ABSTRACT
Liquid crystals (LCs) have been studied extensively in the visible range for their dielectric tunability, and the characterisation in the terahertz (THz) range has gained increasing interest due to the need for active THz modulation and switching devices. In this paper, we use THz time-domain spectroscopy to measure the frequency-dependent birefringence and the absorption coefficient of a number of commercial and non-commercial nematic LCs, including E7, BL037, MDA-98-1602, LCMS-107, GT3-23001 and 1825, over a range of bias voltages at room temperature. Furthermore, several basic components of LC mixture are analysed to establish their contributions to birefringence and theoretical model is used to fit the absorption spectra. The large tunability and low loss measured for a range of samples show that the LCs are useful tunable dielectrics for compact, efficient and broadband THz devices.
GRAPHICAL ABSTRACT
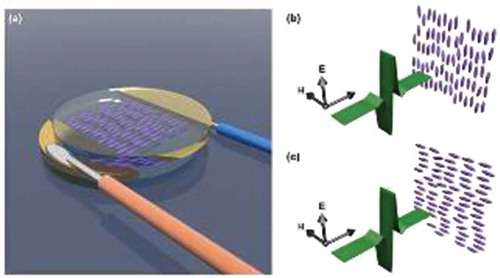
KEYWORDS:
Introduction
The terahertz (THz) spectrum, which spans the gap between microwaves and infrared, has drawn increasing attention over the past two decades.[Citation1,Citation2] Recent development of powerful THz source such as the quantum cascade laser [Citation3] and the availability of efficient spectroscopic characterisation techniques such as THz time-domain spectroscopy (THz-TDS) [Citation4] led to a rapidly growing research area. A range of characteristics of material responses can be investigated at THz frequencies, such as lattice vibrations in crystalline solids,[Citation5] rotational transitions of molecules [Citation6] and intra-band absorption in semiconductors.[Citation7] Consequently, THz technology is finding use in an increasingly wide variety of applications including biomedical sensing [Citation8,Citation9] and wireless communications.[Citation10,Citation11]
However, efficient and reliable THz applications not only require high-performance sources and detectors, but passive or active components for THz wave modulation are also essential.[Citation12,Citation13] Tunable dielectrics with high tunability, low-loss tangent and low dichroism are basic requirements for such applications. Among all existing materials, liquid crystals (LCs), which are organic compounds possessing one or more characteristic phases, have proved their efficiency and reliability in active optical components and systems especially in flat panel displays.[Citation14] Apart from their birefringent nature, the dielectric properties of LCs can easily be tuned by applying electric/magnetic fields or changing temperature. In recent years, the characterisation at THz frequencies of different commercially available or home-made LCs has drawn increasing attention.[Citation15–Citation19] Compared with the visible region, LCs typically exhibit relatively small birefringence and high loss due to the requirement of much larger device thickness for THz radiation,[Citation20,Citation21] leading to disadvantages in the switching on/off time of LCs-based devices given that it is proportional to the square of the effective LCs layer thickness. Thus, high birefringence and low-loss LCs could facilitate fast, efficient and compact THz applications.
Wilk et al. have systematically characterised the cyanobiphenyl (CB) family of LCs and their mixtures in the THz range.[Citation22] These studies demonstrated that an increased π-electron structure in the LC molecules leads to higher THz birefringence. Even with the advantage of low dichroism for extraordinary and ordinary polarisation, these LCs show low birefringence (~0.1). Park et al. investigated the complex refractive index of the LC mixture E7 and BL037 in the THz spectrum.[Citation15] These mixtures, which are composed of CB and other compounds, have relatively large THz birefringence and low viscosity. Recently, other new materials such as NJU-LDn-4,[Citation16] LC 2002 [Citation23] and LC 2010 [Citation24] were reported as suitable for THz applications due to their large birefringence (>0.3) and low absorption. In addition, Kowerdziej et al. reported large tunability of THz metamaterials infiltrated with high birefringent LCs.[Citation25]
In this paper, we investigate the complex THz refractive index and voltage response of different LC mixtures: E7, BL037, MDA-98-1602, LCMS-107, GT3-23001 and 1825. LCMS-107 was purchased from LCMS Ltd. Co., 1825 was formulated and produced by the research group at the Military University of Technology in Warsaw and the remaining of LCs are from Merck (Merck KGaA, Darmstadt, Germany). All of these LCs were designed to have high birefringence and low losses. We compare our data with previous work and more data are obtained for new LC materials. The voltage responses are analysed to interpret the alignment for these LC cells. In addition, absorption spectrum are discussed and fitted into characteristic Poley absorption.
Experiment and data collection
As shown in , the LC cells were assembled in a sandwich configuration. Two 1-mm-thick z-cut quartz plates were chosen as both THz and optically transparent window material. The rigidness, flatness and temperature tolerance provided by the quartz plates are much better than normal polymer substrates such as TPX despite the lower refractive index of the polymer material. A polyimide (PI) thin layer was spin coated on the inner side of the quartz and baked for solvent evaporation. An alignment layer was produced by mechanical rubbing of the PI layer. Two 500-µm-thick copper plates were used both as cell spacer and electrode. Due to the lack of transparent conductor in THz region, we use this structure because there is no need to switch the LC vertically to measure the ordinary refractive index. Instead, we can measure it by simply rotating the cell by 90°. The quartz plates were intentionally mismatched to expose the electrode and facilitate LC filling. We soldered two wires onto the copper plate for applying voltage and fill the LC on temperature-controlled stage under which all the LCs are initially at isotropic phase at rather high temperature. Nematic phase was developed through the temperature cooling down to clearing point and the whole structure was sealed with an UV-cured glue.
Figure 1. (colour online) (a) Schematic illustration of fabricated LCs cell for THz-TDS measurement. (b, c) The extraordinary and ordinary configurations, respectively.
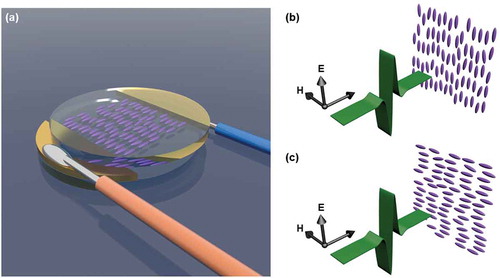
The experiments were performed using a standard THz-TDS system at an ambient temperature of 294 K. As described previously,[Citation26] the generation of the THz pulses was achieved with photoconductive antenna and detection using electro-optical sampling technique using a ZnTe crystal. The measurement chamber was purged with dry nitrogen to remove water vapour from the THz path and the samples were placed at the focal point of the parabolic mirrors. Firstly, two attaching quartz plates were characterised as the reference. Then, LC samples were measured by applying a 1 kHz bipolar square wave in two orthogonal directions (, ). Amplitude and phase information of the samples were extracted using Fast Fourier transform (FFT) processing of the time-domain signal. It should be mentioned that the THz pulse was truncated after 15 ps to cut off Fabry–Perot echoes from the multireflection caused by the substrate and LCs. Such a truncation of the time-domain pulse limits the spectral resolution after FFT as the spectral resolution is inversely proportional to the pulse length and the frequency band is dependent on the time-domain data resolution. In order to achieve a high signal-to-noise ratio, the frequency range considered in this paper is 0.2–2.5 THz and the frequency resolution is 18 GHz. Broader band (0.2–20.0 THz) response, however, was demonstrated with plasma generation and air-biased coherent detection.[Citation27] By comparing the measurements from the LC samples with those acquired from an empty reference cell, the refractive index and absorption coefficient can easily be extracted. In order to ensure the data accuracy, the reference cell was measured separately for every cell and a small air gap between (D = 10 µm) was considered when processing the experimental data.
Data analysis and discussion
The LC mixture E7 is composed of four different compounds – 5CB, 7CB, 8OCB and 5CT – and it has been extensively studied in electro-optic applications due to its comparatively large birefringence in the visible range and the broad temperature range of its nematic phase. The relatively large birefringence is strongly caused by the cyano (CN) terminal group. Measured at THz spectrum (0.2–2.5 THz), E7 exhibits a broadband flat refractive index and an increasing absorption coefficient, as shown in . Compared with the visible range, the refractive index in its ordinary state (no) is rising while that in extraordinary state (ne) is almost the same. Therefore, the birefringence measured in THz is smaller than that in optical frequencies. Both ordinary states show slight descending refractive index value with the increase of frequency (). Moreover, due to the large thickness of the LC, the surface energy of the alignment layer is not strong enough to align all the LC molecules in the cell. Therefore, when no voltage is applied, the birefringence is as small as 0.05 for E7. The strong electrical field applied in plane would pull the LC molecules in one direction and make for good alignment. It should be mentioned that the threshold voltage or electrical field is strongly dependent on the dielectric anisotropy of the LC. The birefringence is around 0.15 across the measured frequency window. The absorption coefficients for both extraordinary and ordinary states increase with frequency. This is due to the absorption peak occurring at higher THz frequencies, which is normally called Poley absorption. Moreover, E7 shows large dichroism with ordinary state absorbing more THz field than extraordinary sate. Our measured values are consistent with a previous report.[Citation18]
Figure 2. (colour online) Refractive index (a) and absorption coefficient (b) of E7 at different voltages.
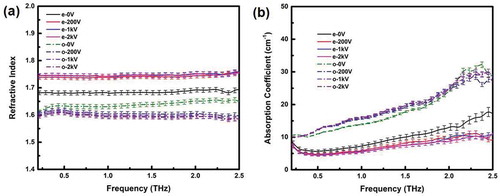
Regarding the chemical constituents, the structure of BL037 is similar to E7 except that the CB compounds are replaced with alkyl and alkoxy groups. In addition, it contains LC components with three-ring systems (biphenyl/cyclohexane and terphenyls). It has been reported that three benzene rings connected directly or with bridge group exhibit larger birefringence than structures with fewer rings.[Citation28] As shown in , BL037 has a flat refractive index in THz spectrum and the birefringence value increases with frequency from 0.17 at 0.5 THz to 0.21 at 2.5 THz. However, the absorption in the ordinary states increases dramatically with frequency (44.6 cm−1 at 2.5 THz), while the extraordinary absorption slope is much less steep. This is due to the fact that the movement in the long-axis direction is easier than the movement around the other two short axes in most of the LC material, and thus vibrations induced by extraordinary waves are more hindered.
Figure 3. (colour online) Refractive index (a) and absorption coefficient (b) of BL037 at different voltages.
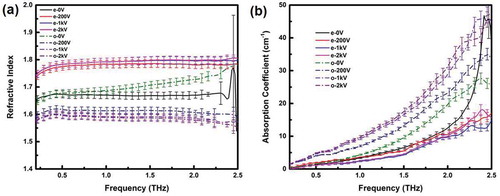
Despite the fact that detailed descriptions of the MDA-98-1602 are not yet revealed, its properties resemble that of BL037 at THz frequencies, as shown in . At room temperature, its birefringence () at visible wavelength is slightly smaller than that of BL037 (
). While its birefringence at THz frequencies is in the similar range of 0.16–0.20, as shown in . The absorption spectrum of MDA-98-1602 resembles that of BL037 as well, as shown in .
Figure 4. (colour online) Refractive index (a) and absorption coefficient (b) of MDA-98-1602 at different voltages.
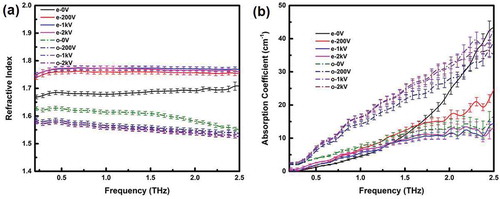
The LC LCMS-107 is composed of a mixture of isothiocyanate (NCS) terminal compounds. According to Reuter et al. [Citation28], LCs with NCS groups enable higher birefringence over a broad temperature range than CN groups and have rather low viscosity. This material has been studied by Trushkevych et al. [Citation29], but they did not use very efficient alignment schemes such as high voltage and the THz band they measured is limited to 0.5–1.6 THz. In our 0.2–2.5 THz spectrum (), LCMS-107 shows a high birefringence (~0.3) across the entire band. And interestingly, the absorption has a crossing point (αe = αo) at 1.0 THz and the absorption difference disappears again at around 2.5 THz. At these frequencies, it is possible to achieve phase-only modulation of the incoming THz wave. Such large birefringence is very promising for a variety of THz applications.
Figure 5. (colour online) Refractive index (a) and absorption coefficient (b) of LCMS-107 at different voltages.
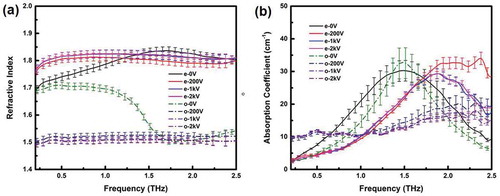
LC mixture 1825 comprising NCS compounds belong to seven families.[Citation30] In order to achieve high birefringence, the mixture was purposely designed to contain the combination of cyclohexane and benzene multiring systems with various bridge groups (single bond or C=C). The refractive index and absorption coefficient for both ordinary and extraordinary states are shown in . The refractive index with birefringence , does not change much under different applied voltages, showing that the LC molecules are well aligned under the effect of alignment layers. Furthermore, the average absorption is smaller than the previously described samples. Compared with the visible range (
at 633 nm, temperature), 1825 retains its high birefringence and low dichroism at THz frequencies, which makes it favourable over other nematic LCs for applications in compact THz devices and applications with high efficiency.
Figure 6. (colour online) Refractive index (a) and absorption coefficient (b) of 1825 at different voltages.
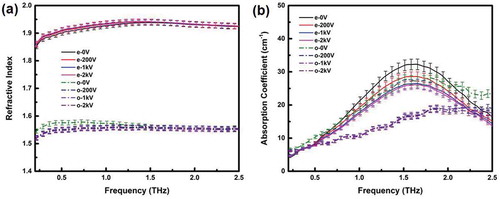
GT3-23001 is a relatively new LC mixture specially designed for low-frequency applications such as in microwaves. Its detailed composition is not publically available. The THz response in this material () is similar to that of BL037 or MDA-98-1602, with a broadband birefringence of . However, its loss parameters and dichroism are smaller than the values observed for the other two.
Discussion
Under strong in-plane alignment effect, the refractive indices and absorption coefficient measured for six different LC samples between 0.2 and 2.5 THz are shown in and , respectively. At room temperature, all the six different nematic LC mixtures exhibit near-flat birefringence ( 0.14–0.38) in the THz range. And these birefringence values measured are smaller than those in the visible range. At low THz frequencies, the refractive indices are nearly independent of frequency and the absorption coefficients are mostly rising with frequency. These materials have a positive anisotropy with ne > no and dichroism αe < αo for CN mixtures in the all investigated THz range, while αe > αo for mixtures comprising isothiocyanato compounds above 1.0 THz range. It has been stated [Citation31] that there are several factors influencing the birefringence: terminal groups, ring structures and bridge groups. From the available LC constituent data we have, molecular mixtures with more benzene rings, NCS terminal and triple bridge bond exhibit larger birefringence compared to those in the normal CB family. A recent systematic study of the impact of the terminal groups on birefringence can be found in ref. [Citation28]. Absorption in LC materials is mainly caused by torsion and reorientation movements in parts of the molecules such as rings, chains and polar substitutes. For the low THz frequencies we studied here, both the ordinary and extraordinary absorption are rising with frequency, which is generally attributed to Poley absorption [Citation32] at higher frequency. Some of the LCs have a crossing point at absorption spectrum where the dichroism is smallest and LCs can be effectively tuned without changing the absorption. At those frequencies, LC cell can be considered as a phase-only device similar to the liquid crystal on silicon spatial light modulator. Above all, in order to get high birefringence and low-loss LC materials, one of the most important principles for their formulation is mixing polar higher birefringence compounds with non-polar low birefringence hydrocarbons with very small rotational viscosity.
Figure 8. (colour online) Comparison of refractive indices (a) and absorption coefficient (b) of different nematic LC materials in THz range. The legend in the refractive index (left) is the same as that in absorption coefficient graph (right).
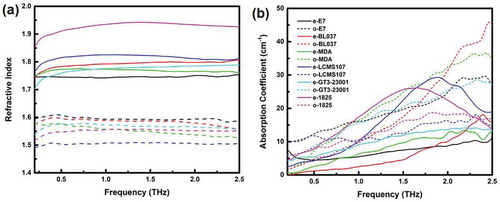
Furthermore, in order to locate the resonance frequency which is located outside of the measured THz-TDS window, we fitted some of the measured LC absorption coefficients with Gaussian line shape model. We chose Gaussian instead of conventional Lorentzian model due to the fact that there are many sources of broadening for molecules in the liquid state. In this simple model, we can describe the absorption spectra with the following equation: . As an example, the Gaussian shape fits well into the measurement data of the ordinary state of E7, shown in . And the fitting parameters are
, peak frequency
, and broadening factor
. However, there are multiple vibrations contributing to the absorption at high THz frequencies and normally the line shape is not symmetrical as shown in ultra-broadband THz measurement.[Citation27]
Figure 9. (colour online) Calculated absorption spectrum of E7 ordinary state. The dotted line indicates a Gaussian fit.
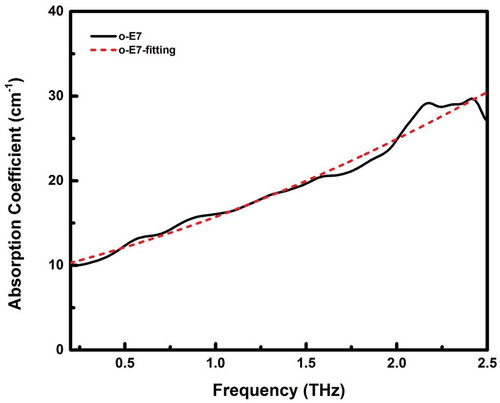
Despite the increasing number of investigations into the THz response of LCs at THz frequencies, only a small fraction of existing LCs have been characterised and a range of materials such as ferroelectric, cholesteric and polymer-dispersed LCs remain to be analysed. In addition, the analysis of the effect of divergent molecule structures or compounds on the refractive indices and absorption will be necessary to identify the most suitable materials for a variety of THz applications.
Summary
We have measured the THz properties of a set of nematic LCs – E7, BL037, MDA-98-1602, LCMS-107, 1825 and GT3-23001 – using THz-TDS. Broadband extraordinary and ordinary refractive indices and absorption coefficient were extracted. In addition, the response as a function of alignment voltages was analysed for thick LC cells. Among all the LC materials studied, 1825 has the largest THz birefringence () with small loss parameter and small dichroism. It is a good candidate for efficient and compact THz modulation, such as phase shifters and switches. To further explore an LC material with lower absorption and lower dichroism while maintaining a reasonable high THz birefringence, detailed studies of the contributions by different constituents will be needed.
Disclosure statement
No potential conflict of interest was reported by the authors.
Additional information
Funding
References
- Ferguson B, Zhang XC. Materials for terahertz science and technology. Nat Mater. 2002;1:26–33. doi:10.1038/nmat708.
- Tonouchi M. Cutting-edge terahertz technology. Nat Photon. 2007;1:97–105. doi:10.1038/nphoton.2007.3.
- Williams BS. Terahertz quantum-cascade lasers. Nat Photon. 2007;1:517–525. doi:10.1038/nphoton.2007.166.
- Naftaly M, Miles RE. Terahertz time-domain spectroscopy for material characterization. Proc IEEE. 2007;95:1658–1665. doi:10.1109/JPROC.2007.898835.
- Walther M, Plochocka P, Fischer B, et al. Collective vibrational modes in biological molecules investigated by terahertz time-domain spectroscopy. Biopolymers. 2002;67:310–313. doi:10.1002/bip.10106.
- Matsushima F, Odashima H, Iwasaki T, et al. Frequency measurement of pure rotational transitions of H2 O from 0.5 to 5 THz. J Mol Struct. 352/353 (1995) 371–378.
- Ulbricht R, Hendry E, Shan J, et al. Carrier dynamics in semiconductors studied with time-resolved terahertz spectroscopy. Rev Mod Phys. 2011;83:543–586. doi:10.1103/RevModPhys.83.543.
- Siegel PH. Terahertz technology in biology and medicine. IEEE Trans Microw Theory Tech. 2004;52:2438–2447. doi:10.1109/TMTT.2004.835916.
- Son JH. Terahertz bio-imaging for medical applications. 2013 Conference on lasers and electro-optics Pacific Rim. 2013 Jun 30-Jul 04; Kyoto, Japan. paper TuC2_1.
- Federici J, Moeller L. Review of terahertz and subterahertz wireless communications. J Appl Phys. 2010;107:111101–111122. doi:10.1063/1.3386413.
- Song HJ, Ajito K, Wakatsuki A, et al. Terahertz wireless communication link at 300 GHz. Proceedings of the IEEE International Topical Meeting on Microwave Photonics (MWP'10), 2010 Oct 5–9; Montreal, Canada, p. 42–45. doi:10.1109/MWP.2010.5664230.
- Kersting R, Strasser G, Unterrainer K. Terahertz phase modulator. Electron Lett. 2000;36:1156. doi:10.1049/el:20000837.
- Chan WL, Chen HT, Taylor AJ, et al. A spatial light modulator for terahertz beams. Appl Phys Lett. 2009;94:213511–213513. doi:10.1063/1.3147221.
- Kawamoto H. The history of liquid-crystal displays. Proc IEEE. 2002;90:460–500. doi:10.1109/JPROC.2002.1002521.
- Park H, Parrott EPJ, Fan F, et al. Evaluating liquid crystal properties for use in terahertz devices. Opt Express. 2012;20:11899–11905. doi:10.1364/OE.20.011899.
- Wang L, Lin X, Liang X, et al. Large birefringence liquid crystal material in terahertz range. Opt Mater Express. 2012;2:1314. doi:10.1364/OME.2.001314.
- Vieweg N, Shakfa MK, Koch M. BL037: a nematic mixture with high terahertz birefringence. Opt Commun. 2011;284:1887–1889. doi:10.1016/j.optcom.2010.12.061.
- Yang C-S, Lin C-J, Pan R-P, et al. The complex refractive indices of the liquid crystal mixture E7 in the terahertz frequency range. J Opt Soc Am B. 2010;27:1866. doi:10.1364/JOSAB.27.001866.
- Chodorow U, Chojnowska O, Parka J. Properties of two-component nematic liquid crystal mixtures in the range of 0.3-3.0 THz. Liq Cryst. 2015;42:1243–1249. doi:10.1080/02678292.2015.1036816.
- Vieweg N, Shakfa MK, Scherger B, et al. THz properties of nematic liquid crystals. J Infrared Millim Terahertz Waves. 2010;31:1312–1320. doi:10.1007/s10762-010-9721-1.
- Vieweg N, Deninger A. Terahertz waves and liquid crystals: prospects and challenges. Proceedings of SPIE 8642, Emerging Liquid Crystal Technologies VIII, 86420K; 2013 Feb 5–6; San Francisco, CA. doi:10.1117/12.2013315.
- Wilk R, Vieweg N, Kopschinski O, et al. THz spectroscopy of liquid crystals from the cb family. J Infrared Millim Terahertz Waves. 2009;30:1139–1147. doi:10.1007/s10762-009-9537-z.
- Yu C, Chodorow U, Parrott EPJ, et al. Large birefringence liquid crystal in terahertz range with temperature tuning. 38th International Conference on Infrared, Millimeter, and Terahertz Waves (IRMMW-THz); 2013 Sept 1–6; Mainz, Germany. doi:10.1109/IRMMW-THz.2013.6665410
- Herman J, Kula P, Aptacy A, et al. New high birefringent liquid crystalline medium for terahertz applications. SID Mid-Europe Chapter Spring Meeting; 2013; P4, p. 69–71, 2013 Apr 15–16; Ghent, Belgium.
- Kowerdziej R, Stanczyk T, Parka J. Electromagnetic simulations of tunable terahertz metamaterial infiltrated with highly birefringent nematic liquid crystal. Liq Cryst. 2015;42:430–434. doi:10.1080/02678292.2014.1000406.
- Parrott EPJ, Zeitler JA, Friscic T, et al. Testing the sensitivity of terahertz spectroscopy to changes in molecular and supramolecular structure: a study of structurally similar cocrystal. Cryst Growth Des. 2009;9:1452–1460. doi:10.1021/cg8008893.
- Vieweg N, Fischer BM, Reuter M, et al. Ultrabroadband terahertz spectroscopy of a liquid crystal. Opt Express. 2012;20:28249–28256. doi:10.1364/OE.20.028249.
- Reuter M, Garbat K, Vieweg N, et al. Terahertz and optical properties of nematic mixtures composed of liquid crystal isothiocyanates, fluorides and cyanides. J Mater Chem C. 2013;1:4457. doi:10.1039/c3tc30464g.
- Trushkevych O, Xu H, Lu T, et al. Broad spectrum measurement of the birefringence of an isothiocyanate based liquid crystal. Appl Opt. 2010;49:5212–5216. doi:10.1364/AO.49.005212.
- Reuter M, Vieweg N, Fischer BM, et al. Highly birefringent, low-loss liquid crystals for terahertz applications. APL Mater. 2013;1:012107. doi:10.1063/1.4808244.
- Dąbrowski R, Kula P, Herman J. High birefringence liquid crystals. Crystals. 2013;3:443–482. doi:10.3390/cryst3030443.
- Evans GJ, Moscicki JK, Evans MW. The Poley absorption in liquid crystals. J Mol Liq. 1986;32:149–160. doi:10.1016/0167-7322(86)80020-4.