbaric oxygen; LDH: lactate dehydrogenase.
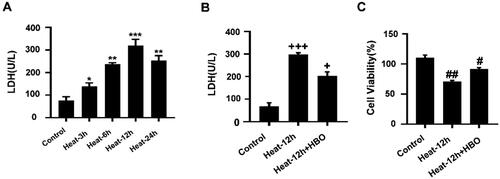
HBO attenuated pro-inflammatory cytokines expression in BV2 cells under heat stress
The expression of pro-inflammatory cytokines was analyzed in heat-stressed BV2 cells with or without HBO. As the western blots displayed, heat stress significantly elevated the level of IL-1β and IL-18 compared with those in the control group (p < 0.001). Notably, HBO markedly suppressed the production of IL-1β (p < 0.01) and IL-18 (p < 0.05) in microglia in comparison with the heat-stressed group (). Subsequently, the levels of IL-1β and IL-18 in the culture supernatants of BV2 cells from different groups were detected by ELISA. Similarly, HBO also markedly attenuated the release of IL-1β and IL-18 into the culture medium after heat stress (p < 0.05, ). These results suggest that HBO suppressed the expression and release of pro-inflammatory cytokines IL-1β and IL-18 in heat-stressed BV2 cells.
Figure 5. HBO reduced the production of pro-inflammatory cytokines caused by heat stress in BV2 cells. (A) The protein levels of IL-1β and IL-18 in different groups were detected by western blotting. (B) The levels of IL-1β and IL-18 in BV2 cell culture supernatants were determined by ELISA. Data are shown as mean ± SEM. *p < 0.05, **p < 0.01, ***p < 0.001. n = 3, each with three parallel wells. HBO: hyperbaric oxygen.
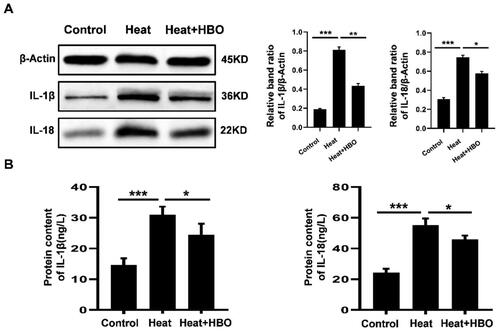
HBO prevented heat stress-induced pyroptosis of BV2 cells
NLRP3 combines with caspase-1 and ASC to form the NLRP3 inflammasome and triggers pyroptosis. We observed that heatstroke-induced activation of the NLRP3 inflammasome and pyroptosis could be mitigated by HBO in vivo, therefore, we further explored the effect of HBO on heat stress-induced microglial pyroptosis in vitro. The results of RT-qPCR () showed that, compared with the control group, the expression levels of pyroptosis-related genes (NLRP3, ASC, and caspase-1) were significantly upregulated in the heat stress group (p < 0.001). Notably, HBO led to the downregulation of these genes at the transcript level compared to the heat stress group. Consistently, western blotting () and IF staining () evidenced similar protein expression patterns of NLRP3, ASC, caspase-1, and cleaved caspase-1 as their mRNA expression. These results suggested that HBO prevents heat stress-induced microglial pyroptosis.
Figure 6. HBO prevented heat stress-induced pyroptosis of BV2 cells. (A) Relative mRNA expression of pyroptosis-related genes in different groups was detected by RT-qPCR. (B, C) Western blotting and statistical comparison of pyroptosis-related protein in different groups. (D–H) Immunofluorescence assays were conducted to explore the expression of pyroptosis-related protein in BV cells. Scale bar: 50 µm. Data are shown as mean ± SEM. *p < 0.05, **p < 0.01, ***p < 0.001, n = 3, each with three parallel wells. HBO: hyperbaric oxygen.
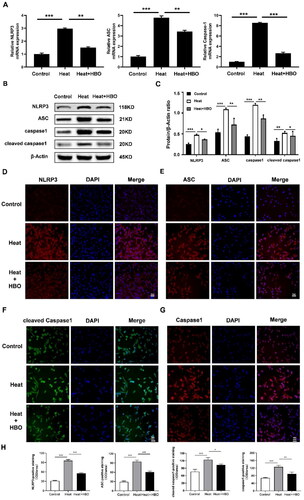
Discussion
In this study, we present a novel neuroprotective mechanism of HBO against heatstroke-induced hippocampal injury by inhibiting microglial pyroptosis and reducing neuroinflammatory response. Acute HBO therapy significantly reduced mortality and hippocampal injury of heatstroke rats by preventing microglial pyroptosis and decreasing the levels of inflammatory cytokines, IL-18 and IL-1β. In in vitro experiments, HBO also decreased the levels of inflammatory cytokines, IL-18 and IL-1β, and suppressed heat stress-induced pyroptosis in BV2 cells. Our findings provide support for the clinical application of HBO in the treatment of heatstroke-induced CNS injury and offer a novel understanding of its anti-inflammatory and neuroprotective mechanisms.
CNS injury is the main feature of severe heatstroke and patients may present with delirium, memory decline, and even coma [Citation30]. Almost all patients experience CNS damage in the early stages of heatstroke and more than 30% of patients have long-term CNS dysfunction [Citation3,Citation31]. The hippocampus is particularly vulnerable to heatstroke. Damage to the hippocampus can lead to high mortality rates and serve as an early sign of heatstroke [Citation5,Citation6]. Currently, there are no standard medications for the treatment of heatstroke-induced CNS damage. The mainstay of heatstroke treatment is still whole body cooling and support of dysfunctional organs to prevent the development of MODSs [Citation32]. However, some patients suffer from hypothermia after rapid cooling due to thermoregulatory disorder and eventually die [Citation19,Citation33]. HBO, a noninvasive and effective treatment, has been widely used in CNS injury-related diseases [Citation19,Citation23,Citation24]. Ni et al. reported that immediate HBO therapy (253 kPa, lasting for 1 or 2 h) after heatstroke effectively prevented hypothermia in rats. In addition, HBO attenuates hippocampal impairment and improves the survival and neurological function of heatstroke rats [Citation23]. Consistent with the results of previous studies, we found that HBO effectively alleviated hippocampal injury and enhanced the survival rates of heatstroke rats. Moreover, the decrease in mNSS revealed that immediate HBO treatment could effectively improve neurological dysfunction in the early stages of heatstroke.
In addition to hyperthermia, ischemia, and hypoxia, inflammation is another important mechanism underlying CNS injury caused by heatstroke. MRI results have shown abnormal inflammatory responses in the hippocampus of patients with heatstroke [Citation7]. Recent studies have shown that the levels of pro-inflammatory cytokines (IL-1, IL-6, and TNF-α) in the hippocampus are significantly increased after heatstroke; reducing the expression of pro-inflammatory cytokines can alleviate hippocampal damage [Citation34]. One of the most important goals of clinical heatstroke treatment has been to reduce or even inhibit the development of neuroinflammation. HBO reduces neuroinflammation and thus plays a neuroprotective role in CNS injury. Hui et al. found that HBO improved locomotor disability, inhibited inflammation and apoptosis in traumatic spinal cord injury rats through modulation of the mTOR signaling pathway [Citation35]. In addition, heat-induced hypothalamic ischemia, neuronal damage and inflammation are substantially reduced by HBO [Citation19]. The present study found that the expression of pro-inflammatory cytokines, IL-18 and IL-1β, in the hippocampus was significantly increased in the early stages of heatstroke (3 h post-heatstroke), and immediate HBO treatment could reduce the expression of IL-18 and IL-1β in the hippocampus of heatstroke rats. These findings suggest that HBO protects against hippocampal injury by inhibiting neuroinflammation in heatstroke rats.
Pyroptosis is a highly inflammatory form of apoptosis, characterized by the activation of inflammasome and results in a spike in pro-inflammatory cytokines, such as IL-1β and IL-18, followed by the activation of other inflammatory cells and amplification of the inflammatory response [Citation13]. As the major innate immune cells of CNS, microglia are central in neuroinflammation. Due to the high expression of pattern-recognition receptors, which can recognize pathogen-associated molecular patterns and damage-associated molecule patterns, and initiate the pyroptosis cascade [Citation36], microglia are also considered to be the main cells in the CNS where pyroptosis occurs [Citation14,Citation15]. Recently, microglial pyroptosis has been identified as a significant source of neuroinflammation in various neurological disorders [Citation37,Citation38]. In this study, it was found that in the early stage of heatstroke, the expression of pyroptosis-related proteins, NLRP3 and caspase-1, was significantly increased in the hippocampus, whereas immediate HBO intervention significantly restrained their expression. Interestingly, during these processes, the microglial marker protein, Iba-1, revealed similar protein expression patterns as pyroptosis-related proteins. These results suggest that HBO alleviates the inflammatory response in the hippocampus of heatstroke rats by inhibiting microglial pyroptosis.
The aberrant activation of NLRP3 inflammasome and pyroptosis have been described as the dominant factors in microglia-mediated neuroinflammation during the development of cerebral injury [Citation39,Citation40]. In an animal study, sepsis-associated encephalopathy was successfully alleviated by attenuating microglial pyroptosis [Citation41]. Current research also indicates that activation of the NLRP3 inflammasome is upregulated after brain trauma and inhibition of NLRP3-mediated microglial pyroptosis can alleviate traumatic brain injury [Citation42]. There is substantial evidence that pyroptosis mainly occurs in microglia in CNS neuroimmune diseases and microglia play a key role in triggering the cellular response to HBO [Citation25]. Mouse microglia have a close resemblance with the primary microglia [Citation43], therefore, we used the mouse microglial cell line, BV2, to establish a heat stress cellular model. Our in vitro studies further confirmed the role of HBO in preventing pyroptosis in heat-stressed BV2 cells. HBO successfully suppressed the expression of pyroptosis-related genes and proteins in heat-stressed BV2 cells, thus alleviating heat-induced BV2 cell damage. Furthermore, HBO suppressed the expression and release of pro-inflammatory cytokines, IL-1β and IL-18, in heat-stressed BV2 cells. The present study demonstrated that HBO successfully suppresses heat-stressed microglial pyroptosis. However, the role of HBO in the modulating of NLRP3 inflammasome activation requires further investigation. HBO treatment directly increases the formation of reactive oxygen species (ROS), which may be the molecular mechanism by which it affects NLRP3 inflammasomes [Citation44]. However, the relationship between ROS and NLRP3 inflammasome remains unclear. Some researchers believe that ROS signaling drives the formation of NLRP3 inflammasomes [Citation45], whereas others report that ROS inducers can inhibit the activation of NLRP3 inflammasomes [Citation46]. A recent study [Citation47] revealed that HBO treatment can inhibit NLRP3-dependent pyroptosis of neural stem cells and the production of inflammatory cytokines by downregulating lncRNA-H19, which significantly reduces nerve damage caused by oxygen–glucose depletion. However, overexpression of lncRNA-H19 blocked HBO-induced neural stem cell proliferation and neuronal differentiation. Therefore, further research is required to elucidate the molecular mechanisms by which HBO affects the NLRP3 inflammasomes. Nonetheless, this study has some limitations due to the complexity of neuroinflammation and nerve injury caused by heatstroke; therefore, further in vivo studies will be conducted to investigate the role of microglia during the process of heatstroke. The mechanism of microglial pyroptosis during heatstroke requires comprehensive and thorough exploration.
Conclusions
In summary, our results preliminarily confirmed that HBO could reduce neuroinflammation by suppressing microglial pyroptosis, thereby alleviating heatstroke-induced hippocampal injury both in vivo and in vitro. The findings of our study provide a new perspective on the underlying mechanisms of HBO in the treatment of heatstroke-induced hippocampus injury and provide a promising approach for the treatment of heatstroke-induced hippocampal injury.
Author contributions
This work was carried out in collaboration with all authors. ACX and GXH designed the study, XCY wrote the protocol, ZQZ and FH managed the literature research, EC and YXH performed the statistical analyses, and ACX, GXH, and XCY wrote and revised the final manuscript. All authors read and approved the final version of the manuscript.
Disclosure statement
The authors declare no competing interests.
Data availability statement
The data presented in this study are available upon request from the corresponding authors.
Additional information
Funding
References
- Bouchama A, Abuyassin B, Lehe C, et al. Classic and exertional heatstroke. Nat Rev Dis Primers. 2022;8(1):8. doi: 10.1038/s41572-021-00334-6.
- Wang L, Ye B, Liu Y, et al. Xuebijing injection attenuates heat stroke-induced brain injury through oxidative stress blockage and parthanatos modulation via PARP-1/AIF signaling. ACS Omega. 2023;8(37):33392–33402. doi: 10.1021/acsomega.3c03084.
- Lawton EM, Pearce H, Gabb GM. Review article: environmental heatstroke and long-term clinical neurological outcomes: a literature review of case reports and case series 2000-2016. Emerg Med Australas. 2019;31(2):163–173. doi: 10.1111/1742-6723.12990.
- Ni X, Liu Z, Xie Q, et al. [Cerebral injury induced by heat stroke and the therapeutic effect of hyperbaric oxygen therapy]. Zhonghua Wei Zhong Bing Ji Jiu Yi Xue. 2017;29(6):572–576. doi: 10.3760/cma.j.issn.2095-4352.2017.06.020.
- Lauretti L, D’Alessandris QG, Gessi M. Acute brainstem compression. BMJ Case Rep. 2018;2018:bcr-2018-224706. doi: 10.1136/bcr-2018-224706.
- Zhu J, Chen Y, Ji J, et al. Microglial exosomal miR-466i-5p induces brain injury via promoting hippocampal neuron apoptosis in heatstroke. Front Immunol. 2022;13:968520. doi: 10.3389/fimmu.2022.968520.
- Mahajan S, Schucany WG. Symmetric bilateral caudate, hippocampal, cerebellar, and subcortical white matter MRI abnormalities in an adult patient with heat stroke. Proc (Bayl Univ Med Cent). 2008;21(4):433–436. doi: 10.1080/08998280.2008.11928446.
- Chauhan NR, Kapoor M, Prabha Singh L, et al. Heat stress-induced neuroinflammation and aberration in monoamine levels in hypothalamus are associated with temperature dysregulation. Neuroscience. 2017;358:79–92. doi: 10.1016/j.neuroscience.2017.06.023.
- Lin YF, Liu TT, Hu CH, et al. Expressions of chemokines and their receptors in the brain after heat stroke-induced cortical damage. J Neuroimmunol. 2018;318:15–20. doi: 10.1016/j.jneuroim.2018.01.014.
- Li P, Shen T, Luo X, et al. Modulation of microglial phenotypes by dexmedetomidine through TREM2 reduces neuroinflammation in heatstroke. Sci Rep. 2021;11(1):13345. doi: 10.1038/s41598-021-92906-5.
- Lin W, Hsuan YC-Y, Su Y-C, et al. CD34− human placenta-derived mesenchymal stem cells protect against heat stroke mortality in rats. Oncotarget. 2018;9(2):1992–2001. doi: 10.18632/oncotarget.23324.
- Pan Y, Cai W, Huang J, et al. Pyroptosis in development, inflammation and disease. Front Immunol. 2022;13:991044. doi: 10.3389/fimmu.2022.991044.
- Tsuchiya K. Inflammasome-associated cell death: pyroptosis, apoptosis, and physiological implications. Microbiol Immunol. 2020;64(4):252–269. doi: 10.1111/1348-0421.12771.
- Xu S, Lu J, Shao A, et al. Glial cells: role of the immune response in ischemic stroke. Front Immunol. 2020;11:294. doi: 10.3389/fimmu.2020.00294.
- Walsh JG, Muruve DA, Power C. Inflammasomes in the CNS. Nat Rev Neurosci. 2014;15(2):84–97. doi: 10.1038/nrn3638.
- Xu S, Wang J, Zhong J, et al. CD73 alleviates GSDMD-mediated microglia pyroptosis in spinal cord injury through PI3K/AKT/Foxo1 signaling. Clin Transl Med. 2021;11(1):e269. doi: 10.1002/ctm2.269.
- Liu W, Chen Y, Meng J, et al. Ablation of caspase-1 protects against TBI-induced pyroptosis in vitro and in vivo. J Neuroinflammation. 2018;15(1):48. doi: 10.1186/s12974-018-1083-y.
- Jiang W, Liu Z, Wu S, et al. Neuroprotection of emodin by inhibition of microglial NLRP3 inflammasome-mediated pyroptosis. J Integr Neurosci. 2023;22(2):48. doi: 10.31083/j.jin2202048.
- Tai PA, Chang CK, Niu KC, et al. Attenuation of heat-induced hypothalamic ischemia, inflammation, and damage by hyperbaric oxygen in rats. J Neurotrauma. 2021;38(8):1185–1192. doi: 10.1089/neu.2010.1323.
- Baratz-Goldstein R, Toussia-Cohen S, Elpaz A, et al. Immediate and delayed hyperbaric oxygen therapy as a neuroprotective treatment for traumatic brain injury in mice. Mol Cell Neurosci. 2017;83:74–82. doi: 10.1016/j.mcn.2017.06.004.
- Yan W, Fang Z, Yang Q, et al. SirT1 mediates hyperbaric oxygen preconditioning-induced ischemic tolerance in rat brain. J Cereb Blood Flow Metab. 2013;33(3):396–406. doi: 10.1038/jcbfm.2012.179.
- Velho V, Kharosekar H, Bhople L, et al. Role of hyperbaric oxygen therapy in traumatic brain injury – evidence-based consensus. Indian J Neurotrauma. 2020;17(01):42–45. doi: 10.1055/s-0040-1713312.
- Ni XX, Nie J, Xie QY, et al. Protective effects of hyperbaric oxygen therapy on brain injury by regulating the phosphorylation of Drp1 through ROS/PKC pathway in heatstroke rats. Cell Mol Neurobiol. 2020;40(8):1253–1269. doi: 10.1007/s10571-020-00811-8.
- Ye Y, Feng Z, Tian S, et al. HBO alleviates neural stem cell pyroptosis via lncRNA-H19/miR-423-5p/NLRP3 axis and improves neurogenesis after oxygen glucose deprivation. Oxid Med Cell Longev. 2022;2022:9030771. doi: 10.1155/2022/9030771.
- Xiong XY, Liu L, Yang QW. Functions and mechanisms of microglia/macrophages in neuroinflammation and neurogenesis after stroke. Prog Neurobiol. 2016;142:23–44. doi: 10.1016/j.pneurobio.2016.05.001.
- Wang M, Cheng L, Chen Z-L, et al. Hyperbaric oxygen preconditioning attenuates brain injury after intracerebral hemorrhage by regulating microglia polarization in rats. CNS Neurosci Ther. 2019;25(10):1126–1133. doi: 10.1111/cns.13208.
- Li C, Sun R, Chen J, et al. Different training patterns at recovery stage improve cognitive function in ischemic stroke rats through regulation of the axonal growth inhibitor pathway. Behav Brain Res. 2022;421:113730. doi: 10.1016/j.bbr.2021.113730.
- He GX, Xu AC, Yu XC, et al. Heat stroke alters hippocampal and cerebellar transmitter metabonomics. World J Emerg Med. 2023;14(4):287–293. doi: 10.5847/wjem.j.1920-8642.2023.068.
- Livak KJ, Schmittgen TD. Analysis of relative gene expression data using real-time quantitative PCR and the 2−ΔΔCT method. Methods. 2001;25(4):402–408. doi: 10.1006/meth.2001.1262.
- Rav-Acha M, Shuvy M, Hagag S, et al. Unique persistent neurological sequelae of heat stroke. Mil Med. 2007;172(6):603–606. doi: 10.7205/milmed.172.6.603.
- Argaud L, Ferry T, Le Q-H, et al. Short- and long-term outcomes of heatstroke following the 2003 heat wave in Lyon, France. Arch Intern Med. 2007;167(20):2177–2183. doi: 10.1001/archinte.167.20.ioi70147.
- Chan YK, Mamat M. Management of heat stroke. Trends Anaesth Crit Care. 2015;5(2–3):65–69. doi: 10.1016/j.tacc.2015.03.003.
- Leon LR, Bouchama A. Heat stroke. Compr Physiol. 2015;5(2):611–647. doi: 10.1002/cphy.c140017.
- Wang L, Deng Z, Zhao Y, et al. Mesenchymal stem cells regulate activation of microglia cells to improve hippocampal injury of heat stroke rats. J Therm Biol. 2021;101:103081. doi: 10.1016/j.jtherbio.2021.103081.
- Chen H, Xu G, Wu Y, et al. HBO-PC promotes locomotor recovery by reducing apoptosis and inflammation in SCI rats: the role of the mTOR signaling pathway. Cell Mol Neurobiol. 2021;41(7):1537–1547. doi: 10.1007/s10571-020-00921-3.
- Vande Walle L, Lamkanfi M. Pyroptosis. Curr Biol. 2016;26(13):R568–R572. doi: 10.1016/j.cub.2016.02.019.
- Xu X-E, Liu L, Wang Y-C, et al. Caspase-1 inhibitor exerts brain-protective effects against sepsis-associated encephalopathy and cognitive impairments in a mouse model of sepsis. Brain Behav Immun. 2019;80:859–870. doi: 10.1016/j.bbi.2019.05.038.
- Lee SW, de Rivero Vaccari JP, Truettner JS, et al. The role of microglial inflammasome activation in pyroptotic cell death following penetrating traumatic brain injury. J Neuroinflammation. 2019;16(1):27. doi: 10.1186/s12974-019-1423-6.
- Freeman L, Guo H, David CN, et al. NLR members NLRC4 and NLRP3 mediate sterile inflammasome activation in microglia and astrocytes. J Exp Med. 2017;214(5):1351–1370. doi: 10.1084/jem.20150237.
- Ma C, Liu S, Zhang S, et al. Evidence and perspective for the role of the NLRP3 inflammasome signaling pathway in ischemic stroke and its therapeutic potential (review). Int J Mol Med. 2018;42(6):2979–2990. doi: 10.3892/ijmm.2018.3911.
- Jing G, Zuo J, Fang Q, et al. Erbin protects against sepsis-associated encephalopathy by attenuating microglia pyroptosis via IRE1α/Xbp1s-Ca2+ axis. J Neuroinflammation. 2022;19(1):237. doi: 10.1186/s12974-022-02598-5.
- O’Brien WT, Pham L, Symons GF, et al. The NLRP3 inflammasome in traumatic brain injury: potential as a biomarker and therapeutic target. J Neuroinflammation. 2020;17(1):104. doi: 10.1186/s12974-020-01778-5.
- Henn A, Lund S, Hedtjärn M, et al. The suitability of BV2 cells as alternative model system for primary microglia cultures or for animal experiments examining brain inflammation. Altex. 2009;26(2):83–94. doi: 10.14573/altex.2009.2.83.
- Matsunami T, Sato Y, Hasegawa Y, et al. Enhancement of reactive oxygen species and induction of apoptosis in streptozotocin-induced diabetic rats under hyperbaric oxygen exposure. Int J Clin Exp Pathol. 2011;4(3):255–266.
- Tschopp J, Schroder K. NLRP3 inflammasome activation: the convergence of multiple signalling pathways on ROS production? Nat Rev Immunol. 2010;10(3):210–215. doi: 10.1038/nri2725.
- Maier NK, Crown D, Liu J, et al. Arsenic trioxide and other arsenical compounds inhibit the NLRP1, NLRP3, and NAIP5/NLRC4 inflammasomes. J Immunol. 2014;192(2):763–770. doi: 10.4049/jimmunol.1301434.
- Arienti C, Pignatta S, Zanoni M, et al. High-pressure oxygen rewires glucose metabolism of patient-derived glioblastoma cells and fuels inflammasome response. Cancer Lett. 2021;506:152–166. doi: 10.1016/j.canlet.2021.02.019.