Catheters have been an indispensable tool in medical practice since ancient times, their use dating back to the sixth century BC by the Indian surgeon Sushruta [Citation1]. Originally made using materials like gold, silver, iron, and wood, catheters have evolved into advanced designs over the centuries across the world. Benjamin Franklin's 1752 invention of a silver catheter made of hinged segments of tubes may be considered the first flexible catheter in recorded history []. The modern balloon-based self-retaining catheter, introduced in 1933, marked a turning point in catheter design and development.
Figure 1. The flexible silver catheter designed by Benjamin Franklin in 1752 [Citation2].
![Figure 1. The flexible silver catheter designed by Benjamin Franklin in 1752 [Citation2].](/cms/asset/de505387-70f2-4283-bfa5-db1cc64b66e1/ysue_a_2240991_f0001_oc.jpg)
Today, three main types of catheters are used: indwelling, external, and short-term catheters, which are available in various sizes, materials (including latex, silicone, Teflon, PVC, etc.), and types (straight or coude tip).
The invasive nature of catheters comes with risks of microbial growth and incompatibility with the human system, leading to infection, inflammation and device rejection and the need to change them frequently especially in the elderly. Catheter-associated urinary infections (CAUTIs) pose a significant concern, contributing to increased mortality rates and substantial economic burdens. UTIs account for 20 to 40% of hospital-associated infections, with an estimated 80% linked to urinary catheters [Citation3].
There has been increasing interest in developing surface modification techniques to afford microbicidal properties and biocompatibility to catheter surfaces, as seen in the increasing number of publications in the area []. These surface modification methods involve the use of coatings or physical micro- and nano-dimensional surface modifications [Citation4].
Figure 2. The number of papers obtained from Scopus using the keywords catheter AND surface AND modification.
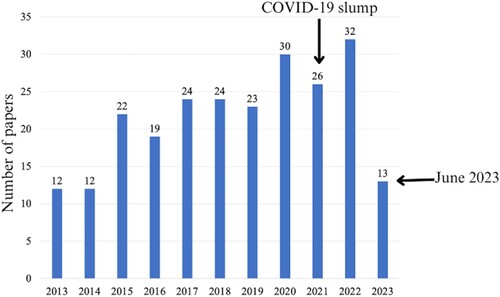
Coatings can be classified based on their mechanism of action: passive strategies include antifouling surfaces, while active approaches involve antimicrobial coatings that disrupt biological pathways.
Antifouling coatings, especially hydrogels [Citation5], poly(tetrafluoroethylene) [Citation6], polyzwitterions [Citation7], and poly(ethylene glycol) [Citation8] are being explored. These coatings are often loaded with antimicrobial agents such as antibiotics, biocidal enzymes, and bacteriophages [Citation9]. The agents prevent CAUTIs through mechanisms such as the slow release of microbicidal chemicals, modifying catheter surfaces to prevent microbial adherence, and disrupting biofilms that allow pathogen colonization. For example, researchers developed a poly(sulfobetaine methacrylate)-tannic acid hydrogel coating loaded with antimicrobials (poly(vinylpyrrolidone)-iodine, copper ions, and nitrofurazone) through non-covalent interactions. The coating exhibited pH-responsive release of the antibacterial agents under alkaline conditions, offering improved antibacterial activity against urease-producing bacteria [Citation10].
Urinary catheters have been coated with thin layers of silver in the form of silver oxide or silver alloy, as well as noble metal alloys (gold, silver, and palladium), to reduce bacterial adherence to their surfaces []. Polymeric coatings have been loaded with these noble metal species as well [Citation11].
Diamond-like coatings (DLC) are biocompatible and offer advantageous properties like low friction, smoothness, and abrasion resistance, making them ideal for medical devices. In a recent study, DLC was deposited into 2 mm inner diameter silicon catheter tubes. Bacterial adhesion and biofilm formation were evaluated using clinical isolates. Results showed reduced adherence and biofilm formation on the DLC-coated samples compared to uncoated ones, indicating their potential for medical applications [Citation13].
Antibiotics such as nitrofurazoneon [Citation14] and minocycline–rifampicin [Citation15] have been immobilized on catheter surfaces. Two approaches have been used for the immobilization. In the first approach, a layer of antibiotic is applied to cover the surface, leading to rapid drug elution. Alternatively, the antibiotic can be directly impregnated into the device polymer during production, either with or without excipients to control the drug release rate.
Figure 3. Antibacterial action of gold nanoparticles, silver nanoparticles, and their ions targeting seven specific bacterial cell components. Sizes not to scale. Image reproduced without modification from [Citation12].
![Figure 3. Antibacterial action of gold nanoparticles, silver nanoparticles, and their ions targeting seven specific bacterial cell components. Sizes not to scale. Image reproduced without modification from [Citation12].](/cms/asset/177453e9-0fea-4dab-afcd-644f0369d409/ysue_a_2240991_f0003_oc.jpg)
A successful two-step polydopamine-based surface modification strategy has been reported to co-immobilize an antimicrobial peptide (Palm) and an enzyme targeting a crucial component of biofilm matrix (DNase I) on polydimethylsiloxane surfaces. This approach provided the surfaces with both anti-adhesive and antimicrobial properties against relevant bacteria, both in single and dual-species scenarios. The modified surfaces demonstrated excellent stability, biocompatibility, and anti-biofilm capabilities. [Citation16]
More recently, catheter surfaces were modified with Lactobacilli probiotics using a ‘bacterial interference’ approach. The probiotics competed for adhesion to the catheter surface and secreted antimicrobial compounds effective against uropathogens like Escherichia coli. 3D-bio printed Lactobacillus rhamnosus-containing silicone scaffolds for urinary catheterization applications demonstrated sustained recovery of live bacteria, lactic acid, and hydrogen peroxide production over 14 days. This study highlights a potential alternative strategy to prevent and treat catheter-associated urinary tract infections through probiotic incorporation using 3D bioprinting [Citation17].
One other problem of invasive devices is that the body may reject them as a foreign entity. Catheter-induced inflammation triggers the release of proteins such as fibrinogen from the bloodstream into the bladder. While fibrinogen typically serves as a healing agent that aids in blood clotting, within the catheterized bladder, it can have an unintended effect. It acts as a ‘basket,’ gathering more microbes and contributing to the spread of infection to the bladder. A joint study by the University of Notre Dame and the University of Maine demonstrated that liquid-infused silicone catheters not only prevent fibrinogen adhesion but also improve catheter flexibility, reducing bladder scratches. These scratches trigger the liver to produce more fibrinogen, which becomes readily available in the bloodstream [] [Citation18].
Figure 4. The reduction of bladder inflammation using liquid-infused silicone (LIS)-catheter. Image reproduced without modification from [Citation18].
![Figure 4. The reduction of bladder inflammation using liquid-infused silicone (LIS)-catheter. Image reproduced without modification from [Citation18].](/cms/asset/6803ef6b-4ccb-4c28-8909-c8ca350b328f/ysue_a_2240991_f0004_oc.jpg)
The drawbacks of catheters featuring protective coatings, such as coating instability, limited short-term antibacterial effectiveness, and reduced efficacy against a narrow spectrum of bacterial species, hinder their practical application for in-dwelling (permanent) and long-term catheterization [Citation19]. Furthermore, the use of antibiotics in coatings can result in antibiotic-resistant bacteria, potentially undermining the efficacy of these drugs even in non-biofilm-related infections. Finally, the use of noble metals can have cost implications [Citation20].
Incorporating micro- or nano-scaled irregularities on catheter surfaces effectively prevents biofouling and overcomes the drawbacks of coating-based protection. Such micro and nanostructured surfaces are found extensively in nature and are aimed at protecting various life forms from bacterial attack. Sea organisms like sharks, pilot whales, sea stars, and mussels have been studied as models for biomimetic antibacterial surfaces, featuring special microstructures that effectively prevent fouling organisms due to their specific spacing and patterns [].
Figure 5. Surface topography of natural models that resist fouling: (a) pilot whale, (b) shark, (c) sea stars, and (d) mussels; scale bars are 1, 100, 100, and 10 µm, respectively. Image adapted from [Citation21].
![Figure 5. Surface topography of natural models that resist fouling: (a) pilot whale, (b) shark, (c) sea stars, and (d) mussels; scale bars are 1, 100, 100, and 10 µm, respectively. Image adapted from [Citation21].](/cms/asset/4bc6ae1f-141b-4649-8acd-7c862ab3e8a5/ysue_a_2240991_f0005_oc.jpg)
In 2011, the effectiveness of a unique microscopic physical surface modification known as Sharklet was investigated to prevent bacterial colonization and migration of uropathogenic E. coli on silicone elastomer. The sharklet micropattern was able to inhibit the colonization and migration of this common uropathogen.
In another study, silicone was micropatterned using microsecond laser ablation [Citation20]. Bacterial adhesion on these patterned surfaces was seen to depend on the cone size; cones of 20 and 40 μm diameter had minimal bacterial adhesion, whereas 25 and 30 μm diameter cones showed higher bacterial coverage []. Controlled micropatterning is thus, a potential method to prevent unwanted biofilm formation in catheters, while encouraging beneficial biofilms for specific applications.
Figure 6. E Coli adhesion on patterned silicone surfaces with cones of diameters (a) 20 μm (b) 25 μm (c) 30 μm and (d) 40 μm, after a 5-hour contact period. Image adapted from [Citation20].
![Figure 6. E Coli adhesion on patterned silicone surfaces with cones of diameters (a) 20 μm (b) 25 μm (c) 30 μm and (d) 40 μm, after a 5-hour contact period. Image adapted from [Citation20].](/cms/asset/adaab5df-52e2-4497-83e9-cbb5f14ec1d5/ysue_a_2240991_f0006_oc.jpg)
Lasers are employed to achieve precise, micro-sized features or patterns on catheter surfaces, enhancing their performance and functionality. A rougher surface facilitates stronger bonding or welding of parts, while a textured or patterned surface aids in managing surface tension and wear or corrosion when moving components come into contact or slide along the same plane or surface [Citation22]. Laser surface texturing also enhances the stability/adhesion of endothelial cells, leading to biocompatibility [Citation23].
A technique that combined coating and nanostructure was recently reported to produce superhydrophobic coatings on catheters [] [Citation24]. A layer-by-layer deposition technique was used to produce a mussel-inspired polydopamine coating on catheters, facilitating in situ anchoring of silver nanoparticles. Subsequent hydrophobic modification with 1H,1H,2H,2H-perfluorodecanethiol resulted in hierarchical micro/nanostructures. The prepared catheters exhibited excellent super-hydrophobicity and prolonged antibacterial activity against E. coli and Proteus mirabilis. Compared to commercial catheters, the superhydrophobic version displayed significant antibiofilm effects, delaying bacterial migration and reducing biomass accumulation. Encrustations in the catheter lumen were also retarded, extending the catheter's lifespan. Additionally, the superhydrophobic catheter demonstrated good biocompatibility with L929 mouse fibroblasts, suggesting a promising direction for future urinary catheter design.
Figure 7. Production of superhydrophobic hierarchically micro/nanostructured coatings for catheters. Schematic reproduced without modification from [Citation24].
![Figure 7. Production of superhydrophobic hierarchically micro/nanostructured coatings for catheters. Schematic reproduced without modification from [Citation24].](/cms/asset/273e6cbb-feee-4632-bf5a-b4e5fea2d9f7/ysue_a_2240991_f0007_oc.jpg)
Catheter surface modification has shown significant progress in combating catheter-associated urinary infections. Various techniques, including coatings and micro/nanostructures inspired by nature, have been explored to prevent bacterial adhesion and biofilm formation. These advancements hold great promise for the development of more effective and biocompatible urinary catheters, reducing the risks of infection and improving patient outcomes. Continued research and innovation in this field are expected to lead to further improvements in catheter design and performance. The opportunity to use new combinations of materials as well as additive manufacturing can vastly expand the tailoring of surfaces for each patient. In addition, tissue engineering is growing such that the cells from the individual can be grafted on to some of these catheters as a coating to avoid any infection or reaction causing discomfort. Cost is always an issue in the final application and the ability to have coatings that are approved by the FDA remains a major hurdle for any new approaches
References
- Kumar P, Khan M. The history of the catheter - from Sushruta to Foley. J Urol. 2009;181(4):386–386. doi:10.1016/S0022-5347(09)61095-8
- The Franklin Institute. “Benjamin Franklin’s Inventions,” https://www.fi.edu/en/benjamin-franklin/inventions. [Online].
- Werneburg GT. Catheter-associated urinary tract infections: current challenges and future prospects. Res Rep Urol. Apr. 2022;14:109–133. doi:10.2147/RRU.S273663.
- Gopal L, Sudarshan T. Functional surfaces through texture management. Surf Eng. Mar. 2023;39(3):239–244. doi:10.1080/02670844.2023.2225004.
- Kaufmann AM, Lye T, Redekop G, et al. Infection rates in standard vs. hydrogel coated ventricular catheters. Can J Neurol Sci / J Canadien des Sciences Neurologiques. Nov. 2004;31(4):506–510. doi:10.1017/S0317167100003723.
- Zhang S, Wang L, Liang X, et al. Enhanced antibacterial and antiadhesive activities of silver-PTFE nanocomposite coating for urinary catheters. ACS Biomater Sci Eng. Jun. 2019;5(6):2804–2814. doi: 10.1021/acsbiomaterials.9b00071.
- Wang X, Wang J, Yu Y, et al. A polyzwitterion-based antifouling and flexible bilayer hydrogel coating. Compos B Eng. Sep. 2022;244:110164. doi:10.1016/j.compositesb.2022.110164. [Online].
- Sankar S, Rajalakshmi T. Application of poly ethylene glycol hydrogel to overcome latex urinary catheter related problems. BioFactors. 2007;30(4):217–225. doi:10.1002/biof.5520300403.
- Al-Qahtani M, Safan A, Jassim G, et al. Efficacy of anti-microbial catheters in preventing catheter associated urinary tract infections in hospitalized patients: a review on recent updates. J Infect Public Health. Nov. 2019;12(6):760–766. doi:10.1016/j.jiph.2019.09.009.
- Miao J, Wu X, Fang Y, et al. Multifunctional hydrogel coatings with high antimicrobial loading efficiency and pH-responsive properties for urinary catheter applications. J Mater Chem B. 2023;11(15):3373–3386. doi: 10.1039/D3TB00148B.
- Sun Y, Ren P, Long X. Role of noble metal-coated catheters for short-term urinary catheterization of adults: a meta-analysis. PLoS One. Jun. 2020;15(6):e0233215. doi:10.1371/journal.pone.0233215. [Online].
- Joshi AS, Singh P, Mijakovic I. Interactions of gold and silver nanoparticles with bacterial biofilms: molecular interactions behind inhibition and resistance. Int J Mol Sci. Oct. 2020;21(20):7658. doi:10.3390/ijms21207658. [Online].
- Watari S, Wada K, Araki M, et al. Intraluminal diamond-like carbon coating with anti-adhesion and anti-biofilm effects for uropathogens: a novel technology applicable to urinary catheters. Int J Urol. Dec. 2021;28(12):1282–1289. doi:10.1111/iju.14675.
- Singha P, Locklin J, Handa H. A review of the recent advances in antimicrobial coatings for urinary catheters. Acta Biomater. Mar. 2017;50:20–40. doi:10.1016/j.actbio.2016.11.070.
- Bonne S, Mazuski JE, Sona C, et al. Effectiveness of minocycline and Rifampin vs Chlorhexidine and silver sulfadiazine-impregnated central venous catheters in preventing central line-associated bloodstream infection in a high-volume academic intensive care unit: a before and after trial. J Am Coll Surg. Sep. 2015;221(3):739–747. doi:10.1016/j.jamcollsurg.2015.05.013.
- Alves D, Magalhães A, Grzywacz D, et al. Co-immobilization of Palm and DNase I for the development of an effective anti-infective coating for catheter surfaces. Acta Biomater. Oct. 2016;44:313–322. doi:10.1016/j.actbio.2016.08.010.
- Kyser AJ, Mahmoud MY, Johnson NT, et al. Development and characterization of Lactobacillus rhamnosus - containing bioprints for application to catheter-associated urinary tract infections. ACS Biomater Sci Eng. Jul. 2023;9(7):4277–4287. doi:10.1021/acsbiomaterials.3c00210.
- Andersen MJ, Fong CK, La Bella AA, et al. Inhibiting host-protein deposition on urinary catheters reduces associated urinary tract infections. Elife. Mar. 2022;11; doi:10.7554/eLife.75798.
- Pascual A. Pathogenesis of catheter-related infections: lessons for new designs. Clin Microbiol Infect. May 2002;8(5):256–264. doi:10.1046/j.1469-0691.2002.00418.x.
- Perni S, Prokopovich P. Micropatterning with conical features can control bacterial adhesion on silicone. Soft Matter. 2013;9(6):1844–1851. doi:10.1039/C2SM26828K.
- Rigo S, Cai C, Gunkel-Grabole G, et al. Nanoscience-based strategies to Engineer antimicrobial surfaces. Adv Sci. May 2018;5(5):1700892. doi: 10.1002/advs.201700892. [Online].
- Spectrum Plastics Group. Lasers & Catheter Technologies. https://www.spectrumplastics.com/components-technology/catheter-technologies/lasers-catheter-technologies/. [Online].
- Purnama A, Furlan V, Dessi D, et al. Laser surface texturing of SS316L for enhanced adhesion of HUVECs. Surf Eng. Dec. 2020;36(12):1240–1249. doi:10.1080/02670844.2018.1495408.
- Zhang S, Liang X, Gadd GM, et al. Superhydrophobic Coatings for Urinary Catheters To Delay Bacterial Biofilm Formation and Catheter-Associated Urinary Tract Infection. ACS Appl Bio Mater. Jan. 2020;3(1):282–291. doi:10.1021/acsabm.9b00814.